
Gale Encyclopedia of Genetic Disorder / Gale Encyclopedia of Genetic Disorders, Two Volume Set - Volume 1 - A-L - I
.pdf
(MTHFR) molecule, there is a molecule known as flavin adenine dinucleotide, or FAD. The FAD molecule binds to the MTHFR molecule and helps with the conversion of homocysteine to methionine. Increased levels of folates help bind FAD more tightly to MTHFR, protect the enzyme against heat inactivation, and allow the homocysteine to methionine conversion pathway to proceed. Betaine and cobalamin also help in the conversion of homocysteine to methionine by acting as cofactors. The rationale behind this method of treatment is that although the methionine levels are raised, the net drop in homocysteine is beneficial as it appears that the elevated levels of homocysteine are what cause ectopia lentis, osteoporosis, mental deficiency, and thromboembolic events.
It appears that the addition of dietary betaine in B6-responsive patients is also beneficial. Homocysteine that is not metabolized to cysteine is converted back to methionine in a reaction that uses betaine, so the addition of betaine may help to make this reaction occur and thus reduce the levels of homocysteine.
Other treatments include protein restriction, specifically a low methionine diet with the addition of extra cysteine. Dietary treatment includes avoidance of all high protein foods throughout life, with the use of a nutritional supplement. Special formulas for infants are available. The reasoning behind this is to reduce the methionine and homocysteine levels that accumulate and supplement the low levels of cysteine.
The occurrence of clinically apparent thromboembolism depends upon the age of the affected individual and whether or not he/she responds to pyridoxine treatment. In one study, untreated pyridoxine-responsive patients were at little risk for a thromboembolic event until age 12. After age 12, the risk for thromboembolism increased. By age 20, patients who would have been responsive to pyridoxine had a 25% cumulative risk for a thromboembolic event. In comparison, individuals with CBS deficiency who were untreated and not responsive to pyridoxine treatment had a similar cumulative risk for a thromboembolic event by age 15.
In reference to the two common CBS gene alterations, CBS deficiency caused by the 1278T gene change is pyridoxine responsive. CBS deficiency caused by the G307S gene tends to be pyridoxine non-responsive; however this is not always the case as some individuals with the G307S gene change are pyridoxine responsive.
Very little is known about the risks to an unborn child of a mother with pyridoxine non-responsive CBS deficiency. There have been numerous reports of healthy children born to women and men who have pyridoxine responsive CBS deficiency, however only two reports of children born to pyridoxine non-responsive women have been reported and one had multiple birth defects that may
have been related to the mother’s condition. Potentially, the mother’s elevated levels of homocysteine can cause problems for a developing baby. This could be similar to the process by which infants of mothers who have phenylketonuria are affected by the elevated levels of phenylalanine if their mothers are not being treated with dietary restriction during pregnancy.
Prognosis
Untreated CBS deficiency leads to mental retardation, lens dislocation, and a decreased life expectancy because of complications associated with blood clots. If untreated from early infancy, approximately 20% of affected patients will have seizures. If treated from birth, prevention or long term delay of the complications of CBS deficiency can be expected.
Resources
BOOKS
Scriver, C.R., A.L. Beaudet, W.S. Sly, and D. Valle, eds. The
Metabolic Basis of Inherited Disease. 6th ed. New York: McGraw-Hill Medical Publishing Division, 1989.
ORGANIZATIONS
National Organization for Rare Disorders (NORD). PO Box 8923, New Fairfield, CT 06812-8923. (203) 746-6518 or (800) 999-6673. Fax: (203) 746-6481. http://www
.rarediseases.org .
WEBSITES
Climb: Children Living with Inherited Metabolic Diseases Support Group. http://www.climb.org.uk .
Reneé A. Laux, MS
Homogentisic acid oxidose deficiency see
Alkaptonuria
I Human Genome Project
The Human Genome Project (HGP) is the international project to sequence the DNA of the human genome. The sequencing work is conducted in many laboratories around the world, but the majority of the work is being done by five institutions: the Whitehead Institute for Medical Research in Massachusetts (WIMR), the Baylor College of Medicine in Texas, the University of Washington, the Joint Genome Institute in California, and the Sanger Centre near Cambridge in the United Kingdom. Most of the funding for these centers is provided by the United States National Institute of Health and Department
Project Genome Human
G A L E E N C Y C L O P E D I A O F G E N E T I C D I S O R D E R S |
565 |
Human Genome Project
of Energy, and the Wellcome Trust, a charitable foundation in the UK.
Completely sequencing the human genome was first suggested at a conference in Alta, Utah in 1984. The conference was convened by the U.S. Department of Energy, which was concerned with measuring the mutation rate of human DNA when exposed to low-level radiation, similar to conditions after an attack by nuclear weapons. The technology to make such measurements did not exist at the time, and the sequence of the genome was one step required for this aim to become possible. The genome was estimated to be 3000Mb long, however, and sequencing it seemed an arduous task, especially using the sequencing technology of the time. If most of the DNA was “junk” (not coding for genes), then scientists assumed that they could speed the process along by targeting specific genes for sequencing. This could be done by sequencing complementary DNAs (cDNA) which are derived from mRNAs used to code for proteins in the cell. Despite several advocates for this method, it was decided that the whole genome would be sequenced, with a target completion date of 2005. The Human Genome Project quickly became the world’s premier science project for biology, involving large factory-like laboratories rather than small laboratories of independent geneticists.
The strategy employed by the HGP involved three stages, and is termed hierarchical shotgun sequencing. The first stage involved generating physical and genetic maps of the human genome. The second stage was placing clones from a genomic library on to these maps. The third stage was fragmenting these genomic clones into smaller overlapping clones (shotgun cloning), which were a more suitable size for sequencing. Then, the complete sequence of each chromosome could be reconstructed by assembling the fragments of sequence that overlapped with each other to generate the sequence of the genomic clone. The sequence of each genomic clone could then be fitted together using the assembly (contig) of genomic clones on the genetic and physical map.
Although the ultimate aim was high-quality sequence of the human genome, it was recognized that the genetic and physical maps generated by the first stage of the HGP would be by themselves very useful for genetic research. The first generation physical map was constructed by screening a yeast artificial chromosome (YAC) genomic library to isolate YACs, and overlaps were identified by restriction enzyme digest “fingerprints” and STS content mapping. These STSs were sequenced around the highly polymorphic CA-repeat markers (microsatellites) that were used to generate the genetic map. Genetic maps were also constructed. These use recombination between markers in families to deduce
the distance separating and order of these markers. The first human genetic map used restriction fragment length polymorphisms (RFLPs) as markers, which only have two alleles per marker, but common microsatellites were used to create a high resolution genetic map.
The second stage of human genome sequencing was made simpler by the development of bacterial artificial chromosomes (BACs), cloning vectors that could carry up to 150kb of DNA. Before then, it was assumed that a contig of YACs and cosmids, carrying up to 2Mb and 40kb of DNA respectively, would be assembled. These two types of genomic clone were found to be liable to rearrangement; the DNA in the vector could be in chunks that were not necessarily in the same order as in the genome. The BAC vector did not rearrange DNA, and could carry more DNA than many other types of genomic clone.
The third stage was made easier by development of high-throughput DNA sequencing and affordable computing power to enable reassembly of the sequence fragments. It was these developments that led to the idea of whole genome shotgun sequencing of the human genome. In contrast to the HGP plan involving the use of genetic contigs and physical maps as a framework for genomic clones and sequence, scientists suggested that the whole genome could be fragmented into small chunks for sequencing, and then reassembled using overlap between fragment sequences (whole genome shotgun sequencing). This required large amounts of computing power to generate the correct assembly, but was considerably faster than the HGP approach. Many scientists did not believe that this method would assemble the genome properly, and suggested that overlap between small fragments could not be the only guide to assembly, because the genome contained many repeated DNA sequences. However, American biochemist J. Craig Venter believed the method could work, and formed Celera, a private company that would sequence the human genome before the HGP. Celera demonstrated that the whole genome shotgun method would work by sequencing the genome of a model organism, the fruit fly Drosophila melanogaster. Despite the successful sequencing of the fly, many people were still skeptical that the method would be successful for the bigger human genome. The publicly funded HGP, in light of Celera’s competition, decided to concentrate, like Celera, on a draft of the human genome sequence (3x coverage—that is each nucleotide has been sequenced an average of three times), before generating a more accurate map of 8x coverage. Celera had an advantage, because the HGP had agreed to release all its data as it was generated on to a freely accessible database, as part of the Bermuda rules (named after the location of a series of meetings during the early stages of the HGP). This allowed Celera to use
566 |
G A L E E N C Y C L O P E D I A O F G E N E T I C D I S O R D E R S |

HGP data to link its sequence fragments with the BAC contigs and genetic/physical maps.
The human genome draft sequence of both groups were published in February 2001 by Celera and the HGP consortium in the journals Science and Nature, respectively. Celera had imposed restrictions on access to its genomic data, and this was a source of disagreement between the private company and the HGP. Celera scientists argue that their methods are cheaper and quicker than the HGP framework method, but HGP scientists, in turn, argue that Celera’s assembly would not have been possible without the HGP data.
For human geneticists in general, and medical researchers in particular, the genome sequence is abundantly useful. Even in its draft form (the complete version is due in 2003) the ability to identify genes, single nucleotide polymorphisms, from a database search speeds up research. Previously, mapping and finding (positional cloning) a gene would take several years of research, a task which now takes several minutes. The investment in the sequencing centers will continue to be of use, with a mouse sequencing project underway, and many genomes of pathogenic bacteria sequenced. This study of genomes and parts of genomes has been called genomics. The medical benefits of genomics were emphasized throughout the project partly to ensure continuing government support. These benefits are not likely to be immediate nor direct, but the genome sequence will have the greatest effect on pharmocogenetics, which studies how genetic variants can affect how well a drug can treat a disease. The impact on non-scientists has been substantial, with the HGP suggested to be the ultimate in self knowledge. Although the mapping of the human genome by the HGP is an important scientific achievement, WIMR director Eric Lander offered a humbling perspective regarding the amount of information yet to be discovered by future generations of scientists. In a speech at the White House, Lander said, “We’ve called the human genome the blueprint, the Holy Grail, all sorts of things. It’s a parts list. If I gave you the parts list for the Boeing 777, and it has 100,000 parts, I don’t think you could screw it together, and you certainly wouldn’t understand why it flew.”
Edward J. Hollox, PhD
I Hunter syndrome
Definition
Hunter syndrome is a defect in the ability to metabolize a type of molecule known as a mucopolysaccharide. Only males are affected. Short stature, changes in the
K E Y T E R M S
Kyphosis—An abnormal outward curvature of the spine, with a hump at the upper back.
Mucopolysaccharide—A complex molecule made of smaller sugar molecules strung together to form a chain. Found in mucous secretions and intercellular spaces.
normal curvature of the spine (kyphosis), a distinctive facial appearance characterized by coarse features, an oversized head, thickened lips, and a broad, flat nose characterize the syndrome.
Description
Hunter syndrome is a one of a group of diseases called mucopolysaccharidoses. It is caused by the deficiency of an enzyme that is required to metabolize or break down mucopolysaccharides (also called glycosaminoglycans). It is also called mucopolysaccharidosis Type II (MPS II) because there are several related but similar diseases. The Hunter syndrome involves a defect in the extracellular matrix of connective tissue. One of the components of the extracellular matrix is a molecule called a proteoglycan. Like most molecules in the body, it is regularly replaced. When this occurs, one of the products is a class of molecules known as mucopolysaccharides (glycosoaminoglycans). Two of these are important in Hunter syndrome: dermatan sulfate and heparan sulfate. These are found in the skin, blood vessels, heart and heart valves (dermatan sulfate) and lungs, arteries and cellular surfaces (heparan sulfate). The partially brokendown molecules are collected by lysosomes and stored in various locations in the body. Over time, these accumulations of partially metabolized mucopolysaccharides impair the heart, nervous system, connective tissue, and bones.
Both of these molecules require the enzyme iduronate-2-sulfatase (I2S) to be broken down. In people with Hunter syndrome, this enzyme is partially or completely inactive. As a result, unchanged molecules accumulate in cells. These mucopolysaccharides are stored and interfere with normal cellular functions. The rate of accumulation is not the same for all persons with Hunter syndrome. Variability in the age of onset is thought to be due to lingering amounts of activity by this enzyme.
The cells in which mucopolysaccharides are stored determine the symptoms that develop. When mucopolysaccharides are stored in skin, the proportions of the face change (coarser features than normal and an enlarged
syndrome Hunter
G A L E E N C Y C L O P E D I A O F G E N E T I C D I S O R D E R S |
567 |
Hunter syndrome
head). When they are stored in heart valves and walls, cardiac function progressively declines. If intact mucopolysaccharides are stored in airways of the lung, difficulty in breathing develops due to obstruction of the upper airway. Storage of the molecules in joints decreases mobility and dexterity. Storage in bones results in decreased growth and short stature. As mucopolysaccharides are stored in the brain, levels of mental functioning decline.
There are two variants of Hunter syndrome: a severe form (MPSIIA) and a mild form (MPSIIB). These can be diagnosed early in life and are distinguished on the basis of mental and behavioral differences. External manifestations of the severe form occur between two and four years of age and the mild form later, up to age 10.
Genetic profile
In both variants, the missing enzyme is L- Sulfoiduronate. Hunter syndrome is X-linked meaning that the I2S gene is located on the X chromosome. The Y chromosome of a male is never affected in Hunter syndrome. Males only have one copy of the I2S gene while females have two. A male who inherits an abnormal I2S gene will develop Hunter syndrome. This can occur in two ways: from a mother who already has the gene (she is a carrier) or from a fresh mutation. Fresh mutations are unusual.
There are four possible genetic configurations. (1) A male can have a normal I2S gene and will be unaffected.
(2) A male can have an abnormal I2S gene and will have Hunter syndrome. Should this male reproduce, his sons will not have Hunter syndrome and his daughters will all be carriers. (3) A female can have two normal I2S genes and be unaffected. (4) A female can have one abnormal I2S gene and be a carrier. Should this female reproduce, half of her sons will, on average, have Hunter syndrome. Half of her daughters, on average, will be carriers. It is possible that no sons will have Hunter syndrome or no daughters will be carriers.
Demographics
Several estimates of the incidence of Hunter syndrome have been published. They vary from one in 72,000 male births (Northern Ireland) to one in 150,000 (United States). Because it is carried on the X chromosome, only males can be affected.
Signs and symptoms
Individuals with Hunter syndrome experience a slowing of growth between one and four years of age.
They attain an average height of 4-5 feet (122-152 cm). The facial features of persons with Hunter syndrome are coarser than normal. Their heads tend to be large in proportion to their bodies. Over time, their hands tend to become stiff and assume a claw-like appearance. Their teeth are delayed in erupting. Progressive hearing loss eventually leads to deafness. Internal organs such as the liver and spleen are larger than normal. They are quite prone to hernias.
Diagnosis
Hunter syndrome can be identified early in life and is often initially diagnosed by the presence of an enlarged liver and spleen (hepatosplenomegaly), hernias, or joint stiffness. Skeletal changes can be seen with radiographs. Elevated mucopolysaccharide levels in urine focuses the diagnosis to a group of disorders. The concentration of dermatan sulfate and heparan sulfate is 5-25 times higher than in normal urine. Both are present in approximately the same amounts. The diagnosis of Hunter syndrome is confirmed by measuring iduronate-2-sulfatase activity in white blood cells, serum, or skin fibroblasts. Prenatal diagnosis is widely available by measuring the activity of I2S enzyme in amniotic fluid.
Hunter syndrome has many diagnostic characteristics in common with Hurler syndrome. However, there are some distinct differences between the two syndromes. Individuals with Hunter syndrome have clear corneas and tend to have deposits of mucopolysaccharides in the skin. These are characteristically on the back of the hands and elbows (the extensor surfaces) and on the upper surfaces of the shoulders. All are males. These differences are important in diagnosis.
Treatment and management
General support and treatment of specific symptoms are the only treatment options presently available. Iduronate-2-sulfatase can be made using cells that have been genetically engineered. However, as of 2001, the safety and clinical effectiveness of injecting I2S into humans has not been established.
Intrauterine testing of amniotic fluid is reliable. Tests to detect a carrier state are imperfect. There is no cure for Hunter syndrome. The heparan sulfate and dermatan sulfate in urine has no pathological significance.
Prognosis
In the severe form, death usually occurs by age 1015. Persons with the mild form usually live near-normal lives and have normal intelligence.
568 |
G A L E E N C Y C L O P E D I A O F G E N E T I C D I S O R D E R S |

Resources
BOOKS
Jones, K. L. “Hunter Syndrome.” In Smith’s Recognizable Patterns of Human Malformation. Edited by Kenneth L. Jones and Judy Fletcher. 5th ed. Philadelphia: Saunders, 1997, pp. 462-463.
McGovern, Margaret M., and Robert J. Desnick. “Lysosomal storage diseases.” In Cecil Textbook of Medicine. Edited by Lee Goldman, et al. 21st ed. Philadelphia: Saunders, 1999, pp. 1104-1108.
Muenzer, Joseph L. “Mucopolysaccharidoses.” In Nelson Textbook of Pediatrics. Richard E. Behrman et al., 16th ed. Philadelphia: Saunders, 2000, pp. 420-423.
PERIODICALS
Hunter, C. “A rare disease in two brothers.” Proceedings of the Royal Society of Medicine. 1917: 10:104.
ORGANIZATIONS
Alliance of Genetic Support Groups. 4301 Connecticut Ave. NW, Suite 404, Washington, DC 20008. (202) 966-5557. Fax: (202) 966-8553. http://www.geneticalliance.org .
Canadian Society for Mucopolysaccharide and Related Diseases. PO Box 64714, Unionville, ONT L3R-OM9 Canada. (905) 479-8701 or (800) 667-1846. http://www
.mpssociety.ca .
Children Living with Inherited Metabolic Diseases. The Quadrangle, Crewe Hall, Weston Rd., Crewe, Cheshire, CW1-6UR UK. 127 025 0221. Fax: 0870-7700-327.http://www.climb.org.uk .
National MPS Society. 102 Aspen Dr., Downingtown, PA 19335. (601) 942-0100. Fax: (610) 942-7188. info @mpssociety.org. http://www.mpssociety.org .
National Organization for Rare Disorders (NORD). PO Box 8923, New Fairfield, CT 06812-8923. (203) 746-6518 or (800) 999-6673. Fax: (203) 746-6481. http://www
.rarediseases.org .
Society for Mucopolysaccharide Diseases. 46 Woodside Rd., Amersham, Buckinghamshire, HP6 6AJ UK. 44 (01494) 434156. http://www.mpssociety.co.uk .
WEBSITES
“Hunter syndrome.” Transkaryotic Therapies, Inc. http:// www.tktx.com/c_patient_hunter.htm .
“Hunter syndrome.” University of Maryland Medicine.
http://umm.drkoop.com/conditions/ency/article/001203
.htm .
National MPS Society. http://www.mpssociety.org/mps2
.htm .
L. Fleming Fallon, Jr., MD, DrPH
Huntington chorea see Huntington disease
I Huntington disease
Definition
Huntington disease is a progressive, neurodegenerative disease causing uncontrolled physical movements and mental deterioration. The disease was discovered by George Huntington of Pomeroy, Ohio, who first described a hereditary movement disorder.
Description
Huntington disease is also called Huntington chorea, from the Greek word for “dance,” referring to the involuntary movements that develop as the disease progresses. It is occasionally referred to as “Woody Guthrie disease” for the American folk singer who died from it. Huntington disease (HD) causes progressive loss of cells in areas of the brain responsible for some aspects of movement control and mental abilities. A person with HD gradually develops abnormal movements and changes in cognition (thinking), behavior, and personality.
Demographics
The onset of symptoms of HD is usually between the ages of 30 and 50, although in 10% of cases, onset is in late childhood or early adolescence. Approximately 30,000 people in the United States are affected by HD, with another 150,000 at risk for developing this disorder. The frequency of HD is four to seven per 100,000 persons.
Genetic profile
Huntington disease is caused by a change in the gene (an inherited unit which contains a code for a protein) of unknown function called huntingtin. The nucleotide codes (building blocks of genes arranged in a specific code that chemically form proteins), contain CAG repeats (40 or more of these repeat sequences). The extra building blocks in the huntingtin gene cause the protein that is made from it to contain an extra section as well. It is currently thought that this extra protein section, or portion, interacts with other proteins in brain cells where it occurs, and that this interaction ultimately leads to cell death.
The HD gene is a dominant gene, meaning that only one copy of it is needed to develop the disease. HD affects both males and females. The gene may be inherited from either parent, who will also be affected by the disease. A parent with the HD gene has a 50% chance of passing it on to each offspring. The chances of passing on the HD gene are not affected by the results of previous pregnancies.
disease Huntington
G A L E E N C Y C L O P E D I A O F G E N E T I C D I S O R D E R S |
569 |
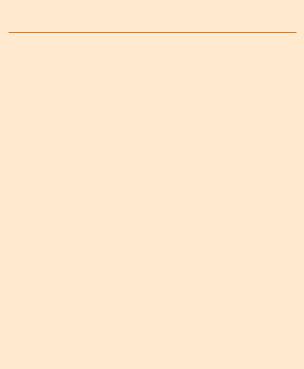
Huntington disease
K E Y T E R M S
Cognition—The mental activities associated with thinking, learning, and memory.
Computed tomography (CT) scan—An imaging procedure that produces a three-dimensional picture of organs or structures inside the body, such as the brain.
Deoxyribonucleic acid (DNA)—The genetic material in cells that holds the inherited instructions for growth, development, and cellular functioning.
Heimlich maneuver—An action designed to expel an obstructing piece of food from the throat. It is performed by placing the fist on the abdomen, underneath the breastbone, grasping the fist with the other hand (from behind), and thrusting it inward and upward.
Neurodegenerative—Relating to degeneration of nerve tissues.
Signs and symptoms
The symptoms of HD fall into three categories: motor or movement symptoms, personality and behavioral changes, and cognitive decline. The severity and rate of progression of each type of symptom can vary from person to person.
Early motor symptoms include restlessness, twitching and a desire to move about. Handwriting may become less controlled, and coordination may decline. Later symptoms include:
•Dystonia, or sustained abnormal postures, including facial grimaces, a twisted neck, or an arched back.
•Chorea, in which involuntary jerking, twisting, or writhing motions become pronounced.
•Slowness of voluntary movements, inability to regulate the speed or force of movements, inability to initiate movement, and slowed reactions.
•Difficulty speaking and swallowing due to involvement of the throat muscles.
•Localized or generalized weakness and impaired balance ability.
•Rigidity, especially in late-stage disease.
Personality and behavioral changes include depression, irritability, anxiety and apathy. The person with HD may become impulsive, aggressive, or socially withdrawn.
Cognitive changes include loss of ability to plan and execute routine tasks, slowed thought, and impaired or inappropriate judgment. Short-term memory loss usually occurs, although long-term memory is usually not affected. The person with late-stage HD usually retains knowledge of his environment and recognizes family members or other loved ones, despite severe cognitive decline.
Diagnosis
Diagnosis of HD begins with a detailed medical history, and a thorough physical and neurological exam. Family medical history is very important. Magnetic resonance imaging (MRI) or computed tomography scan (CT scan) imaging may be performed to look for degeneration in the basal ganglia and cortex, the brain regions most affected in HD.
A genetic test is available for confirmation of the clinical diagnosis. In this test, a small blood sample is taken, and DNA from it is analyzed to determine the CAG repeat number. A person with a repeat number of 30 or below will not develop HD. A person with a repeat number between 35 and 40 may not develop the disease within their normal lifespan. A person with a very high number of repeats (70 or above) is likely to develop the juvenile-onset form. An important part of genetic testing is extensive genetic counseling.
Prenatal testing is available. A person at risk for HD (a child of an affected person) may obtain fetal testing without determining whether she herself carries the gene. This test, also called a linkage test, examines the pattern of DNA near the gene in both parent and fetus, but does not analyze for the triple nucleotide repeat (CAG). If the DNA patterns do not match, the fetus can be assumed not to have inherited the HD gene, even if present in the parent. A pattern match indicates the fetus probably has the same genetic makeup of the at-risk parent.
Treatment and management
There is no cure for HD, nor any treatment that can slow the rate of progression. Treatment is aimed at reducing the disability caused by the motor impairments, and treating behavioral and emotional symptoms.
Physical therapy is used to maintain strength and compensate for lost strength and balance. Stretching and range of motion exercises help minimize contracture, or muscle shortening, a result of weakness and disuse. The physical therapist also advises on the use of mobility aids such as walkers or wheelchairs.
Motor symptoms may be treated with drugs, although some studies suggest that anti-chorea treatment rarely improves function. Chorea (movements caused by abnormal muscle contractions) can be suppressed with
570 |
G A L E E N C Y C L O P E D I A O F G E N E T I C D I S O R D E R S |

|
|
|
|
|
Huntington Disease |
|
|
Huntington |
||
|
|
|
|
|
|
|
|
|
= Affected Symptomatic individual |
|
|
|
|
|
|
|
|
|
|
= Affected Presymptomatic individual |
|
|
|
|
|
|
|
|
|
|
disease |
|
|
|
|
|
|
|
|
|
|
|
|
|
|
|
d.74y |
d.59y |
|
|
|
|
|
|
|
|
|
dx.68y |
dx.50y |
|
|
|
|
||
|
|
|
|
40y |
44y |
|
|
|
|
|
62y |
60y d.58y |
54y |
53y |
d.48y |
46y |
d.50y |
|
|
|
|
|
dx.48y |
|
|
dx.46y |
|
dx.42y |
|
|
|
|
|
d.30y |
|
|
31y |
28y |
32y |
d.30y |
27y |
26y |
|
|
dx.28y |
|
|
|
|
|
dx.29y |
|
|
|
(Gale Group)
drugs that deplete dopamine, an important brain chemical regulating movement. As HD progresses, natural dopamine levels fall, leading to loss of chorea and an increase in rigidity and movement slowness. Treatment with L-dopa (which resupplies dopamine) may be of some value. Frequent reassessment of the effectiveness and appropriateness of any drug therapy is necessary.
Occupational therapy is used to design compensatory strategies for lost abilities in the activities of daily living, such as eating, dressing, and grooming. The occupational therapist advises on modifications to the home that improve safety, accessibility, and comfort.
Difficulty swallowing may be lessened by preparation of softer foods, blending food in an electric blender, and taking care to eat slowly and carefully. Use of a straw for all liquids can help. The potential for choking on food is a concern, especially late in the disease progression. Caregivers should learn the use of the Heimlich maneuver. In addition, passage of food into the airways increases the risk for pneumonia. A gastric feeding tube may be needed, if swallowing becomes too difficult or dangerous.
Speech difficulties may be partially compensated by using picture boards or other augmentative communication devices. Loss of cognitive ability affects both speech production and understanding. A speech-language pathologist can work with the family to develop simplified and more directed communication strategies, including speaking slowly, using simple words, and repeating sentences exactly.
Early behavioral changes, including depression and anxiety, may respond to drug therapy. Maintaining a
calm, familiar, and secure environment is useful as the disease progresses. Support groups for both patients and caregivers form an important part of treatment.
Experimental transplant of fetal brain tissue has been attempted in a few HD patients. Early results show some promise, but further trials are needed to establish the effectiveness of this treatment.
Prognosis
The person with Huntington disease may be able to maintain a job for several years after diagnosis, despite the increase in disability. Loss of cognitive functions and increase in motor and behavioral symptoms eventually prevent the person with HD from continuing employment. Ultimately, severe motor symptoms prevent mobility. Death usually occurs 15–20 years after disease onset. Progressive weakness of respiratory and swallowing muscles leads to increased risk of respiratory infection and choking, the most common causes of death. Future research in this area is currently focusing on nerve cell transplantation.
Resources
BOOK
Watts, R. L., and W. C. Koller, eds. Movement Disorders. New York: McGraw-Hill, 1997.
ORGANIZATION
Huntington Disease Society of America. 140 W. 22nd St. New
York, NY 10011. (800) 345-HDSA.
Laith F. Gulli, MD
G A L E E N C Y C L O P E D I A O F G E N E T I C D I S O R D E R S |
571 |

Hurler syndrome
I Hurler syndrome
Definition
Hurler syndrome is a disorder that results when cells cannot break down two by-products of normal metabolism. These byproducts, dermatan sulfate and heparan sulfate, build up and disrupt normal cell function, leading to severe disease. The disease affects most body systems, causing progressive deterioration of tissues and organs.
Description
Though present from conception, Hurler syndrome may be undetectable at birth. The newborn often looks healthy and seems to develop normally for the first few months. However, symptoms begin to appear around the age of six months, when dermatan sulfate and heparan sulfate reach dangerous levels.
Individuals with Hurler syndrome lack sufficient amounts of the enzyme needed to break down dermatan sulfate and heparan sulfate. This enzyme, alpha-L- iduronidase, is part of a biochemical pathway which splits complex molecules into smaller, recyclable units. Without alpha-L-iduronidase, the complex molecules cannot be eliminated and deposit themselves in cells, tissues, and organs. Deposits in the soft tissues of the face lead to a typical appearance, causing children with Hurler syndrome to resemble each other more than they resemble their own healthy siblings. The spleen and liver become enlarged early in the course of the disease. Deposits stored in the growth plates of bones lead to dwarfism, scoliosis, joint stiffness, and other skeletal abnormalities. Corneal clouding caused by the deposits results in vision damage. Hearing loss usually occurs as well. Deposits in the brain cause loss of skills gained early in life, and severe mental retardation occurs.
The accumulation of dermatan sulfate and heparan sulfate in the airways leads to frequent respiratory tract and ear infections. Deposits also cause coronary artery obstruction and damage to the heart. In fact, respiratory complications and heart failure are the most frequent causes of death in Hurler syndrome patients. Many children with Hurler syndrome die by the age of 12.
Dermatan sulfate and heparan sulfate belong to a class of complex molecules known as mucopolysaccharides, chains formed by smaller sugar molecules strung together. For this reason, Hurler syndrome is also known as a mucopolysaccharidosis, a name meaning, “too many mucopolysaccharides.” To be precise, Hurler syndrome is called Mucopolysaccharidosis I H (MPS I H). There are several other mucopolysaccharidoses, each resulting from absence or deficiency of a different enzyme.
Sometimes Hurler syndrome is called a lysosomal storage disease. Lysosomes are cell parts which normally contain enzymes needed to break down complex molecules. When the enzymes are absent or deficient, the lysosomes store the complex molecules, expand, and eventually destroy the cells from within.
Hurler syndrome takes its most commonly used name from Gertrud Hurler, the German pediatrician who first described the condition in her patients.
Genetic profile
Researchers have identified the gene responsible for Hurler syndrome and have mapped it to the 4p16.3 site on chromosome 4. The gene is named IDUA, for the iduronidase enzyme which it produces when working properly. As of 2001, researchers have connected 52 different IDUA mutations to cases of Hurler syndrome.
Hurler syndrome is an autosomal recessive disorder. This means that it occurs only when a person inherits two defective copies of the IDUA gene. If one copy is normal and the other has a mutation, the person does not have Hurler syndrome. However, the person carries the mutated gene and can pass it on to the next generation.
Carriers of IDUA mutations have only one working gene. As a result, these carriers produce less alpha-L- iduronidase enzyme than do people with two normal IDUA genes. Nevertheless, they produce enough enzyme to break down dermatan sulfate and heparan sulfate, so disease does not occur.
Demographics
Hurler syndrome affects males and females of all races and ethnic groups. It is a rare disorder, occurring in about one out of 100,000 people.
Different IDUA gene mutations appear more frequently in certain populations. For instance, two specific mutations account for most Hurler syndrome cases among Northern Europeans, while two other mutations appear most often in Japanese patients.
Signs and symptoms
A child with Hurler syndrome may be born with a hernia. In fact, hernia is often the first sign of this disorder. However, since it can also occur in other conditions or as an isolated event, it does not immediately point to Hurler syndrome.
Other symptoms appear within six to twelve months of birth. Tissue damage in airways leads to breathing difficulties and frequent respiratory and ear infections. The child’s face begins to take on the coarse, typical features
572 |
G A L E E N C Y C L O P E D I A O F G E N E T I C D I S O R D E R S |

of Hurler syndrome. The skull appears large and unusually shaped, scalp veins are prominent, and the bridge of the nose is flat. The lips are large and the mouth is frequently open due to an enlarged, protruding tongue. Teeth may be late to emerge and are usually small, short, widely spaced, and somewhat malformed. The earlobes are thick, and the eyelids are full.
Skeletal abnormalities begin to appear. The hands are broad, with short, stubby fingers. Joints are often stiff and may limit the child’s movement. The neck is very short; the spine is crooked and bends outward, resulting in a hunchback appearance.
Children under the age of one may already show signs of heart disease. This is usually due to tissue damage in the arteries or valves of the heart, caused by accumulation of dermatan sulfate and heparan sulfate. Accumulation also causes the liver and spleen to become severely enlarged, but these organs continue to function normally.
Hurler syndrome has a devastating effect on mental development. By the age of one or two, developmental delay occurs. The child may make slow progress for a few more years, but then actually begins to lose skills gained earlier. The mental capacity of a person with Hurler syndrome is similar to that of a normal three-year- old. Deterioration of the senses makes this situation worse. Corneal clouding damages vision. Hearing loss, narrowed airways, and enlarged tongue contribute to poor language skills.
Many infants with Hurler syndrome grow quickly during their first few months. However, skeletal abnormalities and progressive tissue damage cause growth to slow down and then to stop before it should. As a result, most people with Hurler syndrome do not grow beyond four feet tall.
Diagnosis
Hurler syndrome shares many symptoms with other mucopolysaccharidoses and with different lysosomal storage diseases. For this reason, laboratory tests are used to confirm Hurler syndrome diagnosis based on a physical exam.
The simplest test available is urine screening. People with Hurler syndrome excrete increased amounts of dermatan sulfate and heparan sulfate in their urine. In addition, a blood test reveals deficiency of alpha-L- iduronidase enzyme. White blood cells and skin cells can be microscopically examined for damage caused by deposits of dermatan sulfate and heparan sulfate.
If Hurler syndrome is present in a family, healthy family members could carry a mutated IDUA gene.
K E Y T E R M S
Alpha-L-iduronidase—An enzyme that breaks down dermatan sulfate and heparan sulfate. People with Hurler syndrome do not make enough of this enzyme.
Hernia—A rupture in the wall of a body cavity, through which an organ may protrude.
Lysosome—Membrane-enclosed compartment in cells, containing many hydrolytic enzymes; where large molecules and cellular components are broken down.
Mucopolysaccharide—A complex molecule made of smaller sugar molecules strung together to form a chain. Found in mucous secretions and intercellular spaces.
Mucopolysaccharidosis I H (MPS I H)—Another name for Hurler syndrome.
Tracheostomy—An opening surgically created in the trachea (windpipe) through the neck to improve breathing.
Several clinical laboratories offer carrier screening to these individuals. A blood sample is all that is required. Most labs screen for carrier status by measuring the level of the alpha-L-iduronidase enzyme. Levels are lower in carriers than they are in people who have two normal IDUA genes. It is also possible to examine the actual genes to see if a Hurler syndrome mutation appears.
Since Hurler syndrome is a rare disorder, most carriers have children with non-carrier partners. Thus there is generally no risk of the disease occurring in the children. However, if two carriers have children together, each child has a 25% chance of having Hurler syndrome. Carrier screening provides an opportunity to assess the risk and consider reproductive options before pregnancy occurs.
Each child born to two carriers has a 50% risk of inheriting one mutated gene and one normal gene. This child, like the parents, is a carrier.
Because a rare autosomal recessive gene can be passed for generations before two carriers have a child together, sometimes an affected child is born into a family with no previous history of Hurler syndrome. This is generally an indication that both parents carry a mutated IDUA gene. These parents worry not only about the health of the affected child, but also about the risk to future children.
syndrome Hurler
G A L E E N C Y C L O P E D I A O F G E N E T I C D I S O R D E R S |
573 |
Hurler syndrome
Prenatal testing is available to find out if a fetus has Hurler syndrome. This can be done by amniocentesis or chorionic villus sampling. Amniocentesis involves removal of a small amount of amniotic fluid from the uterus. Chorionic villus sampling involves removal of a small sample of placental tissue. In either case, the cells present in the sample are checked for enzyme deficiency or gene mutations.
Treatment and management
Treatment of individual Hurler syndrome symptoms does not cure the disease, but it does offer some relief. Surgical repair is available to correct a hernia. Hearing aids sometimes improve hearing and language skills, and eyeglasses may enhance eyesight. Some children with Hurler syndrome improve communication skills by learning sign language.
Skeletal abnormalities require attention, especially if they affect the upper part of the spine and compress the spinal cord. Spinal cord compression and storage of dermatan sulfate and heparan sulfate in the surrounding membranes cause fluid to accumulate in the brain. Brain damage often occurs unless this condition is corrected. A surgeon can implant a shunt in the brain to remove excess fluid. Once present, the mental retardation caused by Hurler syndrome is generally not reversible.
It is important to protect the upper back and neck of a patient with Hurler syndrome. This area should not be manipulated during chiropractic or physical therapy. If the patient undergoes anesthesia for any reason, care should be taken to support the neck and upper back at all times.
Orthopedic treatment can help reduce joint stiffness and its effects on movement.
Several options are available to correct breathing difficulties. Some patients respond well to oxygen treatments. Others require tonsillectomy, adenoidectomy or tracheostomy to remove upper airway obstruction. Medications are available to treat common respiratory infections.
If heart disease is limited to valve damage, valve replacement may be an option for some patients with Hurler syndrome.
Children with Hurler syndrome are generally easygoing and affectionate. They benefit greatly from safe and caring environments. Community support and social services can improve the quality of life for the entire family unit. The family of a child with Hurler syndrome experiences grief and loss throughout the lifetime and upon the death of the child. Genetic counseling is available to offer support, educate families about the disease,
and assess the risk to other family members. The National MPS Society provides additional support and information.
As of 2001, bone marrow transplant (BMT) is the only treatment that appears to improve the long-term outcome of children with Hurler syndrome. BMT replaces the child’s entire blood system with the blood system of a healthy person. The healthy bone marrow contains stem cells, cells from which other cells and tissues arise. These cells produce enough alpha-L-iduronidase to break down dermatan sulfate and heparan sulfate.
Bone marrow transplant is a complicated procedure. If the donated bone marrow is not compatible with the child’s own body tissues, the child’s immune system will destroy it. BMT is most successful if the donor is a close relative of the patient, since this increases the chance of compatibility between donor and patient bone marrow. To reduce the risk of donor bone marrow rejection, the patient receives drugs and radiation to suppress the immune system, leaving the patient vulnerable to infection.
Research indicates that children with Hurler syndrome do better if BMT takes place before the age of two. Beyond that point, prevention or correction of brain damage is unlikely, and other body tissues may be so severely affected that the child would not survive BMT.
Prognosis
As of 2001, bone marrow transplant is the only treatment that can prevent or reduce the effects of Hurler syndrome. However, bone marrow transplant is not an option for every patient. Some patients with severe disease are too weak to survive the transplant procedure or recovery period. For some, a donor match is not available. Others don’t have access to the technological or medical expertise needed for the procedure. In addition, some patients who have bone marrow transplants reject the donor cells.
Research into long-term therapies is underway. Two which appear promising are enzyme replacement therapy and gene therapy.
Enzyme replacement involves giving the patient a substitute for the deficient enzyme. The patient would receive regular enzyme injections, similar to insulin injections used by people with diabetes. Enzyme replacement is complicated in a disorder which affects many different tissues, as Hurler syndrome does. Each tissue interacts differently with the enzyme. For this reason, it is difficult to design a substitute which works with various tissues. Furthermore, the brain has a natural barrier against outside substances. This is called the blood-brain barrier, and it stops the enzyme substitute from reaching
574 |
G A L E E N C Y C L O P E D I A O F G E N E T I C D I S O R D E R S |