

Supplement A3: The Chemistry of Double-Bonded Functional Groups. Edited by Saul Patai Copyright 1997 John Wiley & Sons, Ltd.
ISBN: 0-471-95956-1
CHAPTER 14
Some synthetic uses of double-bonded functional groups
JEFF HOYLE
Chemistry and Soil Science Department, Nova Scotia Agricultural College, Truro, NS, Canada, B2N 5E3
Fax: 902-893-1404; e-mail: jeff.hoyle@nsac.ns.ca
I. INTRODUCTION . . . . . . . . . . . . . . . . . . . . . . . . . . . . . . . . . . . . . |
706 |
|
II. OXIDATION . . . . . . . . . . . . . . . . . . . . . . . . . . . . . . . . . . . . . . . . . |
706 |
|
A. Epoxidation of Alkenes . . . . . . . . . . . . . . . . . . . . . . . . . . . . . . . . |
707 |
|
B. Dihydroxylation of Alkenes . . . . . . . . . . . . . . . . . . . . . . . . . . . . . |
711 |
|
C. Baeyer Villiger Oxidations . . . . . . . . . . . . . . . . . . . . . . . . . . . . . |
713 |
|
D. Reductive-Oxidation Processes . . . . . . . . . . . . . . . . . . . . . . . . . . . |
714 |
|
E. Oxidative Cleavage . . . . . . . . . . . . . . . . . . . . . . . . . . . . . . . . . . |
715 |
|
F. Other Oxidations . . . . . . . . . . . . . . . . . . . . . . . . . . . . . . . . . . . . |
716 |
|
1. |
Formation of ketones and related functionalities . . . . . . . . . . . . . |
716 |
2. |
Formation of acids and their derivatives . . . . . . . . . . . . . . . . . . . |
718 |
3. |
Others . . . . . . . . . . . . . . . . . . . . . . . . . . . . . . . . . . . . . . . . . |
719 |
III. REDUCTION . . . . . . . . . . . . . . . . . . . . . . . . . . . . . . . . . . . . . . . . |
720 |
|
A. Reduction of Ketones and Aldehydes to Alcohols . . . . . . . . . . . . . . |
720 |
|
1. |
Using hydride transfer and related reagents . . . . . . . . . . . . . . . . . |
720 |
2. |
Using boron-containing reagents . . . . . . . . . . . . . . . . . . . . . . . . |
723 |
3. |
Using baker’s yeast . . . . . . . . . . . . . . . . . . . . . . . . . . . . . . . . |
725 |
4. |
Other methods . . . . . . . . . . . . . . . . . . . . . . . . . . . . . . . . . . . |
726 |
B. Reduction of CDN Containing Compounds . . . . . . . . . . . . . . . . . . . |
727 |
|
C. Catalytic Hydrogenation of Alkenes . . . . . . . . . . . . . . . . . . . . . . . |
729 |
|
D. Other Reductions . . . . . . . . . . . . . . . . . . . . . . . . . . . . . . . . . . . . |
730 |
|
IV. C C BOND-FORMING REACTIONS . . . . . . . . . . . . . . . . . . . . . . . |
732 |
|
A. Carbonylation and Relation Reactions . . . . . . . . . . . . . . . . . . . . . . |
732 |
|
B. Vinylations . . . . . . . . . . . . . . . . . . . . . . . . . . . . . . . . . . . . . . . . |
734 |
|
C. Reactions with Organometallic Reagents . . . . . . . . . . . . . . . . . . . . |
735 |
|
1. |
With alkenes . . . . . . . . . . . . . . . . . . . . . . . . . . . . . . . . . . . . . |
735 |
2. |
With carbonyl Compounds . . . . . . . . . . . . . . . . . . . . . . . . . . . |
737 |
3. |
With imines . . . . . . . . . . . . . . . . . . . . . . . . . . . . . . . . . . . . . |
739 |
705

706 |
|
Jeff Hoyle |
|
D. |
1,2-Additions to Carbonyl Compounds . . . . . . . . . . . . . . . . . . . . . . |
739 |
|
E. Reductive Coupling of Carbonyl and Related Compounds . . . . . . . . . |
742 |
||
F. Coupling of Alkenes and Carbonyl Compounds . . . . . . . . . . . . . . . . |
744 |
||
G. Wittig-type Reactions . . . . . . . . . . . . . . . . . . . . . . . . . . . . . . . . . |
745 |
||
H. Others . . . . . . . . . . . . . . . . . . . . . . . . . . . . . . . . . . . . . . . . . . . |
746 |
||
V. PREPARATION OF HETEROCYCLES . . . . . . . . . . . . . . . . . . . . . . . |
747 |
||
A. Heterocycles with a Single Heteroatom . . . . . . . . . . . . . . . . . . . . . |
748 |
||
|
1. |
Threeand four-membered rings . . . . . . . . . . . . . . . . . . . . . . . . |
748 |
|
2. |
Fiveand six-membered rings . . . . . . . . . . . . . . . . . . . . . . . . . |
752 |
|
3. |
Larger rings containing a single heteroatom . . . . . . . . . . . . . . . . |
760 |
B. Heterocycles with Multiple Heteroatoms . . . . . . . . . . . . . . . . . . . . |
762 |
||
VI. ACKNOWLEDGMENTS . . . . . . . . . . . . . . . . . . . . . . . . . . . . . . . . |
764 |
||
VII. REFERENCES . . . . . . . . . . . . . . . . . . . . . . . . . . . . . . . . . . . . . . . |
765 |
||
|
|
|
|
I. INTRODUCTION
Modern organic synthesis requires that polyfunctional molecules be transformable into synthetic targets by simple and mild reactions with total stereocontrol. Although this ideal is not always attained, it is a goal that is strived for by most of the world’s major synthetic organic chemistry groups. Synthetic organic chemists are challenged by this goal which stimulates the development of new reaction strategies using old procedures in novel ways and the development of new reaction pathways.
A cursory glance at a recent volume of any journal or book which covers organic synthesis will convince the reader of the central importance of double-bonded functional groups as sources of starting materials, as key intermediates and as synthetic target molecules. In particular, the reader is referred to the excellent Art in Organic Synthesis1 which chronicles key syntheses that have been successfully completed over the last several decades.
Functional group transformations from alkenes, imines, carbonyl compounds and other double-bonded functional groups may be easily performed in high yield. In this review, transformations as a result of oxidation (Section II) and reduction (Section III) are covered. The reader is urged to consult the excellent Comprehensive Organic Transformations by Larock2 for in-depth listings of functional group interconversions for double-bonded functional groups. The major synthetic importance of these functionalities arises from the ease by which compounds that contain these groups may be used in C C bond-forming reactions (Section IV) and in the preparation of heterocyclic compounds (Section V).
In this review, the above-mentioned useful synthetic processes will be discussed in detail with examples mainly taken from the chemical literature since 1990.
The intent of this review is not to cover all aspects of the use of double-bonded functional groups in synthesis but rather to cover recent developments that are hopefully of interest to the synthetic community at large. Other chapters in the present volume cover some other reactions of double-bonded functional groups.
This chapter does not cover Diels Alder and other pericyclic reactions, in any detail, since these have been the subject of several recent reviews3 8. Nor is the chemistry of double-bonded functional groups involving allenes or carbenes covered in any great detail.
The present review covers the chemical literature up to the beginning of 1995.
II. OXIDATION
Oxidation of double bond-containing functional groups is a key method in synthesis which is used to form other functional moieties, especially in a stereocontrolled fashion. In this section the epoxidation and dihydroxylation of alkenes are covered in some detail. These are particularly common methods used for stereocontrolled elaboration of organic molecules.

14. Some synthetic uses of double-bonded functional groups |
707 |
A. Epoxidation of Alkenes
Epoxides are useful synthetic intermediates or targets which can be readily prepared by many routes from the corresponding alkenes. Traditionally, this process was performed using hydrogen peroxide and organophosphorus electrophiles9 12 or peroxy acids catalyzed with transition metal salts13, but safety concerns with these methodologies have also caused workers to develop many other new routes. However, it is noteworthy that the commercial synthesis of propylene oxide (more than 1 million tonnes annually worldwide) occurs via hydroperoxide oxidation14.
Notwithstanding this safety aspect, mCPBA continues to be used as an epoxidizing agent. In one such reaction, a diepoxidation (equation 1) was brought about as a key step in the synthesis of the spirocyclic core of aranorosin15, which is a novel antibiotic. A radical inhibitor was added in order to achieve an acceptable yield of 46%.
BnO |
OH |
BnO |
OH |
|
mCPBA |
O |
O |
|
CCl4 |
|
(1) |
|
O |
|
O |
NR2 |
O |
NR2 |
O |
|
|
tert-Butyl hydroperoxide acts as a very useful oxidant in the presence of vanadium pentoxide and titanium complexes as catalysts16,17. This method suffers from safety drawbacks due to the toxicity of vanadium oxide dust. Use of a titanium complex gives somewhat improved yields and significantly reduces the safety problems18. This methodology has been used in the asymmetric synthesis of (S)-fenfluramine, an important anorectic agent19.
Oxidation by a hydroperoxide (generated in situ from oxygen and a cobalt salt) enables unfunctional alkenes to be epoxidized in reasonable yield20. A very attractive and much improved methodology for forming epoxides is by use of an oxidant which is regenerated in situ, so as to reduce the amount of oxidant required. This has been performed using oxygen with a sacraficial aldehyde catalyzed by Ni(dmp)221 23, metalloporphyrins24, or ‘clayniac’ (which is a clay supported nickel complex)25,26. These reactions occur in high yields and in a stereocontrolled manner (in some cases) for a wide range of alkenes. Metal alkylperoxides also give a highly diastereoselective epoxidation of N- tosylvinylsulfoximines, under low temperature conditions (equation 2)27,28. The yields are very high and the degree of selectivity depends upon the metal cation being used. Vinyl sulfones also undergo stereocontrolled epoxidation with these reagents.
|
O |
|
Ph |
|
O |
|
Ph |
O |
|
Ph |
|
TolSO2 N |
|
S |
|
RO2 −M+ |
TolSO2 N |
|
S |
O |
+ TolSO2 N |
S |
O |
|
|
||||||||||
|
|
|
|
|
|
(2)
Unfunctionalized alkenes have been epoxidized in 40 80% yield by an aerobic process that is catalyzed by salen-manganese(III) complexes at room temperature (equation 3).
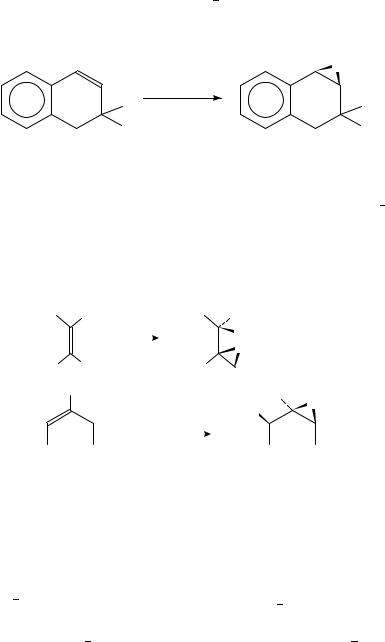
708 |
Jeff Hoyle |
The reaction occurs with some enantioselectivity and requires the presence of pivaldehyde (which is also oxidized)29,30. The reaction occurs for many other alkenes using transition metals coordinated to 1,3-diketone type ligands31 34. Use of a cobalt(II) complex and aldoacetal in place of the Mn(III) compound and pivaldehyde gives a novel method for the synthesis of acid-sensitive epoxides35.
|
O |
O2 |
(3) |
|
|
Mn(III) cat |
|
Oxygen may also be used as the oxidant for epoxidation. For example, a selective epoxidation, in the absence of metal ions, has been performed in excellent yield by treatment of alkenes with oxygen in the presence of a large excess of benzaldehyde36. Photooxygenation of alkenes, in the presence of transition metal catalysts, is a very general and synthetically useful reaction which leads to hydroxy-epoxidation (equation 4)37 39.
The reaction exhibits excellent stereocontrol in most instances, with the major exception being simple alkenes. The reaction has been improved so that even these latter substrates give the desired products, by initial preparation of vinylsilanes (equation 5)40. The reaction occurs in good yields and the Me3Si group can be easily removed at the end of the synthetic sequence.
R1 |
R2 |
R1 |
R2 |
|
|
|
|
1O2 |
|
OH |
(4) |
|
|
cat |
|
O |
|
|
|
|
|
||
R3 |
Me |
R3 |
|
|
|
|
SiMe3 |
|
Me3 Si |
O |
|
|
|
|
|
||
|
|
|
|
HO |
|
|
1.1O2 |
R |
R |
2. NaBH4 |
|
|
3. t-BuOOH / cat |
(5)
R R
Sulfonic peracids, synthesized in situ from arylsulfonyl imidazolides and hydrogen peroxide, are also efficient reagents for the epoxidation of a wide range of alkenes41. Substituents in the aryl group affect the selectivity of this reagents and this reaction occurs in an alkaline medium.
A very useful asymmetric epoxidation reaction of cis-alkenes, in the presence of trans- alkenes, has been developed using sodium hypochlorite (Chlorox bleach) and catalyzed by a complex manganese(III) salt (equation 6)42,43. PhIO may also be used as the oxidant44. This type of selectivity for epoxidation of cis-alkenes has been seen in other epoxidation reactions45 47. The enantiomeric excess of the product is 80 90% and overall yields are reasonable. A similar epoxidation process has been used with a wide range of other alkenes, especially those which lack polar directing groups, to generate optically active synthetic intermediates48 55. The reaction is also catalyzed by porphyrins56 59. Other asymmetric oxidations of alkenes have been performed by Jacobsen and coworkers using
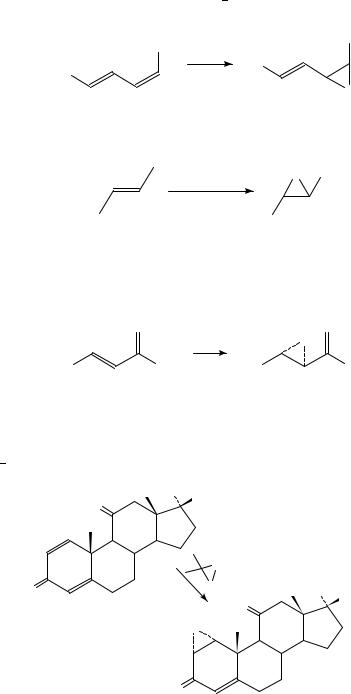
14. Some synthetic uses of double-bonded functional groups |
709 |
hypochlorite and iodosoaromatic compounds60 62.
|
R1 |
|
R1 |
|
|
|
|
R |
NaOCl |
R |
(6) |
cat |
|
||
|
|
O
Alkenes may be epoxidized in an efficient manner by the use of tetrabutylammonium periodate, catalyzed by a manganese porphyrin63 in the presence of imidazole (equation 7)64. Sodium periodate may also be used as oxidant in a similar system65.
Bu4 NIO4
Mn(III) porphyrin imidazole
O
(7)
In the synthesis of optically active aryl glycidic esters, Julia66 type epoxidation (triphasic system of NaOH/aqueous hydrogen peroxide, hexane and a polyamino acid) of an unsaturated ketone gives excellent yields of the required epoxide67. A potent and selective leukotriene antagonist (SK&F 104353) has been synthesized by a route where one of the key steps is this type of epoxidation (equation 8)68.
|
O |
|
|
O |
|
|
|
O |
(8) |
|
|
|
|
|
Ar |
Ar1 |
Ar |
|
Ar1 |
Dimethyldioxirane has also been used as the epoxidizing agent in a key step in the synthesis of A-norsteroids69,70. The reaction occurs in dichloromethane-acetone and is highly regioand stereoselective as shown in equation 9. Dioxiranes may also be generated in situ, by reaction of potassium monoperoxysulfate (sold commercially as OXONE) and cyclohexanones. In this case, cyclohexene derivatives may be smoothly epoxidized in 40 100% yields (equation 10)71.
|
HO |
COCH2 OAc |
|
|
|
O |
|
|
|
|
|
|
|
|
|
|
O |
|
(9) |
|
|
|
|
|
O |
|
O |
HO |
COCH2 OAc |
|
|
|||
|
|
O |
|
|
|
|
|
|
|
|
|
O |
|
|
O
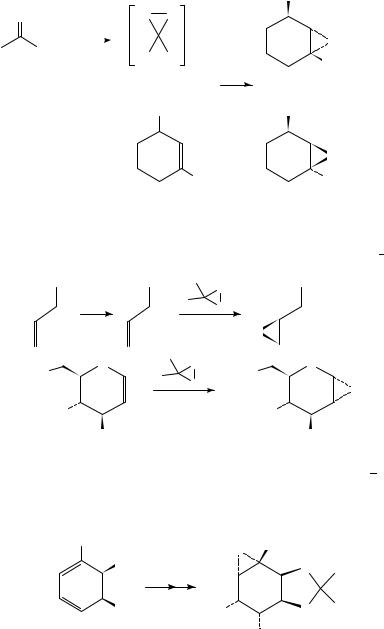
710 |
|
|
|
|
Jeff Hoyle |
|
|
|
|
|
|
R4 |
|
|
O |
|
|
O |
O |
|
|
|
|
|
|
|
|
|
|
|
KHSO4 |
|
|
O |
R1 |
|
R2 |
R2 |
|||
|
|
|||||
|
|
|
|
R1 |
R3 |
|
|
|
|
|
|
|
|
|
|
+ |
+ |
(10) |
||
|
|
|
|
R4 |
R4 |
|
|
|
|
|
|
|
O |
|
|
|
|
|
R3 |
R3 |
Epoxidation of allylic alcohols can be achieved by first protecting the alcohol functional group. The reagent of choice for this process is dimethyldioxirane, which is usually generated in situ (equation 11)72. If the alcohol group is not protected then it is the preferred site of oxidation73. This methodology has been used to prepare the important synthetic intermediates, 1,2-anhydro sugars (glycal epoxides) from glucals (equation 12)74 77.
OH |
OAc |
OAc |
|
|
O |
O |
(11) |
|
O
O |
O |
O |
RO |
|
RO |
O
O
(12)
RO |
RO |
OR |
OR |
An unusual regiocontrolled oxidation (epoxidation and dihydroxylation) of 1-halo-1,3- dienes (equation 13) has been accomplished by use of potassium permanganate MgSO4, after initial treatment with 2,2-dimethoxypropane78. The preferred epoxy diol is formed with the epoxide being adjacent to the chloro substituent. Further elaboration of these molecules yields highly sought after inositols with controlled stereochemistry.
Cl |
|
O |
Cl |
|
|
||
|
|
|
|
OR |
|
|
O |
|
|
|
|
|
|
|
(13) |
OR |
HO |
|
O |
OH
Electrochemical epoxidation of electron-poor alkenes can be accomplished by the use of silver(III) bipyridine-based redox mediators79. The reaction proceeds in aqueous
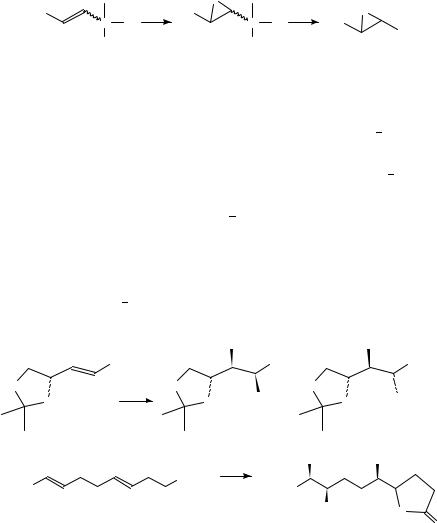
14. Some synthetic uses of double-bonded functional groups |
711 |
acetonitrile at room temperature, in high synthetic yields. Enantioselective epoxidation of unfunctionalized alkenes has recently been reported using hydrogen peroxide with antibody 20B11 as catalyst80. This is the first reported example of an antibody-mediated oxidation at carbon, in vitro.
Finally, even with all the above methods available to the organic chemist, it is still fairly difficult to prepare simple epoxides. One route has been developed recently which uses Sharpless epoxidation81,82 procedures on alkenylsilanols (equation 14)83. This reaction produces an epoxysilanol which is readily converted to the epoxide in good yields by treatment with fluoride ions.
|
|
O |
|
O |
R |
R |
|
|
|
|
Si |
Si |
R |
(14) |
|
OH |
OH |
|
H |
|
|
|
B. Dihydroxylation of Alkenes
Dihydroxylation (especially asymmetric reactions) of alkenes is a very important synthetic tool for the introduction of a new functionality into organic molecules. The reader is referred to recent reviews on the synthetic utility and mechanisms of asymmetric dihydroxylation for useful background material regarding synthetic outcomes84 88.
Catalyzed osmium tetraoxide mediated, asymmetric dihydroxylation (AD) of alkenes has become a very useful means of distereoselective functionalizing alkene-containing molecules, which contain a wide-range of neighboring stereogenic centers89 93. In this reaction the choice of oxidizing agent-coordinating ligands is extremely critical in determining which diastereomers are produced. For example, compound 1 (equation 15) was the major product when phthalazine ligands94 96 were used, whilst compound 2 was the major product when pyrimidine analogues97 were the ligand of choice. The latter ligands are particularly useful in the dihydroxylation of terminal olefins. A key step in the total synthesis of solamin and reticulatacin (naturally occurring acetogenins) was performed using this methodology (equation 16)98. A polymer-bound quinine derivative has also been used recently in the enantioselective dihydroxylation of olefins99. However, in some reactions of this type100 107 the diastereoselectivity is not as good as that achieved by titanium-catalyzed epoxidations108.
|
|
HO |
|
|
HO |
|
COOR |
|
COOR |
|
COOR |
O |
|
O |
O |
|
(15) |
O |
OsO4 |
O |
OH + |
O |
OH |
|
|
|
|||
|
|
(1) |
|
|
(2) |
|
|
|
OH |
|
OH |
C12 H2 5 |
|
CO2 Et |
C12 H2 5 |
|
|
|
|
|
|
OH O
O
(16)

712 |
Jeff Hoyle |
Enantioselective dihydroxylation of trans-alkenes has been performed, with a high degree of selectivity, by catalysis of the oxidation process by chiral diamines109 113. In this way an anthracycline has been prepared via a short-step process, the key step being a catalyzed dihydroxylation (equation 17) which occurred in 85% enantiomeric excess. Asymmetric dihydroxylation of sulfur(II)-containing olefins has been performed successfully, with excellent chemoselectivity114. This is somewhat surprising, but is due to the selectivity of the AD reagents and to the relatively slow oxidation reaction which OsO4 and sulfur(II) atoms undergo.
OMe |
OMe |
OH |
|
CO2 Me |
|
CO2 Me |
|
1. OsO4 , cat |
OH |
(17) |
|
|
− |
||
2. HSO3 |
|
|
|
OMe |
OMe |
|
|
In the synthesis of polyhydroxylated polyenes, osmium tetroxide induced dihydroxylation of an alkene adjacent to a coordinated iron atom has been used as the key synthetic step115. The reaction has been shown to be highly diastereoselective for E-alkenes and diastereospecific for Z-alkenes. With both types of alkene, the reaction occurs anti to the coordinated iron group (equation 18).
|
|
|
|
OH |
RO (CO)3 Fe |
|
RO |
(CO)3 Fe |
OH (18) |
|
OsO4 , py |
|
||
|
|
|
||
MeO2 C |
|
|
MeO2 C |
|
Primary allylic halides may be efficiently asymmetrically dihydroxylated in 40 98% ee. The products may be readily converted into epoxy alcohols in high yields, by treatment with NaOH in THF (equation 19)116.
|
|
OH |
|
|
X |
X |
|
O |
|
OH |
OH |
(19) |
||
|
|
+ |
|
|
|
|
|
|
The key step in the synthesis of novel azole antifungal agents has been performed by asymmetric dihydroxylation117 using a modified AD-mix118 120 (equation 20). The reaction occurs in excellent yields at room temperature in t-BuOH water as solvent.
|
|
|
HO |
|
F |
N |
F |
OH |
N |
|
|
N |
|
N |
|
N |
|
|
N |
|
|
CH3 SO2 NH2 |
(R) |
(20) |
|
|
|
|
|
|
|
t-BuOH, H2 O, A D mix |
|
|
F F

14. Some synthetic uses of double-bonded functional groups |
713 |
Spiro acetals can also be stereoselectively hydroxylated, in high yield, with osmium tetraoxide and a catalyst. The resultant diol is formed by syn-hydroxylation from the least hindered side of the alkene moiety (equation 21)121.
|
OH |
|
|
|
OH |
O |
O |
(21) |
O |
O |
|
A vincinal amino alcohol grouping is present in a fair number of natural products which possess useful biological activity, such as antibiotics122. Such a functionality has been produced from alkenes via osmium-mediated aminohydroxylation (equation 22)123. The reaction proceeds in 40 97% yield and is enantioselective if chiral osmium-Cinchona alkaloid complexes are used to mediate the reaction.
OsO3 N |
HO |
|
|
|
(22) |
chiral |
|
catalyst |
NH |
In a rather obscure, but synthetically very useful reaction, the biooxidative hydroxylation of chloro-benzenes (equation 23) has been used as a key step in the synthesis of inositols124. Cyclohexadiene cis-diols are produced and similarly functionalized compounds may also be obtained from quinolines and isoquinolines125. These reactions have been exploited for the synthesis of a number of natural products126,127.
|
|
|
OH |
|
Pseudom onas |
|
(23) |
|
putida |
|
|
|
|
|
|
Cl |
|
Cl |
OH |
|
|
C. Baeyer Villiger Oxidations
The regioselectivity of the migration in Baeyer Villiger oxidation of ketones is very dependent upon substituents attached to carbon atoms adjacent to the ketone. In a study128 of the MCPBA oxidation of polyhydroxycyclohexanone derivatives, the regioselectivity of the reaction has been carefully identified. This should prove useful to synthetic chemists when planning to use this type of reaction, since cyclitols are important synthons in natural product synthesis.
Baeyer Villiger oxidation of ketones to esters or lactones is a very useful synthetic technique which has been used for many years129,130. Variations of this reaction have recently been performed with molecular oxygen using Fe2O3131, nickel(II) complexes132, and benzaldehyde with a nickel133 or ruthenium134 complex as catalysts. The last of these reactions (equation 24) is noteworthy since it occurs in the absence of ionic metal

714 |
Jeff Hoyle |
catalysts, thus giving a new, milder alternate methodology for this established oxidation process.
(CH2 )n |
|
|
(CH2 )n |
O |
|
O |
O2 |
(24) |
|||
|
|||||
|
PhCHO |
|
|||
|
|
|
O |
||
|
|
RuO2 |
|
||
|
|
|
|
Baeyer Villiger oxidation has been used to selectively oxidize one of two methyl ketones (to esters) in the final step of a stereoselective synthesis of ( )-acetomycin, an antibiotic with potential anti-leukemia activity (equation 25)135. This reaction was accomplished using MCPBA as oxidant, with an excess of sodium bicarbonate and 5- tert-butyl-4-hydroxy-2-methyl phenyl sulfide as a radical inhibitor.
O
O
(25)
O O
O
O
O
O O
D. Reductive-Oxidation Processes
This section covers the hydroboration oxidation of alkenes to give alcohols. The author chooses to include this under oxidation since an oxygen atom is introduced into the molecule. This reaction can be performed in a stereocontrolled fashion and it is these methods that are highlighted here. In addition, one similar reductive-oxidation reaction is included, since it is an extremely facile route to benzyl alcohols and ˛- hydroxyalkanoic acids.
Hydroboration of a wide range of alkenes using catecholborane (and similar boranes), catalyzed by samarium(III)136,137, palladium138,139, iridium140,141 and other transition metal and lanthanide complexes142 145, gives good to excellent yields of alcohols (equation 26). The process is regioselective and is dependent upon the catalyst used146. However, in the presence of some functional groups there is a significant yield of alkane (via hydrogenation) and aldehyde (after the oxidative work-up step)147. The normal regiochemistry can be inverted if allylic sulfones are used. In such a case, rhodiumcatalyzed hydroboration gives the Markovnikov product (equation 27)148. In the absence of catalyst, a mixture of products is obtained. Similar inversion of selectivity is also obtained by rhodium-catalyzed hydroboration of styrenes149 151.
(26)
HO H
RSO2 |
RSO2 |
(27) |
|
|
OH |