
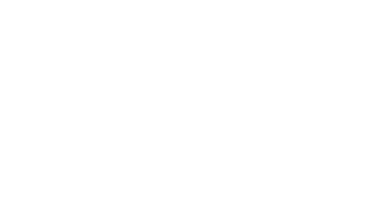
3. Chiroptical properties of amino compounds |
125 |
diastereomers prior to NMR analysis and care has to be taken to insure that neither kinetic resolution nor racemization of the derivatizing agent occurs during derivatization.
Of |
the CDAs, |
the |
most widely used are the |
enantiomers of |
MTPA (83) |
|
which |
have been used |
successfully |
for the estimation of enantiomeric excess of |
|||
chiral |
alcohols and |
amines77. Thus, |
Figure 3 shows |
both the proton |
and fluorine- |
19 NMR spectra of the two diastereomers of N-(4-methyl-2-pentyl)-˛-methoxy-˛- trifluoromethylphenylacetamide, prepared from š -83 and 4-methyl-2-pentylamine [ š - 84]. Figure 3 illustrates the advantage of using the signals from the trifluoromethyl group over those from the protons of the MTPA amide for determination of the enantiomeric composition. The signals for the protons of the isopropyl group, as well as those for the ˛-methoxyl group, occur as two sets of overlapping doublets, one for the R,S/S,R enantiomeric pair and another for the R,R/S,S enantiomeric pair. Any attempt at obtaining the relative proportions of the diastereomers by integration of the overlapping proton signal would lead to very inaccurate results. The 19F NMR spectrum taken at 94.1 MHz is uncomplicated and shows a separation of 10 Hz for the signals for the two diastereomeric pairs, so that the relative areas of these signals easily can be obtained by integration. For use with a chiral amine, the enantiomers of 83 are easily prepared by asymmetric synthesis77 or are available commercially in enantiomerically pure form either as the acid or acid chloride. Because there is no hydrogen atom ˛ to the carboxyl group, racemization during derivatization using an enantiomer of 83 or the respective acid chloride is impossible. Finally, useful NMR configurational correlation schemes have been devised that permit the assignment of the absolute configuration of amines in MPTA derivatives78.
More recently, the chiral phosphonate 85 has been used as a CDA with chiral amines to form diastereomeric phosphonic amides (86)79 which are analyzed by 31P-NMR spectroscopy for the determination of enantiomeric ratios. The reagent is readily prepared from S-2-butanol and phosphorous trichloride, and all ˛-amino acids and amines thus far examined react quantitatively in a few hours at room temperature in aqueous ethanol79.
FIGURE 3. The NMR spectra of the two racemic diastereomers of N-(4-methyl-2-pentyl)-˛-methoxy- ˛-trifluoromethylphenylacetamide prepared from racemic ˛-methoxy-˛-(trifluoromethyl)phenylacetic acid [MTPA, š -83] and racemic 4-methyl-2-pentylamine [ š -84]: (A) 60-MHz proton spectrum in chloroform-d with tetramethylsilane (TMS) as the internal standard; (B) 94.1-MHz fluorine-19 spectrum in chloroform-d with trifluoroacetic acid as the internal standard. Reprinted with permission from Reference 76. Copyright (1969) American Chemical Society

126 |
|
|
Howard E. Smith |
|
|
CH3 CH2 |
H |
CH3 |
CH3 CH2 |
H |
CH3 |
|
C |
|
|
C |
|
|
O |
O |
|
O |
O |
|
|
P |
|
|
P |
|
O |
H |
|
O |
NH R* |
|
C |
|
|
C |
|
CH3 CH2 |
H CH3 |
CH3 CH2 |
H CH3 |
||
|
(85) |
|
|
(86) |
|
Another phosphorus containing CDA (87) has also been described for the estimation of the enantiomeric excess of chiral amines80. The phosphorus CDA 87 is formed quantitatively and instantaneously in situ in an NMR tube by reaction of phosphorus trichloride (PCl3) with the chiral diamine 88. Addition to the NMR tube of the chiral amine (RŁ -NH2) for which the ee is to be determined gives the diastereomeric phosphorous derivatives
CF3 |
CF3 |
|
|
CH3 |
CH3 |
|
|
NH |
N |
|
|
PCl3 |
R* NH2 |
||
|
PCl |
|
|
NH |
N |
|
|
CH3 |
CH3 |
|
|
CF3 |
CF3 |
|
|
(88) |
(87) |
|
|
CF3 |
CF3 |
|
|
CH3 |
CH3 |
|
|
N |
N |
S (Se) |
|
|
S8 |
||
PNHR* |
|
||
P |
|
||
|
or Se8 |
NHR* |
|
N |
N |
||
|
|||
CH3 |
CH3 |
|
|
CF3 |
CF3 |
|
|
(89) |
(90) |
|

3. Chiroptical properties of amino compounds |
127 |
(89), again formed instantaneously and quantitatively80. A first 31P NMR spectrum of the trivalent phosphorus derivative 89 may be recorded and the enantiomeric composition the chiral amine determined, and then the 13C NMR spectrum may also be recorded. In a second step, it is possible to form new derivatives 90 by direct addition to the NMR tube of excess molecular sulphur (S8) or selenium (Se8), the diastereomer derivatives 90 perhaps giving a larger separation of the diastereomeric 31P or 13C NMR signals80.
The enantiomeric excess of a partially racemic chiral amine may also be estimated using an achiral phosphorus coupling reagent, methylphosphonothioic dichloride (91)81. The latter reacts in the presence of triethylamine in deuteriochloroform to afford diastereomeric methylphosphonothionic diamides. When racemic allylglycine methyl ester [ š -92] was used with 91, the 31P NMR spectrum of the reaction product showed three well-separated singlets: one for the racemate š -93 [(R,R)-93 + (S,S)-93] and one for each of the two meso diastereomers [(R,S)-94 and (R,S)-95], with a meso/racemic ratio of 49.51 (Figure 4)81. The ratio of these singlets is directly related to the ee of the ˛-amino acid ester 92. For enantiomerically enriched 92 (ee 96.6%) the two meso signals could just be observed, and integration of the signal gave an ee of 97% for the ˛-amino acid ester 9281. Equally satisfactory results were observed using other ˛-amino acid esters and chiral alkyland ˛- and ˇ-arylalkylamines81.
FIGURE 4. Phosphorus-31 NMR spectrum of a mixture prepared by reaction of racemic allylglycine methyl ester [ š -92] and methylphosphothioic dichloride (91) in deuteriochloroform, showing from left to right, a singlet for a meso compound [(R,S)-94 or (R,S)-95], a singlet for the racemate š -93 and a singlet for the other meso compound [(R,S)-94 or (R,S)-95]. Reprinted with permission from Reference 81. Copyright (1986) American Chemical Society

128 |
|
|
|
|
|
|
|
|
|
|
|
|
|
|
|
Howard E. Smith |
|
|
|
|
|
|
|
|
|
|
|
|
||||||||||||
|
CO2 CH3 |
|
|
|
|
|
|
|
|
|
|
|
|
|
|
|
|
|
|
|
|
|
|
|
|
|
|
|
|
|
|
|
||||||||
|
|
|
|
|
|
|
|
|
|
|
|
|
|
S |
|
|
|
|
|
|
|
|
|
|
|
|
||||||||||||||
CHNH2 |
|
|
|
|
|
|
|
|
|
|
|
|
|
|
|
|
||||||||||||||||||||||||
|
|
|
|
|
|
|
|
|
|
+ |
|
|
|
|
|
|
Et3 N |
|
|
|
|
|
|
|
|
|
|
|
|
|
||||||||||
|
|
|
|
|
|
|
|
|
|
|
|
|
|
|
CDCl3 |
|
|
|
|
|
|
|
|
|
|
|
|
|||||||||||||
CH2 |
|
|
|
|
|
|
|
|
|
|
|
|
|
|
|
|
|
|
|
|
|
|
|
|
|
|||||||||||||||
|
|
|
|
|
|
|
|
|
|
H3 CPCl2 |
|
|
|
|
|
|
|
|
|
|
|
|
||||||||||||||||||
|
|
|
|
|
|
|
|
|
|
|
|
|
|
(91) |
|
|
|
|
|
|
|
|
|
|
|
|
|
|
|
|
|
|
|
|
|
|
|
|
||
CH |
|
|
CH2 |
|
|
|
|
|
|
|
|
|
|
|
|
|
|
|
|
|||||||||||||||||||||
|
|
|
|
|
|
|
|
|
|
|
|
|
|
|
|
|
|
|||||||||||||||||||||||
|
|
|
|
|
|
|
|
|
|
|
|
|
|
|
|
|
|
|
|
|
|
|
|
|
|
|
|
|
|
|
|
|
||||||||
[(±)-92] |
|
|
|
|
|
|
|
|
|
|
|
|
|
|
|
|
|
|
|
|
|
|
|
|
|
|
|
|
|
|
|
|
||||||||
|
|
|
|
CH2 CH |
|
|
|
CH2 |
|
|
|
|
|
CH2 CH |
|
|
|
CH2 |
|
|
CH2 CH |
|
|
|
CH2 |
|||||||||||||||
|
|
|
|
|
|
|
|
|
|
|
|
|
||||||||||||||||||||||||||||
|
|
|
|
|
|
|
|
|
|
|
|
|
||||||||||||||||||||||||||||
|
|
|
|
|
|
|
|
|
|
|
|
|
|
|
|
|
|
|
|
|
|
|
|
|
|
|
|
|
|
|
|
|
|
|
|
|
|
|||
H |
C CO2 CH3 |
|
|
H C CO2 CH3 |
|
H C CO2 CH3 |
||||||||||||||||||||||||||||||||||
|
|
|
|
|
|
|
|
|
|
|
|
|
|
|
|
|
|
|
|
|
|
|
|
|
|
|
|
|
|
|
|
|
|
|
|
|
|
|
|
|
|
|
|
|
|
|
|
|
|
|
|
|
|
|
|
|
|
|
|
|
|
|
|
|
|
|
|
|
|
|
|
|
|
|
|
|
|
||||
|
|
|
|
NH |
|
|
|
|
|
|
NH |
|
NH |
|||||||||||||||||||||||||||
H3 C |
|
|
|
|
|
|
|
|
|
|
+ H3 C |
|
|
|
|
|
|
|
|
|
+ |
S |
|
|
|
|
|
CH3 |
||||||||||||
P |
|
S |
|
|
|
|
P |
|
S |
P |
|
|||||||||||||||||||||||||||||
|
|
|
|
|
|
|
||||||||||||||||||||||||||||||||||
|
|
|
|
|
|
|
|
|
|
|||||||||||||||||||||||||||||||
|
|
|
|
|
|
|
|
|
|
|
|
|
|
|
|
|
|
|
|
|
|
|
|
|
|
|
|
|
|
|
|
|
|
|||||||
|
|
|
|
NH |
|
|
|
|
|
|
NH |
|
NH |
|||||||||||||||||||||||||||
|
|
|
|
|
|
|
|
|
|
|
|
|
|
|
|
|
|
|
|
|
|
|
|
|
|
|
|
|
|
|
|
|
|
|
|
|||||
|
|
|
|
|
|
|
|
|
|
|
|
|
|
|
|
|
|
|
|
|
|
|
|
|
|
|
|
|
|
|
|
|
|
|
|
|
||||
H3 CO2 C C H |
|
|
|
|
|
|
H C CO2 CH3 |
|
H C CO2 CH3 |
|||||||||||||||||||||||||||||||
|
|
|
|
|
|
|
|
|
|
|
|
|
|
|
|
|
|
|
|
|
|
|
|
|
|
|
|
|
|
|
|
|
|
|
|
|
||||
|
|
|
|
|
|
|
|
|
|
|
|
|
|
|
|
|
|
|
|
|
|
|
|
|
|
|
|
|
|
|
|
|||||||||
|
|
|
|
CH2 CH |
|
CH2 |
|
|
CH2 CH |
|
CH2 |
|
CH2 CH |
|
CH2 |
|||||||||||||||||||||||||
|
|
|
|
|
|
|
|
|
|
|
|
|
||||||||||||||||||||||||||||
|
|
|
|
|
|
|
|
|
|
|
|
|
|
|||||||||||||||||||||||||||
|
|
|
|
|
|
|
|
[(R,R)-93] |
|
|
|
|
|
[(R,S)-94] |
|
|
|
[(R,S)-95] |
The first nuclear magnetic resonance chiral lanthanide shift reagent (CLSR) reported was tris[3-(tert-butylhydroxymethylene)-d-camphorato]europium(III) (96), prepared from d-camphor82. Figure 5 illustrates the influence of this reagent on the 1H NMR spectra of the enantiomers of ˛-phenylethylamine (22). The large downfield shift of the resonances of 22 in the presence of 96 from their positions in the absence of 96 in carbon tetrachloride is the result of pseudocontact interaction between the europium(III) atom and a rapidly exchanging mixture of coordinated and free amine. Noteworthy in the spectra in Figure 5 are the frequency differences in the resonance of the corresponding protons of R -22 andS -22, which provide a useful method for determining the enantiomeric excess of chiral compounds including amines82.
CH3
O |
H C NH2 |
Eu/3
O
C(CH3)3
(96) |
[(S)-22] |
Other CLSRs have been developed83 and have been widely used for the determination of enantiomer ratios and the assignments of absolute configurations84. After complexation with europium(III) chloride (EuCl3), N,N0 -1,2-ethylenebis(L-aspartic acid) (L,L-97) and related N,N0 -1,2-ethylenebis(aspartic acid) ligands were recently reported85 as highly
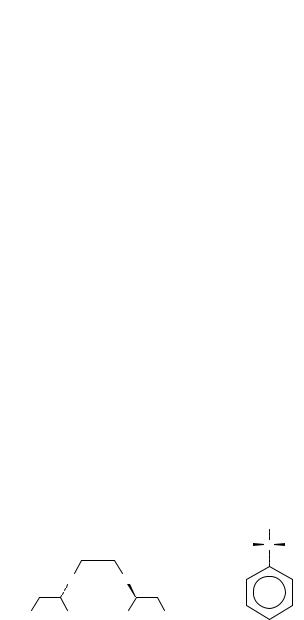
3. Chiroptical properties of amino compounds |
129 |
FIGURE 5. Proton NMR spectra of solutions prepared from S-˛-phenylethylamine [S-22] (10 mL) (upper spectrum) and a mixture of R- and S-˛-phenylethylamine [R- and S-22] (7 and 5 mL, respectively) (lower spectrum) in 0.3 mL of a carbon tetrachloride solution of tris[3-(tert- butylhydroxymethylene)-d-camphorato]europium(III) (96). The chemical shift scale applies only to the lower spectrum. Reprinted with permission from Reference 82. Copyright (1970) American Chemical Society
suitable shift reagents for the determination of enantiomeric ratios of partially racemic ˛-amino acids and ˛-alkyl-substituted ˛-amino acid in aqueous solution.
|
|
|
CF3 |
|
|
|
H C OH |
|
HN |
NH |
|
HO2C |
CO2H HO2C |
CO2H |
|
|
|
(L,L-97) |
[(R)-98] |
Related to the NMR use of CLSRs is the application of chiral solvating agents (CSAs), so-called because the first report of the determination of enantiomeric excess by NMR was that of a partially racemic sample of 2,2,2-trifluro-1-phenylethanol (98),

130 |
Howard E. Smith |
using |
R -˛-phenylethylamine [ R -22] as the NMR solvent86. Since this first report, |
a wide variety of combinations of solute and CSA functional group types have been reported87. Aryltrifluoromethylcarbinols such as 98 and ˛-arylethylamines such as 22 have been among the most extensively employed. This derives in part from their general applicability and in part from their commercial availability in enantiomerically pure forms. Chiral solvating agents of this type rely on hydrogen bonding as the primary solutebinding force. If the solute is a hydrogen bond acceptor such as a tertiary amine, the CSA of choice is an efficient hydrogen bond donor such as 98; and if the solute is a hydrogen bond donor such as an alcohol, the CSA is a hydrogen bond acceptor such as 22. The CSA concentration is usually chosen to be three or four times that of the chiral solute with an achiral cosolvent, aprotic and relatively nonpolar if possible, so chosen that competition with either the solute or CSA is avoided.
More recently R -O-acetylmandelic acid88 [ R -99], S -˛-methoxy-˛-(trifluoro- methyl)phenylacetic acid89 (MTPA) [ S -83] and other chiral acids89 have been used as CSAs. These acids form diastereomeric salts soluble in benzene-d6 or chloroform- d with a wide range of amines and amino alcohols, permitting a direct measure of their enantiomeric composition. Enantiomers of 1,10 -binaphth-2,20 -diol90 (100) and 1,10 - binaphth-2,20-diylphosphoric acid (101) and other derivatives of 100 and 101 have also been successfully used as CSAs in chloroform-d and benzene-d6 for the estimation of enantiomeric excesses of a number of ˇ-amino alcohols90, and enantiomers of 101 have been used for cyclic secondary and tertiary amines91.
CO2 H |
|
O |
H C OCOCH3 |
OH |
O |
|
P |
|
|
|
|
|
OH |
OH |
|
|
O |
[(R)-99) |
[(S)-100] |
[(S)-101] |
IV. ELECTRONIC CIRCULAR DICHROISM (ECD)
A. Amino Chromophore
As discussed in the earlier review of the chiroptical properties of the amino chromophore1, it was recognized very early on the basis of optical rotatory dispersion (ORD) measurements that for some chiral amines, Cotton effects are associated with the electronic transitions below 250 nm, and the ECD spectra are characterized by two oppositely signed dichroic absorption bands in the region 190 240 nm (Figure 6) corresponding to the electronic absorption maxima of trimethylamine in the gas phase at 199 and 227 nm1. The disappearance of the ECD bands on protonation of the nitrogen lone pair of electrons supports the conclusion that this dichroic absorption is associated with electronic transitions of the lone-pair electrons on the nitrogen atom1.
On the basis of a comprehensive ECD study of chiral 2-alkyl-substituted piperidines92, such as S -˛-pipecoline [ S -2-methylpiperazine, S -102] and their N-methyl derivatives [ S -103] in ethanol, the sign of the strong, long-wavelength CE near 200 nm, shifted to

3. Chiroptical properties of amino compounds |
131 |
MOLECULAR ELLIPTICITY [q] × 10−3
5
0
−5
200 220 240 260
−15
λ (nm)
FIGURE 6. Circular dichroism spectra of R-2-methylpyrrolidine [R-109] (solid line) and R-1,2- dimethylpyrrolidine [R-110] (dashed line) in hexane. Reproduced from Reference 94 by permission of Pergamon Press
|
|
H |
.. |
|
H |
H |
|||
|
|
H |
|
R |
H |
|
|
R |
|
|
|
|
|
|
|
||||
N |
R1 |
|
|
|
|
. |
|||
|
H |
|
CH3 |
H |
. |
||||
|
|
|
|
|
|||||
R2 |
|
H |
H |
|
H |
H |
|||
[(S)-102] R1 |
= CH3 |
, R2 = H |
|
|
|
|
|
|
|
[(S)-103] R1 |
= R2 = CH3 |
(104) |
|
|
|
(105) |
|
220 nm in cyclohexane and assigned to the n ! Ł transition of the amino group, is empirically correlated with the absolute configuration at C-292. For an N-methyl-2- alkylpiperidine in cyclohexane, the N-methyl group has an equatorial conformation (104), and the CE near 200 nm for the configuration shown in 104 is positive, and S-102 and S-103 show a positive CE near 200 nm. For other six-membered cyclic secondary amines in which the 2-alkyl group is larger than a methyl group, the lone pair of electrons on nitrogen is equatorial (105), and the CE is negative. Thus a counterclockwise (negative) direction (104) from the C-2 alkyl group to the lone pair of electrons results in a positive CE; a clockwise (positive) direction (105) gives a negative CE93. Similar ECD measurements show that this simple piperidine helicity rule predicts the sign of the observed positive CE near 200 nm for conformationally rigid R-quinuclidin-3-ol [R- 106] and also supports the predicted conformation and assigned absolute configuration for R-1-methylpiperidin-3-ol [R-107] and R-piperidin-3-ol [R-108], R-107 showing a positive CE near 200 nm while that of R-108 is negative93. When the ECD spectra of 2-substituted pyrrolidines R-109, R-110, S-111 and S-112 were studied94,

132 |
Howard E. Smith |
it was observed that as with the 2-substituted piperidines, N-methylation usually results in an inversion of the sign of the observed CEs (Figure 6). However, the sign of the long-wavelength CE at about 220 nm does not conform to the simple helicity rule found for the 2-substituted piperidines, since the pyrrolidine ring is itself chiral and makes its own contribution to the observed CEs. The rotational contribution due to the pyrrolidine ring chirality appears to be opposite in sign and larger in magnitude than that due to the 2-substituent in both the secondary and tertiary amines94.
|
|
OH |
|
|
|
OH |
|
|
|
|
N |
|
CH2 R1 |
|
N |
N |
|||
|
|
|
|
|
|
R |
R2 |
||
[(R)-106] |
[(R)-107] R = CH3 |
[(R)-109] R1 = R2 = H |
||
|
[(R)-108] R = H |
[(R)-110] R1 = H, R2 = CH3 |
||
|
|
|
[(S)-111] R1 = OH, R2 = H |
|
|
|
|
[(S)-112] R1 = OH, R2 = CH3 |
As a continuation of the studies with chiral piperidines and pyrrolidines, the ECD spectrum of the indolizidine S-1-azabicyclo[4.3.0]nonane [S-113] was compared with that of R-coniine [R-2-propylpiperidine, R-114] from which S-113 was prepared95. The optical rotatory dispersion (ORD) of S-114 showed a plain negative curve, descending steeply below 230 nm with a barely visible positive CE centered at 205 nm. For the hydrochloride of S-114, the CE at 205 nm was not present and an almost flat plain negative ORD curve to nearly 200 nm was seen. The free base S-113, which on the basis of its 1H NMR spectrum has its free pair of electrons trans to the hydrogen atom at C-6, and its hydrochloride both showed plain positive ORD curves, similar to that of R-114, and for S-113, also a positive CE at 206 nm. Since the configurationally related S-113 and R-114 each gives a CD maximum of the same sign and similar wavelength and magnitude for the n ! Ł transition of the nitrogen chromophore, the oppositely signed ORD curves for the two compounds must reflect a change in the net rotational contribution from transitions below 200 nm. From this and previous studies93,94, it appears that the indolizidine system as in 113 has its own chiroptical identity and cannot be treated as a simple pyrrolidine or piperidine chromophore. Since the trans indolizidine system with the S configuration gives a positive CE for the longest wavelength transition at 205 nm of the nitrogen chromophore, the sign of this CE may be useful in assigning the absolute configuration of substituted trans-fused indolizidines95.
H |
|
H |
N |
N |
CH2CH2CH3 |
|
N |
|
|
H |
|
|
|
|
[(S)-113] |
[(R)-114] |
[(S)-115] |
The ORD curves and ECD spectra of a number of chiral methyl-substituted cyclic amines, aziridine, azetidine, pyrrolidine and piperidine, and their N-methyl, N-halo and N-cyano derivatives, and of S-1-azabicyclo[3.1.0]hexane [S-115] were measured96.

3. Chiroptical properties of amino compounds |
133 |
The possibility of application of the quadrant rule for the N-chloramine derivatives was discussed, and a similar rule was also proposed for the N-cyano derivatives96.
In contrast to the study of conformationally rigid cyclic secondary and tertiary amines, the ECD of the enantiomers of 2-aminobutane (53) were studied in solution and in the gas phase over the wavelength region 150 260 nm97, and in addition to the CD maxima at shorter wavelength for the amino chromophore, a CD maximum was also detected at about 250 nm. This maximum is at a lower energy than that of the first excited state at 212 nm in the gas phase and 206 nm in hexane solution and is assigned to the forbidden singlet triplet transition of the amino chromophore, negative in the ECD spectrum ofR -53 and positive in that of S -5397.
In line with the current interest in the chemical and physical properties of chiral aziridines10, an experimental and theoretical investigation of the chiroptical properties of a series of N-haloaziridines and N-halodiaziridines, such as S - 1-chloro-2,2-dimethylaziridine [ S -76], (1S,2S)-1-chloro-2-methylaziridine [(1S,2S-77] and (1R,5S,6R)-6-chloro-5-methyl-1,6-diazobicyclo[3.1.0]hexane [(1R,5S,6R)-116], were described98, followed by a similar investigation of N-acetylaziridines99. A study of the ECD of C2-symmetric N-haloaziridines, such as (2S,3S)-1-chloro-2,3-dimethylaziridine [(2S,3S)-117], suggested that a quadrant sector rule for the N-haloaziridine chromophore connects the longest-wavelength CE sign in the 260 300 nm region with the stereochemical environment of the chromophore100. N-Haloaziridinecarboxylic esters are a specific case for the N-haloaziridine chromophore, and the sign of this CE cannot be correlated with their respective configurations. For these compounds, the second CE sign at 220 230 nm in the ECD spectra obeys a reverse quadrant sector rule100. Finally, the chiroptical properties of the bridgehead aziridine S -1-azabicyclo[3.1.0]hexane [ S -115] have been investigated experimentally and theoretically in the ultraviolet and infrared absorption regions101. The ECD spectrum of S -115 was also measured in the gas phase in the vacuum ultraviolet region (150 200 nm)101, and the three ECD maxima detected below 200 nm were assigned to the transitions of the lone pair on the nitrogen atom101, but no simple chirality rule emerged from this UV-ECD study101.
H3 C |
|
H3 C |
CH3 |
|
|
|
|
|
N |
|
N |
Cl |
|
|
|
N |
|
Cl |
|
|
|
|
|
[(1R,5S,6R)-116] |
[(2S,3S)-117] |
B. Effect of the Amino Group on the Benzene Chromophore
1. Benzene sector rule
The influence of the amino group on the carbonyl, the carboxyl and the benzene chromophores was reviewed earlier1. Since then significant work only with regard to the influence of various groups, including the amino, substituted amino and ammonium groups, on the benzene chromophore has been published, including far-ultraviolet ECD
observations on chiral ˛-phenylalkylamines and ˛-phenylalkylamine hydrochlorides102, and reviewed103,104, and has resulted in the formulation of the benzene sector rule105 and the benzene chirality rule38.
The benzene sector rule105 may be used to correlate the absolute configuration of a contiguous chiral center of a monosubstituted benzene compound with the sign of the CE

134 |
Howard E. Smith |
∆ε |
|
|
ε |
+ 0.15 |
|
|
300 |
+ 0.10 |
CD |
|
|
|
|
|
|
+ 0.05 |
|
|
200 |
± 0.00 |
|
|
|
|
|
EA |
100 |
|
|
|
|
|
|
|
0 |
225 |
250 |
275 |
300 |
Wavelength, nm
FIGURE 7. Electronic absorption (EA) and circular dichroism (CD) spectra of S-˛-phenylethylamine [S-22] in methanol. Reproduced from Reference 103 by permission of the Croatian Chemical Society
H
C R2
R1
(118)
R
( )n
N
CH3
[(R)-119] n = 1, R = H [(R)-120] n = 2, R = H [(R)-121] n = 1, R = CH3 [(R)-122] n = 2, R = CH3
associated with 1Lb electronic transitions from about 250 to 270 nm (Figure 7). These CEs are associated with transitions to totally symmetric vibrational modes in the lowestenergy electronically excited state and are the result of vibronic borrowing from benzene transitions at shorter wavelength. The sign of these CEs depends only on the chirality of the center immediately attached to the benzene ring, and for such benzene compounds with a hydrogen atom at this chiral center, molecular orbital calculations and spectroscopic measurements indicate that the preferred conformation is such that the hydrogen atom at the chiral center eclipses or nearly eclipses the phenyl ring plane105. Thus the preferred conformation of S-˛-phenylethylamine [S-22] is shown as 118 R1 D NH2, R2 D CH3 . This conformational preference and ECD data for a whole host of chiral