

6. Dipole moments of compounds containing double bonds |
281 |
Alternatively, the intramolecular hydrogen bond can be represented as a very fast or even somewhat slower equilibrium between two positions of hydrogen (46 46a). The observed mexp would then depend on the dipole moments of the two forms according to vector equation 3 for a fast equilibrium, or (nonvector) equation 4 for a slow equilibrium. Slow or fast here means with respect to the rate of orientation of a molecule in solution. This explanation could be correct for other molecules with stronger hydrogen acceptors137 but in the case of compounds 46 it was not in agreement with dipole moment values138.
mexp D 1 |
pmBM C pmHB |
(3) |
2exp D 1 |
p2BM C p2HB |
(4) |
In the hydrazones of 2-bromo-1-phenylglyoxal (47) the dipole moment produced by the hydrogen bond was still much larger131 since the geometry in the six-membered ring is more favorable. In all these studies it was necessary to have a priori good spectroscopic evidence of the intramolecular hydrogen bond.
O....H
X C N Y
C N
Br
mH 3.4
(47)
B. Conjugated and Cumulated Systems C−C=O
When a CDO bond is conjugated with CDC, the dipole moment increases. The classical explanation by participation of the polar mesomeric formula agrees with all known facts. The conjugation is stronger and the dipole moment greater in the conformation ap than in sp (stereoelectronic effect). Good examples are the stereoisomers of propenal (48) investigated by microwave spectroscopy139. The greater in the ap conformation is accompanied by the shorter C C bond showing more double-bond character. In 49 the
|
|
|
|
|
|
CH3 |
O |
H |
H |
H |
|
O |
O |
O |
CH3 |
|
|
|
|
||||
C |
C |
C |
C |
|
|
|
|
H C |
O |
H C |
|
H |
|
|
|
H |
|
H |
|
O |
|
O |
|
exp |
2.552 |
exp |
3.117 |
|
exp 4.452 calc |
3.54 |
calc 6.35 |
|
|
|
|
|
exp |
3.17 |
|
(48a) |
(48b) |
(49) |
(50a) |
(50b) |

282 |
Otto Exner |
conjugation is crossed, though is very high: this relatively large molecule was still investigated by MW140.
In an interesting example, the stereoelectronic effect was exploited for determining conformation on the central, partially double bond141. Dipole moments of 50a,b were calculated at the semiempirical level and compared with the experiment: the conformation sp was revealed, the same as in the crystalline phase. Of course, conjugation is strengthened by the donor atom at the other end of the conjugated system. This effect is still more expressed142 in the quinone methide derivatives 51, which are conformationally rigid. In addition, the strengthened conjugation was observed also in the dipole moments in the excited state and in the UV spectra142. In cyanochalcones 52 and 53 dipole moments were used, in combination with 1H NMR, for determining E configuration on the CDC143, and further, for estimating conformation on C C. The latter was near to planar ap as shown in the formulas, but agreement with the calculations was not good143. In the long conjugated chain of retinal, configurations on CDC are well known: as expected of the all-E isomer was higher than of the all-Z isomer144 but the difference was small (4.02 against 3.89 D).
X X
X X
O |
O |
NC |
NC |
|
O |
|
|
|
|
|
|
|
|
|
CH3 |
X = CH3 |
exp |
4.60 |
X = H |
exp 3.94 |
exp 4.29 |
X = NC5H11 |
exp |
10.8 |
|
|
|
(51) |
|
|
(52) |
(53) |
Conjugation of a carbonyl bond with an aromatic ring may be already at the border of our subject, but has been studied rather extensively from the points of view of both mesomerism and conformation. Generally, conjugation is strong enough to keep coplanarity of the CDO bond with the ring but can be distorted by steric hindrance. In tetraalkyl derivatives 54 a decrease of dipole moments was observed145 from methyl to t-butyl, and interpreted by a nonplanar conformation with increasing torsional angle from 49° to 90°. These values should not be considered as quite realistic, rather as some effective mean, although they were confirmed also by 1H NMR spectroscopy. The same trend was observed146 in trialkyl derivatives 55 and compared to similar effects in 13C NMR spectra. In another dipole moment study these acetophenone derivatives were taken as standard in which the conjugation and also its steric hindrance are evident; they were also compared to other derivatives, particularly nitro compounds which are probably not conjugated or only very weakly147. Some kind of steric hindrance was also anticipated in acetophenone
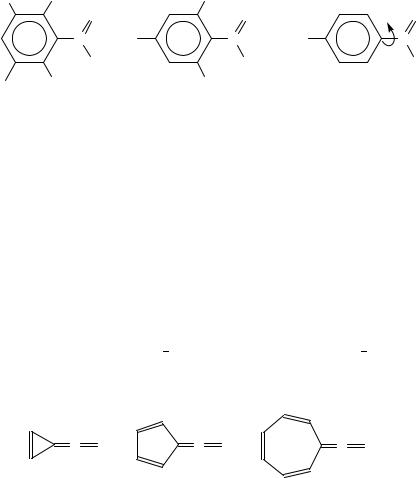
|
6. Dipole moments of compounds containing double bonds |
283 |
||||
CH3 |
CH3 |
|
R |
|
|
|
|
|
O |
|
O |
|
τ O |
|
C |
R |
|
C |
X |
C |
|
|
R |
|
CH3 |
|
CH3 |
CH3 |
CH3 |
|
R |
|
|
|
R = CH3 |
m 2.83 |
|
R = CH3 |
m 2.81 |
X = t-C4 H9 |
τ = 10° |
R = t-C4 H9 |
m 2.65 |
|
R = t-C4 H9 |
m 2.52 |
X = NO2 |
τ = 20° |
(54) |
|
(55) |
|
(56) |
|
para derivatives without methyl substituents (56). In the case of certain substituents X, their conformation was claimed to be nonplanar148 but the result was dependent not only on dipole moment measurements but mainly on the Kerr constant and should be certainly confirmed by other means. In the simple molecule of benzaldehyde, any distortion of planarity cannot be forced experimentally, but can be studied theoretically149. In the nonplanar form, would be reduced by 0.38 D. In substituted benzophenones depends on the position of benzene rings which are rotated out in the same sense by unequal angles150. In substituted diacetylnaphthalenes, the coplanarity is evidently prevented by strong steric hindrance: was calculated and discussed but not measured151.
In other aromatic or heterocyclic compounds, the conjugation was not evaluated and dipole moments exploited only for determining conformation. Examples are fural and diformylfuran152: the preferred conformation was very near to free rotation.
The conjugated cyclic ketenes 57 59 are analogous to cyclic ketones 4 6 and their aromatic character was deduced from the calculated dipole moments in a similar way74. The result was that 57 is aromatic but less than 4, while 58 and 59 are not aromatic.
C O |
C O |
C O |
m calc 4.39 |
m calc 0.39 |
m calc 1.30 |
(57) |
(58) |
(59) |
Structure and conformation of the conjugated dialdehyde 60 is known from X-ray; in solution it is in equilibrium with 61. Experimental was first decomposed into the contributions of 60 and 61 (as in equation 4, of 61 was estimated from bond moments), then the strong electron displacement in 60 was revealed153. As expected, the sevenmembered ring bears some negative charge. In some ortho and para quinones, was calculated154 but not analyzed in terms of conjugation.
In 1,2-diketones or their functional derivatives no conjugation is apparent in the dipole moment values, but these can serve for determining conformation on the central, formally single bond. In (E)- and (Z)-benzil monoximes (62) the configuration on CDN was known, and the ap conformation on N O was assumed (and finally confirmed): from dipole

284 |
Otto Exner |
|
|
O |
O |
O |
O |
|
|
H |
H |
H |
H |
m cal c |
1.25 |
m calc |
1.71 |
m exp |
5.25 |
|
|
(60) |
(61) |
moments mainly the ap conformation on C C was deduced155 for either isomer, the same as in the crystal. Deciding in this case was the possibility of para chloro substitution.
X |
|
X |
|
|
O |
|
|
|
O |
|
|
|
|
|
C |
|
|
C |
|
C |
|
O |
C |
|
N |
|
H |
N |
|
O |
X |
|
|
X |
H |
|
|
||
X = H: mcalc |
2.56 |
|
mcalc |
2.56 |
mexp |
3.16 |
|
mexp |
3.13 |
(62a) |
|
|
(62b) |
Several curious molecules with cumulated CDC bonds and a carbonyl group at the end have received attention. Problems were the separation of unstable compounds and the measuring technique, and also the direction of the dipole moments and sometimes even the geometry: the exact linearity can be doubted in some cases. In the series of compounds 63 with an increasing number of cumulated double bonds, the dipole moments (in the gas phase) change in an irregular way156; the compound with n D 2 is not linear.
H2C(DC)nDO
exp 2.332, 1.422, 2.297, 1.977
(63)
While dichloroketene (64) is practically nonpolar157, monochloroketene (65) has an appreciable moment158, noncollinear with the CDO bond but oriented with the negative end probably nearer to the oxygen. The parent ketene was investigated theoretically:

6. Dipole moments of compounds containing double bonds |
285 |
particularly interesting was its protonation (on carbon) and the dipole moment of the protonated form159. The dipole moment of an ion is an unusual concept: in principle it is possible to express an arbitrary system of charges in space as a sum of a pole, a dipole, a quadrupole etc., but in common chemical applications one such term is given a physical meaning only when the preceding terms are zero.
Cl |
H |
|
|
|
|
|
|
|
|
|
|
|
|
|
|
|
|
|
|
C C O |
C C O C |
|
C |
|
C |
|
O O |
|
C |
|
C |
|
C |
|
C |
|
C |
|
O |
|
|
|
|
|
|
|
|
|
|||||||||||
|
|
|
|
|
|
|
|
|
|||||||||||
Cl |
Cl |
|
|
|
|
|
|
|
|
|
|
|
|
|
|
|
|
|
|
mexp < 0.1 |
mexp 1.2 |
mexp 2.39 |
|
|
|
|
|
|
|
|
|
|
|
|
|
||||
(64) |
(65) |
(66) |
|
(67) |
|
|
|
|
|
The molecule C3O with an electron sextet (66) is strongly polar160: according to ab initio calculations the negative end of the dipole is on oxygen161. However, agreement with experiment was not good, even at the 6-31GŁ basis. The symmetrical molecule of 67 should be nonpolar if it is exactly linear162. Dipole moments of various derivatives 68 were only calculated163 and not measured.
O
O |
H |
O |
C |
|
C C |
C |
C |
X |
C |
X |
H |
|
|||
|
|
O |
|
X = CH3 |
m calc 2.18 |
m calc |
3.84 |
|
(68a) |
(68b) |
C. Conjugated Systems O−C=O and S−C=O
The extremely stable and general sp conformation of esters and carboxylic acids (3a) is one of the remarkable features of stereochemistry. It was observed uniformly2 in all other molecules with the group O CDO and also with their heteroatom analogues with S, Se or Te. In contrast with the rigid conformation, the conjugation in the ester molecule, expressed by the formulas 69, is relatively weak and can be just detected in the dipole moment values164.
O |
|
O− |
R1 C |
|
R1 C |
|
||
O R2 |
|
O+ R2 |
(69a) |
|
(69b) |

286 |
Otto Exner |
Stereochemical studies were oriented toward finding esters of special structure with reversed conformation and/or with the presence of the second conformer in smaller amounts. As reviewed previously2, these attempts were mostly unsuccessful and claims of the conformation ap were again rebutted. The only exception are certain formates, particularly t-butyl formate, in which more ap form is present in polar solvents6,165. A more recent dipole moment study166 assumed the ap form even in nonpolar solvents in para-substituted phenyl formates in an amount of 10%, but the uncertainty was of the same magnitude.
A further problem was the exact direction of the group moment COO. The agreement of two independent studies is not bad: 100° from the C C(O) bond (on the basis of substituted benzoates164) or 116° (from aliphatic esters referring also to the Kerr constant167). In further esters, conformation of neighboring groups was dealt with: in substituted phenyl esters and in phenyl acetates the position of the phenyl ring (from dipole moments and from the Kerr constant168,169), in chloroalkyl esters the position of the C Cl bond170. As in ketones, the dipole moment of the COO group can also be influenced by an intramolecular hydrogen bond. The hydrogen-bonded structure of glycolic acid in the gas phase (70) was determined twice171,172 but the direction of the dipole moment is not quite certain and the differences between the two studies exceed the given experimental errors.
The conformation and the dipole moments of peroxy compounds were reviewed173 in 1983. In peresters (71) it could be difficult to deal both with the conformation on the O O bond and with the conjugation inside the COO group. The problem was solved so that the conjugation was estimated to be equal as in structurally similar esters; then the conformation was determined from experimental dipole moments164. As expected, the conformation is nonplanar as the conformation of hydrogen peroxide: the dihedral angle is rather sensitive to structure (71). Steric arrangements of diacyl peroxides (72) have been reported previously2. Later the problem was reinvestigated, since the substitution from two sides allowed the geometry to be determined more exactly174. Certain quite small
H |
|
|
|
O |
|
|
|
|
|
O |
O |
X |
|
C |
|
|
|
||
CH2 |
C |
|
|
O O |
|
O H |
|
|
R |
|
|
|
R = CH3 |
τ 130° |
|
|
|
R = t-C4 H9 |
τ 170° |
(70) |
|
(71) |
|
|
|
|
O |
O |
|
X |
|
C |
C |
X |
|
|
O |
O |
|
τ 115°
(72)

6. Dipole moments of compounds containing double bonds |
287 |
differences in angle were required compared to the solid state geometry; only the dihedral angle is enlarged more, to 115° (the crystallographic value being 91°). Remarkably enough, the conformation is only slightly changed when the central atoms O O are replaced by O CH2 or by other groups175.
In peroxy acids176 (73) the hydrogen bond is manifested by a contribution to the dipole moment, mH: this is larger than in the case of ketones 46, which also have a fivemembered ring. The conformation of 73 was known previously, and the conjugation was only estimated while attention was focused on the hydrogen bond176.
|
|
|
R |
|
|
O |
H |
O |
|
X |
C |
C O |
||
|
||||
|
O |
O |
O |
|
|
|
|||
|
mH 2.46 |
|
R |
|
|
|
|
||
|
(73) |
|
(74) |
In alkyl or aryl carbonates, the problem of conformation is repeated twice on the two C O bonds linked to the same central atom. It was stated generally177 that in such cases the common conformation of the monofunctional compound is repeated in either moiety. This holds with a few exceptions and/or modifications when the molecule is strongly crowded. This is also the case of carbonates. According to this rule, the sp,sp conformation (74) was found in carbonates in two independent investigations. In the first178 para-substituted phenyls were used in place of R; the second started from the fixed conformation of trimethylene carbonate and besides the dipole moment exploited also the Kerr constant179.
Conjugation of the carboxyl group with CDC bonds affects its group moment only marginally, but conformation on the partially double bond can be sometimes determined from the overall dipole moment. In alkyl acrylates and methacrylates dipole moments were little influenced by conjugation180 and were insufficient for a decision whether or not the conjugation is hindered. In (Z)-cyanoacrylates181 the conformation 75a prevails in a ratio 2:1, in agreement with the IR spectra. In the E-isomers, the two conformers (76a,b) are indistinguishable either by dipole or by IR spectroscopy181. Electron distribution in this system was not dealt with, but it was tacitly assumed as unchanged by conjugation. Dipole moments of aromatic esters are influenced by the conjugation with the aromatic ring and by its steric hindrance182 similarly as mentioned with the aromatic ketones145 (54).
|
R |
|
|
|
|
O |
|
O |
R |
NC |
C O |
|
NC |
C O |
C |
C |
|
C C |
|
|
|
|||
|
|
|||
H |
H |
|
H |
H |
(75a) |
(75b) |

288 |
|
Otto Exner |
|
|
||
|
R |
|
|
|
|
|
|
O |
|
|
|
O |
R |
H |
C O |
|
|
H |
C |
O |
C |
C |
|
|
C |
C |
|
|
|
|
||||
|
|
|
||||
NC |
H |
|
|
NC |
H |
|
|
(76a) |
|
|
|
(76b) |
|
Conjugated systems S CDO were sometimes investigated183 together with similar compounds containing the groups O CDS and S CDS (the latter are mentioned in Section VI). The sp conformation of S-methyl thiobenzoate was determined by simple comparison with the bond-moment values184. The same result was obtained already previously with more precision, exploiting also several substituted derivatives185. The dipole moment of the trisulfide 77 was measured186 but the conformation was not speculated about, although it could be estimated from other trisulfides to be approximately as shown in 77.
O O
C S C
F S S F
(77)
D. Conjugated Systems N−C=O
Amides represent a class of compounds which, although very important, are not quite suitable for dipole moment measurement due to the low solubility and association in nonpolar solvents. These problems are avoided187,188 in substituted N,N- dimethylbenzamides (78): the dipole moments were interpreted in terms of resonance by the approach described in Section IV.B. The mesomeric dipole moment mm is appreciable187 (1.4 D) but its orientation is not from N toward O but merely toward C. If we avoid speculations, a modest conclusion would be that mesomeric formulas do not describe the structure completely. In substituted benzamides (79), mm is smaller (ca 0.6) and the conclusion less safe187. On the contrary, dipole moments of the same compounds (79) were interpreted by a nonplanar conformation; an important piece of evidence was from the Kerr constant189. This result should be accepted cautiously since some similar previous conclusions from the Kerr constant190 were in error. The dipole moment of the parent formamide was calculated at a high level191 (in connection with an investigation of its tautomeric equilibrium) but was not analyzed.
The example of amides offers us an opportunity to present the problem of mesomeric dipole moments more generally. Let us suppose that the real structure of a molecule is represented as mesomeric between the two limiting structures: the dipole moments of these structures can be anticipated as vectors A and B. The effective experimental dipole moment of the compound is then given by the vector equation 5, where p is a measure of relative importance of the two structures. Equation 5 is formally identical with equation 3 and is always valid for mesomeric structures, since these are assumed to equilibrate by

6. Dipole moments of compounds containing double bonds |
289 |
|||||
|
O |
|
|
O− |
|
|
X |
C |
|
|
X |
C |
|
|
|
|
||||
|
N CH3 |
|
|
N+ |
CH3 |
|
CH3 |
|
|
CH3 |
|
||
X = H µ exp 3.81 |
|
|
|
|
||
µ m |
1.4 |
|
|
|
|
|
(78a) |
|
|
|
|
(78b) |
|
|
|
|
|
O |
|
|
|
X |
|
C |
|
|
|
|
|
|
|
NH2 |
|
|
|
X = H |
τ = 39˚ |
|
|
||
|
X = NO2 |
τ = 0° |
|
|
||
|
(79) |
|
|
|
||
an infinite velocity. |
mexp D 1 p A C pB |
|
|
|||
|
|
5 |
Since A and B have been obtained by calculation, their directions are known. This need not apply to mexp whose direction has to be determined by more sophisticated procedures,
for instance from comparison with substituted derivatives187. Furthermore, calculation of A and B need not be equally feasible: the polar form has a much larger dipole moment whose calculation is more difficult. The problem was solved in the literature on several levels, according to the data which were available and using more or less sophisticated concepts.
Let us follow the example of N,N-dimethylformamide in Figure 2.
(1)When only A and the absolute value of mexp are known, the latter can be represented by a circle arc. When the absolute values of the vectors A and mexp differ, one can state that there is some conjugation or mesomerism (in a qualitative sense).
(2)In order to evaluate the extent of resonance quantitatively, one can use the definition of mm according to equation 1, which corresponds geometrically to constructing a triangle.
A solution is possible if the direction of mm can be anticipated96,97. In the case of amides, if one assumes the direction of mm from N toward O, one obtains mm as A1E0 in Figure 2.
(3) A better estimate is possible when the direction of mexp is known. Then the above assumption is not necessary and mm is obtained as A1E. In the example in Figure 2 the direction of mexp was transferred from N,N-dimethylbenzamide187 where it should be essentially identical: m 1.4 was obtained. The direction of mm obtained in this way need not be always identical5,98,187 as anticipated in point (2) above: in such a case one has a new result to explain.
(4) When B is also known, equation 5 can be solved for p. In geometrical terms this equation requires that the end point of mexp must lie on the line connecting the end points of A and B. When this is not fulfilled, the deviations show the inaccuracy of calculations or of the whole approach. In simple calculations, B is controlled by the two charges in

290 |
Otto Exner |
+ B2
+ B3
E E′
+ + A1
A3
+A2
O
H C
N Me
Me
FIGURE 2. Analysis of the experimental dipole moment (E) of N,N-dimethylformamide and/or N,N- dimethylbenzamide in terms of the resonance structures 78a and 78b (A and B) and of the mesomeric dipole moment ( m) according to various approximations
78b. Using the values shown by A2 (little different from A1) and B2 one obtained89 p D 0.19, m D 1.9; the full calculation was rather complex.
(5) The previous procedure may be improved by a more sophisticated calculation99 of B, taking into account induction caused by the charges in the remaining parts of the molecule. Using the values denoted A3 and B3 in Figure 2, one obtains99 p D 0.34,m D 1.3. When the above methods are compared, the most sophisticated one need not always be the best. In a concrete example, the differences are usually not dramatic (see Figure 2). In the writer’s opinion, the results possess some reliability only as far as they are near the nonpolar classical structure: then mm or p appear as small corrections. Commonly, their values are used only to compare similar systems. Then it is sufficient when all these values have been determined by the same method.