
Wypych Handbook of Solvents
.pdf1
Introduction
Christian Reichardt
Department of Chemistry, Philipps University, Marburg, Germany
Chemical transformations can be performed in a gas, liquid, or solid phase, but, with good reasons, the majority of such reactions is carried out in the liquid phase in solution. At the macroscopic level, a liquid is the ideal medium to transport heat to and from exoand endothermic reactions. From the molecular-microscopic point of view, solvents break the crystal lattice of solid reactants, dissolve gaseous or liquid reactants, and they may exert a considerable influence over reaction rates and the positions of chemical equilibria. Because of nonspecific and specific intermolecular forces acting between the ions or molecules of dissolved reactants, activated complexes as well as products and solvent molecules (leading to differential solvation of all solutes), the rates, equilibria, and the selectivity of chemical reactions can be strongly influenced by the solvent. Other than the fact that the liquid medium should dissolve the reactants and should be easily separated from the reaction products afterwards, the solvent can have a decisive influence on the outcome (i.e., yield and product distribution) of the chemical reaction under study. Therefore, whenever a chemist wishes to perform a certain chemical reaction, she/he has to take into account not only suitable reaction partners and their concentrations, the proper reaction vessel, the appropriate reaction temperature, and, if necessary, the selection of the right reaction catalyst but also, if the planned reaction is to be successful, the selection of an appropriate solvent or solvent mixture.
Solvent effects on chemical reactivity have been studied for more than a century, beginning with the pioneering work of Berthelot and Saint Gilles1 in Paris in 1862 on esterification reactions and with that of Menschutkin2 in St. Petersburg in 1880 on the quaternization of tertiary amines by haloalkanes. At this time Menschutkin remarked that “a reaction cannot be separated from the medium in which it is performed... Experience shows that solvents exert considerable influence on reaction rates.” Today, we can suggest a striking example to reinforce his remark, the rate of the unimolecular heterolysis of 2-chloro-2-methylpropane observed in water and benzene increases by a factor of approximately1011 when the nonpolar benzene is replaced by water.3,4 The influence of solvents on the position of chemical equilibria was discovered in 1896 by Claisen5 in Aachen, Knorr6 in Jena, Wislicenus7 in Wòrzburg, and Hantzsch8 in Wòrzburg. They investigated almost simultaneous but independent of one another the keto-enol tautomerism of 1,3-dicarbonyl compounds and the nitro-isonitro tautomerism of primary and secondary
2 |
Christian Reichardt |
aliphatic nitro compounds. With this example, the enol content of acetylacetone increases from 62 to 95 % when acetonitrile is substituted with n-hexane.3,9
The proper solvent and solvent mixture selection is not only important for chemical but also for physical processes such as recrystallization, all extraction processes, partitioning, chromatographic separations, phase-transfer catalytic reactions, etc. Of particular interest in this context is the influence of solvents on all types of light absorption processes, e.g., on UV/Vis, IR, ESR, and NMR spectra, caused by differential solvation of the ground and excited states of the absorbing species.3,12 In 1878, Kundt10 in Zòrich proposed the rule that increasing dispersion interactions between the absorbing solute and the solvent lead in general to a bathochromic shift of an UV/Vis absorption band. Later, in 1922, Hantzsch11 termed the solvent-dependence of UV/Vis absorption spectra “solvatochromism”. UV/Vis absorption of solute molecules can be influenced not only by the surrounding solvent sphere, but also by other entities in the surroundings such as solids, polymers, glasses, and surfaces. In order to emphasize this influence, the use of the more general term “perichromism” (from Greek peri = around) has been recommended.12,13 A typical, more recent, example of extraordinary solvatochromism is the intramolecular charge-transfer Vis-absorption of 2,6-diphenyl-4-(2,4,6-triphenyl-l-pyridinio)phenolate, a zwitterionic betaine dye: its corresponding absorption band is shifted from λmax = 810 nm to λmax = 453 nm (Δλ = 357 nm) when diphenyl ether is replaced by water as solvent.3,12 Such solvatochromic dyes can be used as empirical solvent polarity indicators.12
The number of solvents generally available to chemists working in research and industrial laboratories is between 250 and 3003,14 (there is an infinite number of solvent mixtures), and this number is increasing. More recently and for obvious reasons, the search for new solvents has been intensified: peroxide-forming solvents are being substituted by solvents which are more stable against oxidation (e.g., diethyl ether by t-butyl methyl ether or by formaldehyde dialkyl acetals), toxic solvents are being replaced by nontoxic ones (e.g., the cancerogenic hexamethylphosphoric triamide, HMPT, by N,N'-dimethylpropyleneurea, DMPU15) and environmentally dangerous solvents by benign ones (e.g., tetrachloromethane by perfluorohexane16). The development of modern solvents for organic syntheses is the subject of much current research.17 Amongst these modern solvents, also called “neoteric solvents” (neoteric = recent, new, modern) in contrast to the classical ones, are ionic liquids (i.e., room-temperature liquid salts such as 1-ethyl-3-methylimidazolium tetra-chloroaluminates18,19), supercritical-fluid solvents, SCF, (such as SCF carbon dioxide20,21), and perfluorinated solvents (e.g., partially or perfluorinated hydrocarbons as used in so-called “fluorous biphase catalysis reactions”, making possible mono-phase reactions and a two-phase separation of catalyst and reaction products22-24). Even plain water has found a magnificent renaissance as a solvent for organic reactions.25,26 These efforts have also recently strengthened the search for completely solvent-free reactions, thus avoiding the use of expensive, toxic, and environmentally problematic solvents.27,28
With respect to the large and still increasing number of valuable solvents useful for organic syntheses, a chemist needs, in addition to his experience and intuition, to have general rules, objective criteria, and the latest information about the solvents' physical, chemical, and toxicological properties for the selection of the proper solvent or solvent mixture for a planned reaction or a technological process. To make this often cumbersome and time-consuming task easier, this “Handbook of Solvents” with its twenty-five chapters is designed to provide a comprehensive source of information on solvents over a broad range
1 Introduction |
3 |
of applications. It is directed not only to chemists working in research laboratories, but also to all industries using solvents for various purposes. A particular advantage is that the printed handbook is accompanied by a compact-disc (CD-ROM) containing additional solvent databases with hundred ten fields for over eleven hundred solvents. This makes large data sets easily available for quick search and retrieval and frees the book text from bulky tables, thus giving more room for a thorough description of the underlying theoretical and practical fundamental subjects.
Fundamental principles governing the use of solvents (i.e., chemical structure, molecular design as well as physical and chemical properties of solvents) are given in Chapter 2. Solvent classification, methods of solvent manufacture together with properties and typical applications of various solvents are provided in Chapter 3. Chapters 4, 5 and 6 deal with all aspects of the dissolution of materials in solvents as well as with the solubility of selected systems (e.g., polymers and elastomers) and the influence of the solute's molecular structure on its solubility behavior. In particular, the valuable solubility-parameter concept is extensively treated in these chapters. All aspects of solvent transport within polymeric system and the drying of such polymeric systems, including coated films, are described in Chapter 7. The fundamentals of the interaction forces acting between ions or molecules of the solvents themselves and between solutes and solvents in solutions are presented in Chapter 8. Chapter 9 deals with the corresponding properties of solvent mixtures. Specific solute/solvent interactions, particularly Lewis acid/base interactions between electron-pair donors (EPD) and electron-pair acceptors (EPA), are reviewed in Chapter 10, together with the development of empirical scales of solvent polarity and Lewis acidity/basicity, based on suitable solvent-dependent reference processes, and their application for the treatment of solvent effects. The theory for solvent effects on electronic properties is provided in Chapter 11 and extended to solvent-dependent properties of solutes such as fluorescence spectra, ORD and CD spectra. Aggregation, swelling of polymers, their conformations, the viscosity of solutions and other solvent-related properties are treated in Chapter 12. A review concerning solvent effects on various types of chemical reactivity is given in Chapter 13, along with a discussion of the effects of solvent on free-radical polymerization and phase-transfer catalysis reactions.
The second part of this handbook (Chapters 14-25) is devoted more to the industrial use of solvents. Formulating with solvents applied in a broad range of industrial areas such as biotechnology, dry cleaning, electronic industry, food industry, paints and coatings, petroleum refining industry, pharmaceutical industry, textile industry, to mention only a few, is extensively described in Chapter 14. Standard and special methods of solvent detection and solvent analysis as well as the problem of residual solvents in various products, particularly in pharmaceutical ones, are the topics of Chapters 15 and 16.
At present, large-scale chemical manufacturing is facing serious solvent problems with respect to environmental concerns. National and international regulations for the proper use of hazardous solvents are becoming increasingly stringent and this requires the use of environmentally more benign but nevertheless economical liquid reaction media. This has enormously stimulated the search for such new solvent systems within the framework of so-called green chemistry. Supercritical fluids, SCF,20,21 and ionic liquids (room temperature liquid salts)18,19 have been known and have been the subject of scientific interest for a long time. It is only recently, however, that the potential benefits of these materials in solvent applications have been realized.17 This handbook includes in Chapters 17-25 all
4 |
Christian Reichardt |
the knowledge necessary for a safe handling of solvents in research laboratories and in large-scale manufacturing, beginning with the environmental impact of solvents on water, soil, and air in Chapter 17, followed by considerations about safe solvent concentrations and the risks of solvent exposure in various industrial environments in Chapter 18. Chapter 19 summarizes the corresponding legal regulations, valid for North America and Europe, and Chapter 20 describes in detail the toxic effects of solvent exposure to human beings. Authors specializing in different fields of solvent toxicity give the most current information on the effect of solvent exposure from the point of view of neurotoxicity, reproductive and maternal effects, nephrotoxicity, cancerogenicity, hepatotoxicity, chromosomal aberrations, and toxicity to brain, lungs, and heart. This information brings both the results of documented studies and an evaluation of risk in different industrial environments in a comprehensive but easy to understand form to engineers and decision-makers in industry. Chapter 21 is focused on the substitution of harmful solvents by safer ones and on the development of corresponding new technological processes. Chapter 22 describes modern methods of solvent recovery, solvent recycling. When recycling is not possible, then solvents have to be destroyed by incineration or other methods of oxidation, as outlined in Chapter 22. Chapter 23 describes natural attenuation of solvents in groundwater and advanced remediation technologies as well as management strategies for sites impacted by solvent contamination. Protection from contact with solvents and their vapors is discussed in Chapter 24. Finally, new trends in solvent chemistry and applications based on the recent patent literature are discussed in Chapter 25.
In most cases, the intelligent choice of the proper solvent or solvent mixture is essential for the realization of certain chemical transformations and physical processes. This handbook tries to cover all theoretical and practical information necessary for this often difficult task for both academic and industrial applications. It should be used not only by chemists, but also by physicists, chemical engineers, and technologists as well as environmental scientists in academic and industrial institutions. It is to be hoped that the present compilation of all relevant aspects connected with the use of solvents will also stimulate further basic and applied research in the still topical field of the physics and chemistry of liquid media.
REFERENCES
1M Berthelot, L P¾an de Saint Gilles, Ann. Chim. Phys., 3. S¾r., 65, 385 (1862); ibid. 66, 5 (1862); ibid. 68, 255 (1863).
2N Menschutkin, Z. Phys. Chem., 5, 589 (1890); ibid. 6, 41 (1890); ibid. 34, 157 (1900).
3C Reichardt, Solvents and Solvent Effects in Organic Chemistry, 2nd ed., VCH, Weinheim, 1988.
4(a) G F Dvorko, E A Ponomareva, Usp. Khim., 53, 948 (1984); Russ. Chem. Rev., 53, 547 (1984);
(b) M H Abraham, Pure Appl. Chem., 57, 1055 (1985); and references cited therein.
5 L Claisen, Justus Liebigs Ann. Chem., 291, 25 (1896).
6L Knorr, Justus Liebigs Ann. Chem., 293, 70 (1896).
7W Wislicenus, Justus Liebigs Ann. Chem., 291, 147 (1896).
8 A Hantzsch, O W Schultze, Ber. Dtsch. Chem. Ges., 29, 2251 (1896).
9M T Rogers, J L Burdett, Can. J Chem., 43, 1516 (1965).
10A Kundt, Poggendorfs Ann. Phys. Chem. N. F., 4, 34 (1878); Chem. Zentralbl., 498 (1878).
11A Hantzsch, Ber. Dtsch. Chem. Ges., 55, 953 (1922).
12C Reichardt, Chem. Rev., 94, 2319 (1994).
13Prof. E M Kosower, Tel Aviv, private communication to C.R.
14Y Marcus, The Properties of Solvents, Wiley, Chichester, 1998.
15(a) Editorial, Chimia, 39, 147 (1985);
(b)D Seebach, Chemistry in Britain, 21, 632 (1985).
16S M Pereira, G P Sauvage, G. W. Simpson, Synth. Commun., 25, 1023 (1995).
Introduction |
5 |
17P Knochel (Ed.), Modern Solvents in Organic Synthesis, Topics in Current Chemistry, Vol. 206, Springer, Berlin, 1999.
18Y Chauvin, H Olivier-Bourbigou, CHEMTECH, 25(9), 26 (1995).
19(a) K R Seddon, Kinetika i Kataliz, 37, 743 (1996); Kinetics and Catalysis, 37, 693 (1996); Chem. Abstr., 125, 285927s (1996);
(b) K R Seddon, J. Chem. Technol. Biotechnol., 68, 351 (1997); Chem. Abstr., 126, 306898w (1997).
20R Noyori (Ed.), Supercritical Fluids, Chem. Rev., 99, 353-633 (1999).
21W Leitner, Top. Curr. Chem., 206, 107 (1999).
22B Cornils, Angew. Chem., 109, 2147 (1997); Angew. Chem., Int. Ed. Engl., 36, 2057 (1997).
23B Betzemeier, P Knochel, Top. Curr. Chem., 206, 61 (1999).
24J J Maul, P J Ostrowski, G A Ublacker, B Linclau, D P Curran, Top. Curr. Chem., 206, 79 (1999).
25P A Grieco, Organic Synthesis in Water, Blackie Academic and Professional, Hampshire, 1998.
26A Lubineau and J. Aug¾, Top. Curr. Chem., 206, 1 (1999).
27 |
J O Metzger, Angew, Chem., 110, 3145 (1998); Angew. Chem., Int. Ed. Engl., 37, 2975 (1998). |
28 |
A Loupy, Top. Curr. Chem., 206, 153 (1999). |

10
Acid-base Interactions
10.1 GENERAL CONCEPT OF ACID-BASE INTERACTIONS
George Wypych
ChemTec Laboratories, Inc., Toronto, Canada
Acid-base interactions have found numerous applications in research dealing with adsorption of molecules of liquids on the surfaces of solids. The main focus of this research is to estimate the thermodynamic work of adhesion, determine mechanism of interactions, analyze the morphology of interfaces and various surface coatings, develop surface modifiers, study the aggregation of macromolecular materials, explain the kinetics of swelling and drying, understand the absorption of low molecular weight compounds in polymeric matrices, and determine the properties of solid surfaces. In addition to these, there are many other applications.
Several techniques are used to determine and interpret acid-base interactions. These include: contact angle, inverse gas chromatography, IGC, Fourier transform infrared, FTIR, and X-ray photoelectron spectroscopy, XPS. These methods, as they are applied to solvents are discussed below.
Contact angle measurements have long been used because of common availability of instruments. In recent years, they have been developed from simple optical devices to the present day precise, sophisticated, computer-controlled instruments with sufficient precision. Van Oss and Good1,2 developed the basic theory for this method. Their expression for surface free energy is used in the following form:
|
|
|
γ = γLW + γAB = γLW + 2 γ+γ− |
[10.1.1] |
where:
γLW |
Lifshitz-van der Waals interaction |
γAB |
acid-base interaction |
γ+ |
Lewis acid parameter of surface free energy |
γ- |
Lewis base parameter of surface free energy. |
The following relationship is pertinent from the equation [10.1.1]:
|
|
|
γAB = 2 γ+γ− |
[10.1.2] |
A three-liquid procedure was developed1-3 which permits the determination of the acid-base interaction from three measurements of contact angle:

566 |
|
|
|
|
|
|
|
|
|
|
|
|
|
|
George Wypych |
γL1 (1+ cos θ1 ) = 2( |
|
|
|
|
|
|
|
|
|
|
|
|
) |
|
|
γSLW γLLW1 |
|
+ |
γS+ γL−1 |
|
|
+ |
γS− γ+L1 |
|
|||||||
γL 2 (1+ cos θ2 ) = 2( |
|
|
|
|
|
|
|
|
|
) |
|
||||
γSLW γLLW2 |
+ |
|
γS+ γL− |
2 |
+ |
γS− γ+L 2 |
[10.1.3] |
||||||||
γL 3 (1+ cos θ3 ) = 2( |
|
|
|
|
|
|
|
|
|
) |
|
||||
γSLW γLLW3 |
+ |
|
γS+ γL− |
3 |
+ |
|
γS− γ+L 3 |
|
Solving this set of equations permits the calculation of solid parameters from equation [10.1.1]. The work of adhesion, Wa, between a solid and a liquid can be calculated using the Helmholtz free energy change per unit area:
− GSL = WaSL = γS + γL − γSL = 2(γSLW γLWL +
γ+S γ−L +
γS− γ+L ) [10.1.4]
Determination is simple. However, several measurements (usually 10) should be taken to obtain a reliable averages (error is due mostly to surface inhomogeneity). There is a choice between measuring the advancing or the receding contact angle. Advancing contact angles are more representative of equilibrium contact angles.3
Inverse gas chromatography data are interpreted based on Papirer’s equation:4,5
RT lnVN = 2N(γSD )1/ 2 a(γLD )1/ 2 + c |
[10.1.5] |
where:
Rgas constant
Ttemperature
VN |
net retention volume |
NAvogadro’s number
γD |
dispersion component of surface energy |
S, L |
indices for solid and liquid, respectively |
amolecular area of adsorbed molecule
cintegration constant relative to a given column.
Several probes are used to obtain the relationship between RTlnVN and aγDL . From this relationship the reference retention volume, VNREF , is calculated and used to calculate the acid-base interaction’s contribution to the free energy of desorption:
GAB |
= RT ln |
VN |
[10.1.6] |
|
|
|
|||
|
V REF |
|
||
|
|
N |
|
|
If data from a suitable temperature range for |
GAB can be obtained, the acid-base enthalpy, |
|||
HAB can be calculated using the following equation: |
||||
HAB = KaDN + Kb AN |
[10.1.7] |
|||
where: |
|
|
|
|
Ka |
acid interaction constant |
|
||
Kb |
base interaction constant |
|
||
DN |
donor number |
|
||
AN |
acceptor number |
|
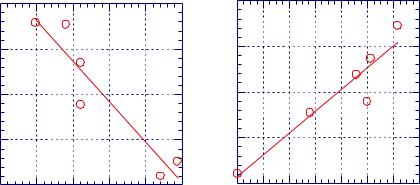
10.1 General concept of acid-base interactions |
567 |
|
199.5 |
|
|
|
|
|
323.5 |
|
|
|
|
|
|
|
|
|
|
|
|
|
|
|
|
|
|
|
|
|
|
|
|
|
199 |
|
|
|
|
eV |
323 |
|
|
|
|
|
|
|
|
|
|
|
|
|
|
|
|
|
|
|
|
|
|
||
|
|
|
|
|
|
|
|
|
|
|
|
|
|
|
|
Cl 2p , eV 3/2 |
198.5 |
|
|
|
|
Binding energy, |
322.5 |
|
|
|
|
|
|
|
|
198 |
|
|
|
|
322 |
|
|
|
|
|
|
|
|
||
|
197.5 |
1.5 |
2 |
2.5 |
3 |
3.5 |
321.5 |
20 |
40 |
|
60 |
80 |
100 |
120 |
140 |
|
1 |
0 |
|
||||||||||||
|
|
- |
H, kcal mol-1 |
|
|
|
|
- |
H |
, kJ mol-1 |
|
|
|||
|
|
|
|
|
|
|
|
|
|
|
AB |
|
|
|
|
Figure 10.1.1. Correlation of binding energy and HAB for several polymers. [Adapted, by permission, from J F Watts, M M Chehimi, International J. Adhesion Adhesives, 15, No.2, 91-4 (1995).]
Figure 10.1.2. Correlation of binding energy and HAB for several solvents. [Adapted, by permission, from
J F Watts, M M Chehimi, International J. Adhesion Adhesives, 15, No.2, 91-4 (1995).]
Finally the plot of HAB/AN vs. DN/AN gives Ka and Kb. Further details of this method are described elsewhere.4-9 It can be seen that the procedure is complicated. The various conditions of experiments conducted in various laboratories were sufficiently different to prevent correlation of data between laboratories.10 To rectify this situation, a large body of data was obtained for 45 solvents and 19 polymers tested under uniform conditions.10
Fowkes11 showed that the carbonyl stretching frequency shifts to lower values as the dispersion component of surface tension increases. The following empirical relationship
was proposed: |
|
|
HAB = 0.236 v AB |
[10.1.8] |
|
where: |
|
|
HAB |
the enthalpy change on acid-base adduct formation |
|
vAB |
projection of carbonyl stretching frequency on dispersive line. |
|
It should be noted that, as Figure 7.1.16 shows, the change in frequency of carbonyl stretching mode is related to the process of crystallization.12
XPS is emerging as very precise method for evaluating acid-base interactions based on the works by Chehimi et al.6-9 There is very good correlation between XPS chemical shift and the change in exothermic enthalpy of acid-base interaction. Drago’s equation is used for data interpretation:13
− HAB = E A E B + CA CB |
[10.1.9] |
where:
A, B |
subscripts for acid and base, respectively |
EA, EB |
susceptibility of acid or base species to undergo an electrostatic interaction |
CA, CB |
susceptibility of acid or base species to undergo a covalent interaction. |

568 |
|
|
|
|
|
|
|
|
|
|
|
1 |
|
|
|
|
|
|
|
|
|
|
|
|
|
|
|
|
CCl |
|
|
|
|
0.9 |
|
|
|
|
|
|
4 |
|
|
|
|
|
|
|
|
|
|
|
|
|
Cl2p/ClLMM |
0.8 |
|
|
|
|
|
|
|
|
|
|
|
|
|
TLC |
|
|
|
|
|
|
0.7 |
|
|
|
|
|
|
|
|
|
|
|
|
|
|
|
|
|
|
DCM |
|
|
0.6 |
|
|
|
|
|
|
|
|
|
|
|
|
|
|
|
DCE |
|
|
|
||
|
|
|
|
|
|
|
|
|
||
|
0.5 |
THF |
|
|
|
|
|
CHCl |
|
|
|
|
|
|
DXN |
|
|
|
|
3 |
|
|
|
|
|
|
|
|
|
|
|
|
|
0.4 |
|
15 |
10 |
5 |
0 |
5 |
10 |
15 |
20 |
|
20 |
|||||||||
|
|
|
|
DN |
|
|
|
AN |
|
|
Figure 10.1.3. Intensity ratio vs DN and AN. [Adapted, |
||||||||||
by permission, from M L Abel, M M Chehimi, |
||||||||||
Synthetic Metals, 66, No.3, 225-33 (1994).] |
|
|
George Wypych
|
50 |
|
|
|
|
|
|
% |
40 |
|
|
|
|
|
|
content, |
|
|
|
|
|
|
|
30 |
|
|
|
|
|
|
|
|
|
|
|
|
|
|
|
surface |
20 |
|
|
|
|
|
|
|
|
|
|
|
|
|
|
PMMA |
10 |
|
|
|
|
|
|
|
|
|
|
|
|
|
|
|
0 |
0.5 |
0.6 |
0.7 |
0.8 |
0.9 |
1 |
|
0.4 |
||||||
|
|
|
Cl2p/ClLMM |
|
|
||
Figure 10.1.4. PMMA surface content vs. intensity ratio. |
|||||||
[Data from M L Abel, M M Chehimi, Synthetic Metals, |
|||||||
66, No.3, 225-33 (1994).] |
|
|
|
|
|
10 |
|
|
|
|
|
|
|
|
|
|
|
|
|
|
|
|
|
|
|
|
|
|
|
|
|
|
|
|
|
|
|
|
|
|
angstrom |
8 |
|
|
|
|
|
|
|
|
|
|
|
|
|
|
|
|
|
|
|
|
|
|
|
|
|
|
|
|
|
|
|
|
|
|
|
|
|
|
|
|
|
|
|
|
|
|
|
|
|
|
|
|
|
|
|
|
|
|
|
|
|
|
|
|
|
|
|
|
||
|
|
|
|
|
|
|
|
|
|
|
|
|
|
|
|
|
|
|
|
|
|
|
|
|
|
|
|
|
|
|
|
|
|
||
|
|
|
|
|
|
|
|
|
|
|
|
|
|
|
|
|
|
|
|
|
|
|
|
|
|
|
|
|
|
|
|
|
|
||
|
|
|
|
|
|
|
|
|
|
|
|
|
|
|
|
|
|
|
|
|
|
|
|
|
|
|
|
|
|
|
|
|
|
||
6 |
|
|
|
|
|
|
|
|
|
|
|
|
|
|
|
|
|
|
|
|
|
|
|
|
|
|
|
|
|
|
|
|
|
|
|
|
|
|
|
|
|
|
|
|
|
|
|
|
|
|
|
|
|
|
|
|
|
|
|
|
|
|
|
|
|
|
|
|
|
||
|
|
|
|
|
|
|
|
|
|
|
|
|
|
|
|
|
|
|
|
|
|
|
|
|
|
|
|
|
|
|
|
|
|
||
|
|
|
|
|
|
|
|
|
|
|
|
|
|
|
|
|
|
|
|
|
|
|
|
|
|
|
|
|
|
|
|
|
|
||
|
|
|
|
|
|
|
|
|
|
|
|
|
|
|
|
|
|
|
|
|
|
|
|
|
|
|
|
|
|
|
|
|
|
||
|
|
|
|
|
|
|
|
|
|
|
|
|
|
|
|
|
|
|
|
|
|
|
|
|
|
|
|
|
|
|
|
|
|
|
|
|
|
|
|
|
|
|
|
|
|
|
|
|
|
|
|
|
|
|
|
|
|
|
|
|
|
|
|
|
|
|
|
|
|
|
|
Thickness, |
4 |
|
|
|
|
|
|
|
|
|
|
|
|
|
|
|
|
|
|
|
|
|
|
|
|
|
|
|
|
|
|
|
|
|
Figure 10.1.6. Schematic diagram of surface roughness |
|
|
|
|
|
|
|
|
|
|
|
|
|
|
|
|
|
|
|
|
|
|
|
|
|
|
|
|
|
|
|
|
|
|||
|
|
|
|
|
|
|
|
|
|
|
|
|
|
|
|
|
|
|
|
|
|
|
|
|
|
|
|
|
|
|
|
|
|||
|
|
|
|
|
|
|
|
|
|
|
|
|
|
|
|
|
|
|
|
|
|
|
|
|
|
|
|
|
|
|
|
|
|||
|
|
|
|
|
|
|
|
|
|
|
|
|
|
|
|
|
|
|
|
|
|
|
|
|
|
|
|
|
|
|
|
|
|
||
|
|
|
|
|
|
|
|
|
|
|
|
|
|
|
|
|
|
|
|
|
|
|
|
|
|
|
|
|
|
|
|
|
|
of polypyrrole and deposition of PMMA from two |
|
|
|
|
|
|
|
|
|
|
|
|
|
|
|
|
|
|
|
|
|
|
|
|
|
|
|
|
|
|
|
|
|
|
|
||
2 |
|
|
|
|
|
|
|
|
|
|
|
|
|
|
|
|
|
|
|
|
|
|
|
|
|
|
|
|
|
|
|
|
|
different solvents. [Adapted, by permission, from |
|
|
|
|
|
|
|
|
|
|
|
|
|
|
|
|
|
|
|
|
|
|
|
|
|
|
|
|
|
|
|
|
|
|
|||
|
|
|
|
|
|
|
|
|
|
|
|
|
|
|
|
|
|
|
|
|
|
|
|
|
|
|
|
|
|
|
|
|
|
M L Abel, J L Camalet, M M Chehimi, J F Watts, |
|
|
|
|
|
|
|
|
|
|
|
|
|
|
|
|
|
|
|
|
|
|
|
|
|
|
|
|
|
|
|
|
|
|
|
|
|
|
0 |
|
|
|
|
|
|
|
|
|
|
|
|
|
|
|
|
|
|
|
|
|
|
|
|
|
|
|
|
|
|
|
|
|
P A Zhdan, Synthetic Metals, 81, No.1, 23-31 (1996).] |
|
|
|
|
|
|
|
|
|
|
|
|
|
|
|
|
|
|
|
|
|
|
|
|
|
|
|
|
|
|
|
|
|
|
||
|
|
|
|
|
|
|
|
|
|
|
|
|
|
|
|
|
|
|
|
|
|
|
|
|
|
|
|
|
|
|
|
|
|
|
|
|
|
|
|
|
|
|
|
|
|
|
|
|
|
|
|
|
|
|
|
|
|
|
|
|
|
|
|
|
|
|
|
|
|
|
|
|
|
|
|
|
|
|
|
|
|
|
|
|
|
|
|
|
|
|
|
|
|
|
|
|
|
|
|
|
|
|
|
|
|
|
|
|
0.4 |
0.5 |
0.6 |
0.7 |
0.8 |
|
0.9 |
|
|
1 |
The determination of properties of un- |
||||||||||||||||||||||||
|
|
|
|
|
|
|
|
|
|
|
|
|
|
|
|
|
|
|
|
|
|
|
|
|
|
|
|
|
|
|
|
|
|
|
|
|
|
|
|
|
|
|
|
|
|
|
|
Cl2p/ClLMM |
|
|
|
|
|
|
|
|
|
known system requires that master curve be |
Figure 10.1.5. Thickness of PMMA overlayers vs. |
constructed. This master curve is deter- |
intensity ratio. [Data from M L Abel, M M Chehimi, |
mined by testing a series of different poly- |
Synthetic Metals, 66, No.3, 225-33 (1994).] |
mers exposed to a selected solvent. An |
|
|
|
example of such a relationship is given in |
Figure 10.1.1. The enthalpy change caused by the acid-base adduct formation is obtained from a study of the same solid with different solvent probes (see Figure 10.1.2). Having this data, coefficients E and C can be calculated from the chemical shifts in any system.
Figure 10.1.3 shows that there is a correspondence between DN and AN values of different solvents and Cl(2p)/Cl(LMM) intensity ratios.7 Figure 10.1.4 shows that the type of solvent (measured by its Cl(2p)/Cl(LMM) intensity ratio) determines adsorption of basic PMMA on acidic polypyrrole. Figure 10.1.5 shows that also the thickness of PMMA overlayer corresponds to Cl(2p)/Cl(LMM) intensity ratio of solvent.

10.1 General concept of acid-base interactions |
|
|
|
|
|
569 |
||||
|
8 |
|
|
|
|
-0.04 |
|
|
|
|
|
|
|
|
|
|
|
|
|
|
|
|
6 |
|
|
|
|
|
|
|
|
|
|
4 |
|
|
|
|
-0.08 |
|
|
|
|
V N |
|
|
|
|
|
|
|
|
|
|
ln |
2 |
|
|
|
|
χ |
|
|
|
|
RT |
|
|
|
|
|
|
|
|
|
|
|
0 |
|
|
|
|
-0.12 |
|
|
|
|
|
-2 |
|
|
|
|
|
|
|
|
|
|
-4 |
|
|
|
|
-0.16 |
|
|
|
|
|
5 |
6 |
7 |
8 |
9 |
1.76 |
1.8 |
1.84 |
1.88 |
1.92 |
|
|
Number of carbon atoms |
|
|
|
1000/T, K-1 |
|
|
||
|
|
|
|
|
|
|
|
|||
Figure 10.1.7. Relationship between RT ln VN and |
|
Figure 10.1.8. Flory interaction parameter vs. inverse |
||||||||
number of carbon atoms in n-alkanes. [Adapted, by |
|
temperature for polyamide. [Adapted, by permission, |
||||||||
permission from M M Chehimi, E Pigois-Landureau, |
|
from L Bonifaci, G Cavalca, D Frezzotti, E Malaguti, |
||||||||
M Delamar, J F Watts, S N Jenkins, E M Gibson, Bull. |
|
G P Ravanetti, Polymer, 33(20), 4343-6 (1992).] |
|
|||||||
Soc. Chim. Fr., 9(2) 137-44 (1992).] |
|
|
|
|
|
|
|
XPS analysis combined with analysis
by atomic force microscopy, AFM, deter-
mines differences in the surface distribution
of deposited PMMA on the surface of
polypyrrole depending on the type of sol-
vent used (Figure 10.1.6).6 AFM, in this ex-
periment, permitted the estimation of the
surface roughness of polypyrrole. When
PMMA was deposited from
tetrahydrofuran, a poor solvent, it assumed
a surface roughness equivalent to that of polypyrrole. Whereas PMMA, when deposited from a good solvent - CHCl3,6 developed a smooth surface. The above examples show the importance of solvents in coating morphology.
Figure 10.1.7 shows the correlation between the number of carbon atoms in n-alkanes and the net retention volume of solvent using IGC measurements.8 Such a
correlation must be established to calculate the acid-base interaction’s contribution to the free energy of desorption, GAB, as pointed out in discussion of equations [10.1.5] and [10.1.6]. Figure 10.1.8 shows that the Flory interaction parameter (measured by IGC) increases as the temperature increases.