

15. Hydrogenation of compounds containing CDC, CDO and CDN bonds 811
Transfer hydrogenation of carbonyl groups is a popular method for reduction and may be mediated by a range of catalysts using an alcohol196 or formic acid197 as the hydride source. Microwaves have been shown to accelerate the reaction197. Iridium complexes have proved to be some of the most suitable for the reduction of enones to allylic alcohols198. Whilst several neutral complexes have been used, o-dimethylaminodiphenylphosphine appears to be a particularly suitable ligand which generates a very high degree of chemoselectivity in the reduction198a.
1,3,5-Triaza-7-phosphaadamantane 1, which has been described in a previous section, imparts aqueous solubility on the hydrogenation complexes of ruthenium, of which it forms a part199. The complexes thus formed are highly selective for the reduction of the CDO bond of enones, as indeed are several cluster complexes of carboxyates with Co, Mo and Cu200. The use of sulphonic acid groups on phosphines can also impart aqueous solubility on transition metal complexes, however in one report the addition of such a ligand to an aldehyde, the reduction substrate, to give a stable salt, was observed201. This side reaction would of course be far less of a problem with non-polar CDC bonds.
Whilst the use of supercritical carbon dioxide as a solvent has recently been reported, it should be noted that it can also be a substrate; one ruthenium catalyst is reported to be capable of its conversion to formic acid at the rate of 1400 mol per mol of catalyst per hour202.
2. Diastereoselective and enantioselective reductions
There has been tremendous growth in this research area in recent years, and some remarkable breakthroughs have been achieved. This section of the review will focus on hydrogenation reactions using either hydrogen gas or transfer hydrogenation methods. Hydrosilylation will not be described in detail, although it should be noted that this process generates the same overall chemical transformation as hydrogenation.
As was the case for the reduction of CDC bonds, proximal co-ordinating groups were believed to be essential for the realization of high enantioselectivities, hence early investigations have concentrated on substituted substrates. ˛-Amino substituted ketones have proved to be ideal substrates for hydrogenation, early success being achieved with the use of the ligand P28 (related to P17 in Table 1)203. Scheme 38 summarizes the reduction of a typical substrate, which benefits not only from a high level of compatibility with the reaction conditions but also from the fact that it provides a means for the synthesis of a physiologically important class of target molecule. Rhodium complexes derived from other chiral phosphines, for example (S)-BPPM (P7), and related ligands, have also been employed to good effect in similar reductions204. One example is the reduction of 41 to
OH
PPh2
Fe
PPh2
(P28) (R) (S)-BPPFOH

812 |
|
Martin Wills |
|
|
|
||
|
O |
|
|
|
|
OH |
|
HO |
NHMe.HCl |
|
HO |
|
* |
NHMe.HCl |
|
|
|
|
|
|
|
|
|
|
|
|
100% |
|
|
|
|
HO |
|
|
|
HO |
|
|
|
Reagents: (i) [Rh(P28)(NBD)]ClO4 ,H2 |
|
|
|
|
95% e.e |
|
|
|
|
|
SCHEME 38 |
|
|
|
|
Ph |
|
|
|
Ph |
|
|
|
|
N |
|
|
|
|
N |
|
O |
N |
|
Ph |
H |
OH |
N |
Ph |
|
|
|
|||||
|
(41) |
|
|
|
|
(42) |
|
Cy2 P
PPh2
N
CONHMe
(P29)
O |
N |
O |
N |
|
H |
|
H |
|
O |
|
HO H |
|
|
|
|
|
(43) |
|
(44) |
|
O |
HO |
H |
|
NR1R2 |
|
NR1R2 |
|
(45) |
|
(46) |
the amino alcohol 42 in ca 90% e.e. using a complex of P29 with rhodium204a . Since the enantiomeric ligand is available, either product diastereisomer can be prepared. The same ligand has been used, in a complex with rhodium, to mediate the reduction of 43 to (S)-propronolol 44, in this case in 90.8% e.e.204b and in the reduction of the ˇ-amino ketone 45 to R-fluoxetine 46 in 91% e.e.204c.

15. Hydrogenation of compounds containing CDC, CDO and CDN bonds 813
Notwithstanding the excellent results described above, the ligand/metal combination which has without a doubt made the biggest impression on this area is that of ruthenium and BINAP205 207. This combination will feature throughout the rest of this section of the review. As was the case for CDC reductions, either neutral (e.g. [Ru(BINAP)(OAc)2]) or cationic complexes (e.g. [Ru(BINAP)Cl(arene)]X)205 may be employed in asymmetric carbonyl reductions. Complexes may also be formed in situ by a ligand exchange between a complex such as [RuCl2(benzene)2] and BINAP206, thus avoiding the need to prepare a sensitive organometallic complex prior to the reaction. Very high enantioselectivities are achieved using Ru/BINAP combinations for the reductions of unprotected ˛-amino ketones205 although protection of the amino group electron-withdrawing functions can make the substrate less compatible; reduction of 47 to 48 using the cationic Ru/BINAP combination gave a product of only 81% e.e.207. Following the reaction the phthalimide group was removed to give a primary amine. In one of a number of related reports the use of a rhodium complex of a biaryl diphosphine ligand, closely related to BINAP, was shown to be capable of the reduction of the hydrochloride salt of ˛-amino acetophenone in 93% e.e.133c.
O O |
O HO H |
CO2 M e |
CO2 M e |
N |
N |
O |
O |
(47) |
(48) |
Several other classes of substituted ketones are suitable for reduction by Ru/BINAP complexes208. Scheme 39 and Table 4 summarize the range which is applicable. The sense of induction follows simply from the position of the available co-ordinating group in the substrate. In general, high ratios of substrate to catalyst may be employed and the enantiomeric inductions are consistently high. Diketones are reduced in correspondingly high e.e.s208. Several examples have been reported of the reductions of ˛,˛0 -dihydroxy ketones. A steric difference between the groups on the oxygen atoms is sufficient to produce an enantiodifferentiation in the reduction of 49 to give 50 in up to 96% e.e.209. The
|
OH |
|
|
O |
|
|
OH |
|
|
i |
|
ii |
|
||
|
X |
|
|
X |
|
|
X |
R |
( )n |
|
R |
( )n |
|
R |
( )n |
X = NR2 , CO2 R, Br, OH, etc., n = 2, |
|
|
|
|
|||
Reagents: (i) [Ru(R-BINAP)OAc)2 ], H2 , (ii) [Ru(R-BINAP)(OAc)2 ], H2 |
|
||||||
|
|
|
SCHEME 39 |
|
|
|
|
|
O |
|
|
|
H OH |
|
|
|
RO |
OCPh3 |
RO |
|
|
OCPh3 |
|
|
(49) |
|
|
|
(50) |
|

814 |
|
|
Martin Wills |
|
|
|
|
TABLE 4. Reductions of ketones of ruthenium/BINAP catalysts |
|
|
|
||||
|
|
|
|
|
|
|
|
|
Substrate |
R/S-BINAP |
Product |
S/C |
Yield (%) |
e.e (%) |
|
|
|
|
|
|
|
|
|
O |
|
S |
OH |
|
780 |
72 |
96 |
|
NMe2 |
|
NMe2 |
||||
|
|
|
|
|
|
||
|
O |
|
OH |
|
|
|
|
|
NMe2 |
S |
|
NMe2 |
390 |
83 |
95 |
O |
|
R |
OH |
|
230 |
100 |
92 |
|
OH |
|
OH |
||||
|
|
|
|
|
|
||
O |
|
R |
OH |
|
780 |
97 |
83 |
|
|
|
|
||||
|
CO2 E t |
|
|
CO2 E t |
|
|
|
O |
OH |
R |
OH |
OH |
900 |
100 |
98 |
|
|
|
|
||||
O |
|
R |
OH |
|
1260 |
100 |
>99 |
|
CO2 E t |
|
|
||||
|
|
|
CO2 E t |
|
|
|
|
O |
|
S |
OH |
|
680 |
100 |
96 |
|
CONMe2 |
|
CONMe2 |
||||
|
|
|
|
|
|
||
O |
CO2 H |
|
OH |
CO2 H |
|
|
|
|
|
R |
|
|
220 |
100 |
92 |
O |
Br |
|
OH |
Br |
|
|
|
|
|
R |
|
|
1100 |
97 |
92 |
O |
|
|
OH |
|
|
|
|
|
|
S |
|
|
680 |
100 |
100 |
|
O |
|
|
OH |
|
|
|
O |
O |
R |
OH |
OH |
2000 |
100 |
100 |
|
|
|
|
||||
|
|
|
|
|
|
|
|
presence of a trityl group as one of the protecting groups is essential for high inductions, however in the case where one hydroxyl is unprotected, the scope for substitution at the other position is rather broader (Scheme 40)210.
˛-Keto esters and amides are excellent hydrogenation substrates. As early as 1978 the phosphine BPPM (P7) was used in a complex with rhodium for the reductions of ketopantolactone (Scheme 41) in high e.e. (87%)211. The same complex was used

15. Hydrogenation of compounds containing CDC, CDO and CDN bonds 815
O |
|
O |
OH |
F |
O |
86% i |
|
O |
|
O |
OH |
H |
OH |
F
95% e.e
Reagents:(i) [Ru2 Cl4 (S-BINAP)(NEt3 )]. H2 , MeOH
SCHEME 40
|
i |
BPPM (P7) 87% e.e. |
O |
100% |
O (P30) 92% e.e. |
O |
|
HO * |
O |
|
O |
Reagents:(i) [Rh(BPPM-P7)] or [Rh(P30)], H2 , 30 °C, 48 h, benzene
SCHEME 41
earlier for the reductions of ˛-keto esters212. A large number of ligands have since been screened, although perhaps the most interesting observation is the superiority in many cases of dialkylphosphine ligands over diphenylphosphines, which are otherwise commonly used213,192. An example is the use of BCPM, P30, a derivative of BPPM, in the reduction shown in Scheme 41, the e.e. of which is increased slightly, to 92%214. Using BICHEP (P23) and derivatives as ligands, cationic ruthenium complexes proved to be somewhat superior to the rhodium complexes215,101a. Again, however, the best ligands proved to be those containing fully hydrogenated rings on the phosphorus atom, such as P31 (Scheme 42).
A series of reports have appeared describing the use of phosphines derived from amino acids and amides in combination with rhodium or ruthenium. Again, the best ligands proved to be those containing hydrogenated rings on the phosphines, for example P32216 and P33217. Of these ligands, the latter appear to give slightly better results up to 98.7% e.e. for the ketopantolactone reduction shown in Scheme 41 and 87% e.e. for reductions of ˛-keto amides. Silica-supported variants have also been reported216d.

816 |
Martin Wills |
+
Cy2 P
PPh2 |
PCy2 |
− |
|
Ru(arene)Cl |
|||
N |
Cl |
||
P |
|
||
CO2 But |
Cy2 |
|
|
|
|
(P30)-BCPM |
|
(P31) |
|
|
|||
N |
|
OPCy2 |
O |
N |
OPCy2 |
||
|
|||||||
|
|
|
|||||
PCy2 |
|
|
|
PCy2 |
|||
|
|
|
|
|
|
||
(P32) |
|
|
|
|
|
(P33) |
|
O |
|
|
|
|
|
O |
|
|
|
|
i |
|
|
|
> 99% e.e. |
X |
|
|
X = OMe, NHBn, NHBut |
|
|
* |
X |
|
|
|
|
|
|
||
O |
|
|
|
|
|
OH |
|
Reagents:(i) P31, 5 atm H2
SCHEME 42
An interesting example of 1,2-diketone reduction using methods other than metal/phosphine catalysts is the use of a cobalt complex, in combination with quinine, for the reduction of benzil218. In this example the product was formed in 78% e.e. (Scheme 43).
O |
|
|
O |
|
Ph |
i |
Ph |
|
|
Ph |
|
|
||
|
Ph |
78% e.e. |
||
O |
|
|
OH |
|
|
|
|
||
|
|
|
|
Reagents:(i) Co(dimethylglyoxime)2 , quinine, benzylamine, −10° C
SCHEME 43

15. Hydrogenation of compounds containing CDC, CDO and CDN bonds 817
Ruthenium complexes of chiral diphosphines have proved to be some of the best reagents for the reduction of ˇ-keto esters (Scheme 44). Both neutral and cationic complexes may be used. The sense of the asymmetric induction follows that obtained in the reduction of other substituted ketones assuming co-ordination of the metal by both ketone
and ester groups. Since the initial reports of this and related reactions in 1987/8208 the area has grown at a dramatic rate and reductions of this type are now widely used219,126a. Improved methods have been reported for the preparation and use of catalysts by the same team that first reported this methodology205,220, and others221. Many important target molecules have been prepared using this as a key step, including building blocks for Vitamin D3219d and related HMG-CoA reductase inhibitors219e. The synthesis of carnitine, the carrier of acyl groups in fatty acid metabolism, provides an excellent example (Scheme 45)208c. In this reaction it was found that the use of high temperature (100 °C) for a short reaction time (5 minutes!) gave a superior result to the use of lower temperatures and longer reaction times. Only 0.05 mol% of the catalyst was employed in this reaction.
O |
O |
|
|
|
OH |
O |
|
|
|
|
95−100% |
98−100% e.e. |
|||
|
|
|
|
|
|
|
|
R |
OEt |
|
|
|
|
||
|
|
|
R |
OEt |
|||
Reagents:(i) [Ru/BINAP(P5)], |
H2 , 100 atm |
|
|||||
|
|
|
|
SCHEME 44 |
|
||
O |
O |
|
|
|
OH |
O |
|
Cl |
|
|
95% |
|
Cl |
97% e.e. |
|
|
|
|
|
|
|||
|
OEt |
|
|
|
|
OEt |
Reagents:(i) 0.05 mol% [Ru (S-BINAP (P5) (OAc)2 ],01atm H2 , 100 ° C, 5 min
SCHEME 45
Some interesting experimental observations have been made. For example, the addition of 0.5 mol% triethylamine stops the hydrogenation (using 0.02 0.05% catalyst) and the addition of 1% HCl restarts the process221c. The use of hydrogen pressures of around 50 psi in a Parr shaker apparatus appears to be a satisfactory experimentally suitable procedure for most applications221a c. Ruthenium allyl complexes containing BINAP have been reported to be suitable for atmospheric pressure reductions221e.
The reductions of ˇ,υ-diketoesters 51 with ruthenium/BINAP catalysts result in formation of the trans-diols 52 in high selectivity and e.e. Experiments have revealed that it is likely that the reduction takes place via the coordination of both ketones to the metal the selectivity matches that obtained in the reduction of ˇ-diketones222. Biaryl diphosphines closely related in structure to BINAP have also given excellent results223. Other excellent
O |
O |
O |
|
OH |
OH |
O |
R |
|
|
OMe |
R |
|
OMe |
|
(51) |
|
|
|
(52) |
|

818 |
Martin Wills |
diphosphines which have recently been applied to this process include Josiphos (P11)89a and the doubly C2 symmetric ligand P14 (BPE)224. In the latter case, using the isopropyl substituted derivative with ruthenium(II), a large number of reductions were achieved in over 98% e.e. and in some cases up to 99.4% e.e.224.
An exciting variation on the ˇ-diketone reduction process is the dynamic kinetic resolution of ˛-substituted substrates (Scheme 46). Since the racemization rate considerably outpaces that of the reduction, the result is the formation of one predominant product out of four possible products. Cyclic or acyclic substrates may be employed. In the acyclic case the syn product predominates. This reaction has been studied in some depth by Noyori and coworkers225, who have also published a detailed mathematical treatment of the results226. Although BINAP is by far the most widely used ligand for this process, excellent results have also been obtained using BPE ligand P14224 and modified, partially hydrogenated BINAP derivatives227. Applications of this type of methodology are extensive, being an excellent method of introducing two new valuable chiral centres in one step. ˛-Amino-ˇ-keto esters can be reduced selectively to the ˛-amino-ˇ-hydroxy ester products with high selectivity225b,228, and a simple procedure has been developed for the synthesis of L-or D-threonine from 3-oxo-butyrate esters (Scheme 47)228. Reductions of ˛-chloro-ˇ-keto esters229 and ˛-aminomethyl-ˇ-keto esters230 with similar high selectivity have also been reported. The latter reduction leads to the formation of ˇ-lactam precursors
O |
O |
|
|
|
|
|
|
|
OH |
O |
OH |
O |
2 R |
|
OE t |
95 − 100% |
|
|
|
|
|
|
OE t + 2 R |
|
|
|
|
|
|
|
2 R |
|
OE t |
|||||
R1 |
|
|
|
|
|
|
|
|
R1 |
|
|
R1 |
|
|
|
|
|
|
|
|
Major product |
Minor product |
|||
|
|
|
|
|
|
|
|
|
>95% e.e. |
>95% e.e. |
||
Reagents: (i) [Ru/S-BINAP (P5)], H2 , 100 atm |
|
|
|
|||||||||
|
|
|
|
|
|
|
SCHEME 46 |
|
|
|
||
|
O |
|
O |
|
|
|
|
OH |
O |
|
||
|
|
|
|
|
|
|
i, ii, iii |
|
95% e.e. |
|
||
|
|
|
|
|
|
|
|
|
|
|
|
|
|
|
|
OEt |
|
|
|
|
|
|
OEt L-threonine |
||
|
|
|
|
|
|
|
|
|
|
NH2 |
|
|
|
Reagents:(i) HNO2 , Pd/C, H2 , (ii) [Ru((+)-BINAP (P5), X2 ], H2 , (iii) hydrolysis |
|
||||||||||
|
|
|
|
|
|
|
SCHEME 47 |
|
|
|
||
O |
|
O |
|
|
|
|
|
OH |
O |
|
||
|
|
|
|
|
i, ii, iii |
|
|
98% d.e. |
||||
|
|
OMe |
|
|
OMe |
99% e.e. |
||||||
|
|
|
|
|
|
|
|
|
||||
|
|
NHCOPh |
|
|
|
|
|
|
NHCOPh |
|
Reagents:(i) 0.1 mol% [Ru(BINAP P 5 ] (arene)X]X, CH2 Cl2 , MeOH
SCHEME 48
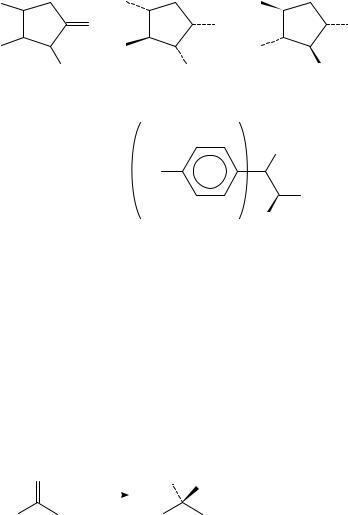
15. Hydrogenation of compounds containing CDC, CDO and CDN bonds 819
in up to 98% d.e. and 99% e.e. (Scheme 48). Highly active allylically substituted ruthenium complexes of BINAP have been used in this process to good effect231. In the reduction of racemic trans-53, each enantiomer was reduced to a different major diatereoisomer, 54 and 55 respectively, thereby allowing a method for the separation of the products232. Asymmetric dynamic hydrogenations of ˛-amino and ˛-bromo-ˇ-keto phosphates have also been reported, the selectivities of which are at least as good as the carboxylic ester analogues233.
Ar |
Ar |
Ar |
|
|
O |
OH |
OH |
Ar |
Ar |
Ar |
|
|
CO2 M e |
CO2 M e |
CO2 M e |
|
trans-(53) |
trans-(54) |
trans-(55) |
|
|
|
NH2 |
|
|
M eO |
|
|
|
|
NH2 |
|
|
2 |
R1 |
|
|
(56) R1 = Me, Pri, Bui |
Asymmetric hydrogenations of simple ketones lack a suitable proximal co-ordinating group, however some dramatic recent developments have had a major impact on the field. Most significantly, Noyori has reported that the combination of a ruthenium complex with a suitable chiral diamine such as 56 results in formation of a complex which is capable of the reduction of simple ketones in e.e.s of up to 95% (Scheme 49)234. Remarkably, ˇ-keto esters are inert under these conditions. The asymmetric reduction of deuterated aldehydes with chiral ruthenium/BINAP complexes has also been reported (up to 89% e.e.)235. The combination of iridium(I) with BINAP, together with the addition of an essential monophosphine such as 57, also results in the formation of an excellent carbonyl
reduction catalyst236. Aromatic/aliphatic ketones may be reduced in e.e.s of up to 84%236a using this combination, and cyclic ketones in up to 95% e.e. (Scheme 50)236b. The use of both phosphines is essential for the induction of high e.e.s, and it has been speculated that the monophosphine modifies the catalyst in a subtle manner by filling an otherwise vacant
|
O |
|
H |
|
R |
e.e. |
R1 in 56 |
|
|
|
OH |
Me |
87 (R) |
i |
|||
|
|
i |
|
|||||
|
|
|
|
|||||
|
|
|
|
|
Bu |
|||
|
99% |
|
|
|
Bun |
90 (R) |
Me |
|
Ph |
R |
Ph |
|
R |
Pri |
95 (R) |
Pri |
|
|
|
|
|
Reagents;(i) [Ru(S-BINAP)(P5)(56)], H2 , KOH
SCHEME 49

820 |
Martin Wills |
coordination site. Iridium hydrogenation complexes are notably selective for the reduction
PhnP |
3-n |
|
Me2 N |
|
(57) |
O |
OH |
|
|
i |
n = 1,2 |
|
up to 95% e.e. |
( )n |
( )n |
Reagents:(i) [Ir(BINAP)(COD)]BF4 , E61, 50 atm H2 , THF/MeOH
SCHEME 50
of the carbonyl groups of enones. Reports have appeared on the application of the above approach236c and a closely related system237, to the synthesis of allylic alcohols, however the e.e.s do not yet match those of other ketones.
Another recent development which has implications for the asymmetric reductions of simple ketones is asymmetric transfer hydrogenation238 240. Some very recent studies have led to the development of several practical systems for achieving highly selective reductions in this manner, which benefit from the lack of a need for high-pressure hydrogenation equipment. Two examples serve to illustrate this exciting new area. In the first (Scheme 51) the ruthenium catalyst 58 is employed239, and in the second (Scheme 52), use is made of the monoprotected diamine 59, again in combination with a ruthenium(II) source238. The latter example is reported to work simply by mixing the reagents in an open vessel and in both cases the hydrogen is supplied by an alcohol, 2-hydroxypropane. Both reports have come from the Noyori group. The use of a samarium complex with a chiral, C2 symmetric amino diol in a related type of transformation has been reported to give excellent enantioselectivities in the reduction of aromatic ketones240e.
|
|
Ph |
NHSO2 Tol-p |
NH |
Cl |
NH |
.[RuCl2 (mesitylene)]2 |
|
Ru |
Ph |
NH2 |
|
|
||
P |
Cl |
P |
|
Ph2 |
|
Ph2 |
|
(58) |
(59)(SS)-TSDPEN |