

13. Diazotization of amines and dediazoniation of diazonium ions |
637 |
complexes of the diazonium ions with the arenesulfonates rather than to diazosulfonates (ArN2 OSO2Ar0 ) as previously thought. For a diazonium ion in acetic acid/water (4:1), the complex equilibrium constants are found to increase in the order naphthalene < 1- methylnaphthalene < naphthalene-1-sulfonic acid < 1-naphthylmethanesulfonic acid. The sequence reflects the combined effects of the electron-donor properties of these compounds and the Coulomb attraction between the diazonium cation and the sulfonate anions (where present). Arenediazonium salt solutions are also stabilized by crown ethers.
C. Diazotization of 2- and 4-Aminophenols
Diazotized 2- and 4-aminophenols as well as corresponding diazotized aminonaphthols and hydroxy derivatives of higher condensed aminoaromatic systems exist in neutral aqueous solutions as zwitterions (23b) which are mesomeric with the corresponding quinone diazides (23a). They can therefore be classified either as diazonium ions or as diazoketones. Indeed, preparative methods for these compounds include those typical for diazonium ions and those used for diazoketones.
|
+ |
− |
−O |
+ |
O |
N |
N |
N N |
|
|
(23a) |
|
|
(23b) |
As the preparative methods related to the syntheses used for diazoketones are no diazotizations and, in part, do not start from amines, they are not within the scope of this book. They are reviewed in Zollinger’s books on diazo chemistry3b,7c.
A compilation of some 240 examples in the book by Ershov, Nikiforov and de Jonge44a demonstrates, however, that the most frequently used method is diazotization, applied to 2- or 4-aminophenols in the same or a similar way as described in Section II.A. It may be recalled that the very first diazotization (Griess, 1858) was carried out with an aminophenol derivative.
The major problem of these diazotizations is oxidation of the initial aminophenols by nitrous acid to the corresponding quinones. Easily oxidized amines, in particular aminonaphthols, are therefore commonly diazotized in a weakly acidic medium (pH ³ 3) so-called neutral diazotization or in the presence of zinc or copper salts. This process, which is due to Sandmeyer, is important in the manufacture of diazo components for metal complex dyes, in particular those derived from 1-amino-2-naphthol-4-sulfonic acid.
Sometimes decomposition reactions can be avoided by carrying out diazotizations in concentrated sulfuric acid. By this method Law and coworkers45 obtained the 1,5-bisdiazonium salt (incorrectly called tetrazonium salt) of 1,5-diamino-4,8- dihydroxyanthraquinone, which is deprotonated to 24. The structure was verified by cross-polarization magic angle spinning (CPMAS) 13C NMR spectroscopy.
O O N2
N2 |
O |
O |
(24)

638 |
Heinrich Zollinger |
On the other hand, there is at least one case of an aromatic amine without a hydroxy group in the 2-position, namely 1-aminophenazine (25) which, after the initial diazotization, is oxidized within minutes by air or additional nitrous acid to the quinone diazide 26 (equation 9)46. In the corresponding diazotization of 2-aminophenazine the proportion of the quinone diazide (isomer of 26) amounted to only 16%, but 30% unsubstituted phenazine was also found. The phenazine may have resulted from the overall redox reaction.
NH2 |
|
N2 |
N |
N |
O |
NaNO2 |
|
|
HCl |
oxidation |
(9) |
|
|
|
N |
N |
|
(25) |
(26) |
|
The diazonio group is characterized by extremely strong -M and -I effects. Therefore aromatic diazonium ions with a strongly nucleofugic leaving group in the 2- or 4-position readily undergo nucleophilic aromatic substitution of that leaving group by a hydroxy group, forming a quinone diazide. Primarily suitable for this type of substitution are compounds with halogens as leaving groups, but there are also cases in which a nitro group has been replaced by a hydroxy group, for example 4,2-chloronitrobenzene-diazonium ion hydrolyzes by denitration, forming 4-chloro-2-quinone-1-diazide47,48. On the other hand, in 2,3,5,6-tetrafluoro-4-nitroaniline the fluorine rather than the nitro group is displaced49.
Similar nucleophilic substitutions of other groups during diazotizations (chloro-de- nitrations) were found by Oku and Matsui50 and Trimmer51.
The general applicability of this type of synthesis of quinone diazides is neverthe-
less |
limited since, |
depending on the type and number of substituents in the 2-, 4- |
and |
6-positions of |
benzenediazonium ions, either hydroxy-de-diazoniation (reaction A |
in Scheme 4) or nucleophilic substitution of one of the groups in the 2-, 4- or 6-position (reaction B) will predominate52.
N |
+ |
2 |
|
|
+ H2 O |
|
NO2 |
Cl |
|
A |
B |
OH |
N2 |
+ N2 + H+ |
+ Cl− + 2H+ |
NO2 |
NO2 |
Cl |
O |
SCHEME 4

13. Diazotization of amines and dediazoniation of diazonium ions |
639 |
Recently, the substituent effect on the diazotization of substituted 2-aminophenols was studied53 in aqueous hydrochloric acid solution. When the substituent is strongly electron-withdrawing, such as a nitro group, the diazotized product deprotonates and forms a substituted 1,2-benzoquinone-1-diazide which usually precipitates.
Syntheses of complex heterocyclic quinone diazides have been tabulated by Ershov, Nikiforov and de Jonge44b and more recently by Tisler’sL group54.
D. Formation of Diazonium Salts under Anhydrous Conditions
In the classical methods of diazotization an equimolar amount of water is formed as by-product. In certain cases this is not desirable. One method for obtaining diazonium salts without formation of water is the rearrangement of N-nitroso-N-arylacetamides. Bamberger55 discovered in 1897 that N-nitrosoacetanilide decomposes easily in benzene to give biphenyl, acetic acid and dinitrogen (equation 10). Huisgen and Horeld56 showed that the key step of Bamberger’s reaction is the isomerization of N-nitrosoacetanilide to the diazo acetate (27) and Suschitzky57 found that 27 dissociates into the diazonium-acetate ion pair 28 (equation 11).
C6H5N(NO)COCH3 C C6H6 ! C6H5C6H5 C N2 C CH3COOH (10)
N OCOCH3
C6 H5N(NO)COCH3 C6 H5N
(27)
(11)
[C6 H5N2 + CH3 COO− ]
(28)
The consecutive dediazoniation is fast relative to the rate-determining steps in equation 11. The influence of the presence or absence of water on these dediazoniations of ion pairs like 28 will be discussed briefly in Section III.A of this review.
A second method for forming diazonium ions was initiated by Turcan58 in 1935. He reported that nitrosyl chloride and dinitrogen tetroxide react with N-benzylideneaniline under mild conditions in anhydrous ether or benzene to produce benzaldehyde and the corresponding benzenediazonium salt (equation 12). After nitrosonium salts became readily available (Olah and coworkers59) Doyle’s group60 demonstrated that with NOCBF4 or
in anhydrous acetonitrile or nitromethane yields are usually higher than 90%. Bachman and Michalowicz61, Zimmer and Singh62 and Bott63 found analogous ways of nitrosating other compounds of the type Ar NDY, where Y is CO (phenylisocyanate), P(C6H5)3 (triphenylphosphinephenylimine) or SO (sulfinylphenylimine).
C6H5CH |
|
NC6H5 + XNO |
|
C6H5CHO + C6H5N2+ X − |
(12) |
|
|
||||
|
|
X = Cl, NO3, etc.
Nitrosyl halides, particularly NOCl and NOBr, can also be used as diazotization reagents under anhydrous conditions, as these gases (NOCl: mp 59.6 °C, bp 6.4 °C; NOBr: bp ³ 0 °C) are readily soluble in many organic solvents. They can also be generated in situ in chlorinated hydrocarbons by reaction of trimethylsilyl halides with alkyl nitrites (equation 13) as shown by Weiss and Wagner64. The group of Weiss65 found also that N,N-bissilylated anilines react in aprotic dichloromethane with generation of diazonium salts and formation of the nonnucleophilic hexamethyldisiloxane (equation 14).

640 |
Heinrich Zollinger |
The authors indicate that the monosilylated aniline C6H5NHSiMe3 reacts in many cases in an analogous way. This seems surprising, since the hydroxytrimethylsilane HOSiMe3 that is formed is a potential proton donor, as it will rapidly condense to give Me3SiOSiMe3 C H2O.
RO |
|
NO |
C |
Me3SiX ! XNO |
C |
RO |
|
SiMe3 |
(13) |
|
|
|
|
|
|
||||
|
|
|
|
X D Cl, Br, l |
|
|
|
|
|
C6H5N[SiMe3]2 C XNO ! C6H5N2C X C [Me3Si]2O |
(14) |
Furthermore, Weiss and coworkers66 developed also a method of diazotizing aminocyclopropenium salts with nitrosyl salts in the presence of two equivalents of trimethylchlorosilane to trap the water produced. In a modification of this method the authors showed that the use of the tert-butylated amine was an advantage, as the risk of oxidation of the alkylated amino group by the nitrosyl ion is less than in the case of the primary amine. This method would also appear to be suitable for the diazotization of other sensitive amines.
2-Amino- and 4-amino-1,2,4-triazole undergo a reductive dediazoniation to 1,2,4- triazole when treated with nitrosonium tetrafluoroborate in the presence of mesitylene67.
Doyle and Bryker68 reported high yields of arenediazonium tetrafluoroborates when aromatic amines were reacted with tert-butyl nitrite and trifluoroboro etherate in CH2Cl2. It is likely that nitrosyl fluoride is formed as nitrosating reagent by fluoride-alkoxy exchange.
E. Mechanism and Kinetics of Rate-limiting Nitrosation Steps
Challis and Butler’s review on diazotizations in the volume on amines in The Chemistry of Functional Groups series1 was published at a time (1968) when the principal mechanistic aspects of these reactions and the pioneering kinetic investigations allow a consistent description and evaluation of the characteristics of diazotizations to be given. In particular, there was convincing evidence for nitroso-de-protonation as the essential part of the diazotization. Kinetic results were consistent with three types of reacting nitrosation reagents, namely depending on reaction conditions with dinitrogen trioxide
(nitrous anhydride, ON ONO), nitrosyl halides and a cationic nitrosating reagent which
+
was either the nitrosonium ion (ONC ) or the nitrosoacidium ion (ON OH2). Corresponding to Ingold’s general concepts of electrophilic substitutions it was assumed that, as a substrate, only arylamines, but, under strongly acidic conditions, arylammonium ions were considered with some reservation to be also capable of being reactive partners in diazotizations.
We are gratified to realize, almost thirty years later, that these results are essentially still valid. We owe this pleasant development first of all to Ridd. He started to investigate the mechanism of diazotization under Ingold and Hughes in 1950 and continued it independently until the early 1990s. Some of his former coworkers and other chemists became active in the same field.
The classical discoveries of the reacting nitrosation reagents mentioned above were mainly the result of kinetic investigations. There were some surprising results, e.g. the dependence of the diazotization rate on the square of the nitrous acid concentration in sulfuric or perchloric acid up to 0.3 M. Nitrous acid is a fairly weak acid (pKa D 3.1569). Therefore, the low concentration of nitrite ions in the last step of the nitrous acid equilibria system of Scheme 5 does not appear to favor the formation of dinitrogen trioxide. N2O3 is, however, strongly indicated by the second order of rate on the (analytical) nitrous acid concentration.
Yet at fairly high nitrous acid concentrations (0.1 M) and at moderate acidities (4 M) the blue color of N2O3 ( max D 625 nm) is easily detected by eye. A relatively recent

13. Diazotization of amines and dediazoniation of diazonium ions |
641 |
||||||||||
NO2 |
C |
HC |
! HNO2 |
|
|
|
|
|
|||
|
|
|
|
+ |
|
|
|
|
|
||
HNO2 |
|
|
HC |
! |
! |
NOC |
|
H2O |
|
||
C |
H2 O NO |
C |
|
||||||||
NOC |
|
|
|
|
|
|
|
|
|||
C |
NO2 |
! |
ON O |
NO |
|
|
|
|
|||
|
|
|
|
|
|
|
|
|
|
SCHEME 5
determination of the overall equilibrium constant of the system (equation 15) gave the value K D 3.0 ð 10 3 M70. Accurate determination of this constant is difficult, since N2O3 decomposes easily into NO and NO2. Pure N2O3 is stable only as a pale blue solid or as an intensely blue liquid just above its freezing point ( 100 °C). The liquid starts to boil with decomposition above 40 °C. In spite of these qualitative properties, the value of K tells us clearly that the nucleophilicity of nitrite ion is sufficiently high for the last equilibrium of Scheme 5 to be effective.
|
K |
|
|
|
|
2HNO2 |
! |
N2O3 |
C |
H2O |
15 |
|
|
|
|
|
The decomposition of nitrous acid solutions was investigated by Bayliss and Watts71 in 10 65% sulfuric acid. The rate constant for decomposition at 19.5 °C increases from 2.5 ð 10 4 s 1 in 10% H2SO4 to 14 ð 10 4 s 1 in 50% H2SO4, but decreases to almost zero in 65% H2SO4. As the maximum rate of decomposition occurs in those solutions in which both the nitrosyl ion (or the nitrosoacidium ion) and the nitrous acid molecule are present in appreciable concentrations, it is likely that the main pathway is that shown in equations 16 and 17. A more detailed inspection of Bayliss’s and Watt’s data (unfortunately given only in a figure, and not numerically) indicates, however, that there are other parallel reactions leading to decomposition.
HNO2 |
C |
NOC H2O |
! |
N2O3 |
C |
HC (H2O) |
(16) |
|
|
|
|
|
|
||
N2O3 |
! NO C NO2 |
|
|
|
(17) |
At higher concentration of sulfuric acid (>0.3 M), diazotization rates are no longer second order with respect to nitrous acid, but first order. Therefore, N2O3 cannot be the nitrosating reagent. The marked acid catalysis indicates that the new agent is some species whose equilibrium concentration increases rapidly with increasing acidity. It may
be the nitrosoacidium ion, but could also be the nitrosyl ion. Arguments for either NOC
+
or H2 O NO or both as nitrosating reagent were discussed in the literature for many years. The arguments have been reviewed by Ridd72a and by Williams73a. In the opinion
of the present author, there is no experimental evidence that clearly falsifies either NOC
+
or H2 O NO as reagent in water.
More than thirty years ago Seel and Winkler74 as well as Bayliss and coworkers75 investigated the UV absorption spectra of sodium nitrite in aqueous solutions of sulfuric and perchloric acids (equation 18, for H2SO4). The absorption band found at 250 nm
is due either to the nitrosoacidium ion or to the nitrosyl ion. From the absorbency of
+ +
this band the equilibrium concentrations of HNO2 and NO or H2 O NO were calculated over the acid concentration ranges 0 100% H2SO4 (by weight) and 0 72% HClO4 (by weight). For both solvent systems the concentrations determined for the two (or three) equilibrium species correlate with the acidity function HR. This acidity function is defined for protonation dehydration processes, and it is usually measured using triarylcarbinol indicators in the equilibrium shown in equation 19 (for a relatively recent review see Cox and Yates76).

642 |
|
|
|
|
|
|
Heinrich Zollinger |
|
|
|
|
|
|||||||
H2SO4 |
|
|
HNO2 |
! |
H2 |
+ |
|
|
NO |
|
HSO4 |
! |
NOC |
|
H2O |
|
HSO4 |
||
C |
O |
|
C |
C |
C |
||||||||||||||
|
|
|
|
|
|
|
|
|
|
|
|
(18) |
|||||||
|
|
|
|
|
|
|
|
|
|
|
|
|
|
|
|
|
|
|
|
Ar3COH |
C |
HC |
! |
Ar3CC |
C |
H2O |
|
|
|
|
|
|
(19) |
||||||
|
|
|
|
|
|
|
|
|
|
|
|
|
|
|
|
The carbinol equilibrium (equation 19) is comparable to the nitrous acid equilibrium (equation 18), but this correlation does not exclude the nitrosacidium intermediate because the corresponding protonated carbinol was not positively excluded in equation 19.
A speculative proposal was made thirty years ago by Schmid and Krenmayr77, namely that a nitrosyl ion solvated, but not covalently bonded, by a water molecule may be involved in these systems. This hypothesis was investigated theoretically in 1984 by Nguyen and Hegarty78 who carried out ab initio SCF calculations of structure and properties employing the minimal STO-3G basis set, a split-valence basis set plus polarization functions. Optimized geometries of six planar and two nonplanar forms were studied for the nitrosoacidium ion. The lowest minimum of molecular electrostatic potential resulted for the structure 29. Among the bond lengths it is indeed the N O(2) bond (196.8 pm) that turns out to be significantly longer than expected for an N O single bond (120 140 pm). Species 29 is therefore indeed a complex which, in classical terms, may be called a nitrosyl ion solvated with one molecule of water. The complex is calculated to be more stable than its fragments by 75 kJ mol 1.
H(1)
|
131.1° |
97.2 |
|
|
|
97 |
|
|
108.1° |
O(2) |
117.8°H(2) |
O(1) |
N |
196.8 |
|
|
106.2 |
|
|
(29)
Shortly afterwards Jørgensen and Lawesson79 investigated the same problem based on the concept of reactions controlled by charge and frontier orbitals80,81. Geometrical optimization has been performed using a 4-31G basis set. The calculated N O(2) bond length is even a little longer than in Nguyen and Hegarty’s work, namely 212.8 pm. The energy of the lowest unoccupied molecular orbital of the free nitrosyl ion is 123 kJ mol 1 lower than the LUMO energy for the hydrated complex. This difference might account for the difference in reactivity. Jørgensen and Lawesson interpret these results on the basis of Coulomb interactions rather than orbital interaction.
On the basis of theory it is therefore now more likely that the solvated nitrosyl ion, but not the nitrosoacidium ion nor the ‘free’ nitrosyl ion, is the nitrosating agent in diazotization in moderately to strongly acidic solutions.
Is the anilinium ion able to react with a nitrosating reagent in strongly acidic solutions?
+
Originally it was assumed that unsubstituted and alkylated ammonio groups like NH3
+
and N(CH3)3 are prototypes of substituents without electron-donating power. Since quite some time, however, a large mass of data obtained with several completely different probes82 indicates that these ammonio groups are modest -donors with resonance effects similar to that of the isoelectronic tert-butyl group CMe3. This is best seen in Taft’s dual
substituent parameter RC83,84 : NMe3 has the value RC D 0.11, similar to the value0.18 for CMe385. These negative values are clear evidence for electron donation. The
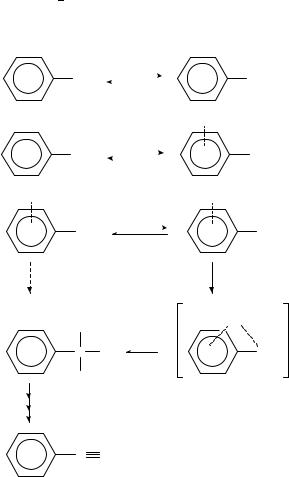
13. Diazotization of amines and dediazoniation of diazonium ions |
643 |
donor activity of the ammonio substituent is, however, much smaller than that of the amino group, for which RC D 1.7583.
Ridd and coworkers86 found that the logarithm of the diazotization rate constant of aniline increased in the acidity function range H0 C0.5 to 2.9 linearly with a slope of 1. This result was interpreted with a slow step involving first the formation of a chargetransfer complex of the nitrosyl ion with the -electrons of the anilinium ion, followed by the migration of the nitroso group to the nitrogen atom of the ammonio group. This rearrangement is postulated to be concerted with the transfer of an ammonio proton to a base of the system (H2O). The formation of a charge-transfer complex as intermediate is consistent with the fact that rates of diazotization are greater for naphthylamines than for anilines and aminopyridines of similar pK87a ,88. Zollinger89 proposed a slight change in that part of the mechanism involving the migration of the nitroso group. The pKa of the dicationic charge-transfer complex must be significantly lower than that of the anilinium ion: electrophilic complexation of any benzene derivative bearing a Brønsted acid group such as NH3C will increase the acidity constant of that group. Incorporating this consideration into the reaction mechanism of diazotization, the modified Ridd mechanism is shown in equations 20 24. In the original Ridd mechanism the steps 22 and 23 were combined in one step (here indicated by the dashed arrow). The inclusion of the solvated nitrosyl ion and the release of a water molecule in 21 is tentative. The original papers of Ridd86 and Zollinger89 contain only the (free) nitrosyl ion.
+ |
|
|
|
pKa ( N ) |
|
|
|
+ H+ |
(20) |
NH3 |
|
|
|
|
|
|
NH2 |
||
|
|
||||||||
|
|
|
|
||||||
|
|
|
|
|
|
|
NO+ |
|
|
+ |
|
|
|
+NO+(H2O) |
+ |
+ H2O |
(21) |
||
NH3 |
|
|
|
|
|
NH3 |
|||
|
|
|
|
||||||
|
|
|
|||||||
NO+ |
|
|
|
|
NO+ |
|
|
||
+ |
|
|
|
pKa ( π) |
|
|
NH2 + H+ |
(22) |
|
NH3 |
|
|
|
|
|
||||
|
|
|
|
|
δ+
NΟ |
NO |
|
N+ H |
δ+ |
(23) |
NH2 |
|
H
+ |
pKa(N) |
>> pKa ( π) |
|
N N |
(24) |
||
|
|
|

644 |
Heinrich Zollinger |
At very high acidities H0 < 3.5 the logarithmic rate constant of diazotization decreases again. The dependency of log k is linear in h0 2.1. At these high acidities the nitrous acid equilibrium (equation 18) is almost completely on the side of the (solvated) nitrosyl cation and that of the amine on the side of anilinium ion. Together with additional experimental data Ridd concluded already in 1960 that the deprotonations of the - nitrosoanilinium dication (equation 22) and of the N-nitrosoanilinium ion (equation 24) belong to the rate-determining part of the reaction. We do not discuss these conditions because they were reviewed already by Challis and Butler in the original volume on amines in this series1.
Aminopyridines90,91, aminopyridine-1-oxides25, 3-aminoquinoline92 and 2-aminothi- azole93 are obviously diazotized by analogous processes. For 4-aminopyridine, 3-amino- quinoline and 2-aminothiazole it was shown that the monocation, protonated at the heterocyclic nitrogen, is nitrosated preferentially.
F. Nucleophilic Catalysis and the Transformation Mechanism of N -Nitrosoamines into Diazonium Ions
In the preceding section we concentrated on the rate-limiting steps of diazotizations in aqueous sulfuric and perchloric acid. The results were the identification of dinitrogen trioxide and (solvated) nitrosyl ions as electrophilic reagents, aniline and anilinium ion as nucleophilic reagents and an interpretation of the influence of acidity.
More complex diazotizations in other mineral acids and the pathways of the transformations of the primarily formed N-nitrosoamines are the subject of this section.
As found by Schmid in the 1930s94 diazotizations in aqueous hydrochloric or hydrobromic acid are more complex because they are catalyzed by halide ions. Hammett95a postulated an equilibrium (equation 25) which is shifted to the right on increasing the concentration or the nucleophilicity of the nucleophile X . Hydrogen sulfate and perchlorate ions are very weak nucleophiles; they are not measurably effective. In the absence of other nucleophiles the nitrite ion will be the only candidate in aqueous H2SO4 solutions (except H2O and OH , of course) and N2O3 is formed (see Section II.E). Although NO2 is more strongly nucleophilic than Cl or Br , the halide ions will be more effective in dilute hydrochloric or hydrobromic acid, since their concentrations are much higher. Nitrosyl chloride and nitrosyl bromide are formed, which act as nitrosating agents in such systems. Later it was shown by various authors that other nucleophiles have an analogous effect, namely thiocyanate ion (SCN ), thiosulfate ion (HS2O3 ), dimethyl sulfide and thiourea (H2NCSNH2) or its alkyl derivatives. Equilibrium constants K for the formation of nitrosyl compounds XNO in equation 27 as well as diazotization rates with aniline, 2- and 4-chloroaniline, 1-naphthylamine and other aromatic amines were measured (see summary by Williams73b and by Zollinger7d).
HNO2 |
|
HC |
|
X |
|
k1 |
|
|
H2O |
|
|
(25) |
|||
C |
C |
! XNO |
C |
|
|
||||||||||
|
|
|
|
|
k |
|
|
|
|
|
|
||||
|
|
|
|
|
|
|
|
|
|
|
|
|
|
||
|
|
|
|
|
|
|
|
|
1 |
|
|
|
|
|
|
|
|
|
|
|
|
k2 |
|
|
|
+ |
|
fast |
+ |
|
(26) |
|
|
|
|
|
|
|
|
|
|
|
|
||||
XNO + ArNH2 |
|
|
|
|
|
ArNH2 |
NO ( |
|
ArN2 |
) |
|
||||
KXNO D |
k1 |
D |
HNO2 [HC ][X ] |
|
|
|
(27) |
||||||||
k 1 |
|
[XNO] |
|
|
|
|
|
k2 HNO2 [HC ][X ] ArNH2 Ka
rate D (28)
KXNO [HC ] C Ka
Ka D acidity constant of ArNH3C

13. Diazotization of amines and dediazoniation of diazonium ions |
645 |
The constants Ka increase with increasing nucleophilicity of X (Cl < Br < SCN < H2N 2CS). The rate constants for the nitrosation step (k2 in equation 26) are close to diffusion control in the case of X D Cl or Br but lower for reactions with nitrosation reagents XNO with stronger nucleophiles X (as expected). As in the case of diazotization by N2O3 (Section II.E), either the formation of XNO or the nitrosation may be ratelimiting. The rate equation 28 is applicable only for reactions with a steady-state of XNO.
For a representative and relatively recent example of a comparative kinetic investigation of diazotization of aniline and 1-naphthylamine with various catalysts X , reference is made to the work of Castro and coworkers98. These authors found that the (overall) rates decrease under comparable conditions in the order X D Cl > Br > SCN > H2N 2CS > HS2O3 . This sequence is not caused by higher nitrosation reactivity k2 of the nitrosating reagent XNO, but by higher values for KXNO in the equilibrium (equation 25).
The nitrosation step (equation 26) was studied in the last ten years in more detail with respect to diffusion-controlled preassociation phenomena and the steps following the formation of the N-nitrosoanilinium ion in the step in equation 26. Besides the case of the diazotization of very reactive aniline derivatives where the formation of the nitrosyl
halide (equation 25) is rate-limiting97, deprotonation of the N-nitrosoanilinium ion may
+
be rate-limiting, namely if the denitrosation of Ar NH2NO, reforming Ar NH2, is faster than deprotonation88,98. For this denitrosation two mechanisms seem to be plausible, as shown in Scheme 6, namely either a monomolecular N N bond dissociation into the amine and a nitrite ion which ultimately give NOCl again (A), or a bimolecular reaction of the N-nitrosoanilinium ion with a chloride ion (B).
|
|
|
|
ArNHNO |
fast |
ArN2+ |
|
|
|
|
|
|
|||
|
|
|
|
k 3 |
−H+ |
|
|
|
|
k2 |
+ |
|
|
|
|
|
|
|
|
|
|||
ArNH2 + NOCl |
|
|
|
ArNH2NO + Cl− |
|
||
|
k−2 |
|
|||||
|
|
B |
k′ |
A |
|
||
|
|
k′′ |
|
|
|
|
|
|
|
ArNH2 + NO+ |
|
||||
|
|
+ Cl− |
|
|
|
|
SCHEME 6
The work of the groups of Casado88 and Castro98, as summarized in Scheme 6, brings us to the subsequent steps which are faster and therefore not easily accessible to mechanistic studies. Scheme 7 summarizes the most reasonable, but still tentative, mechanism.
It is worth noting, however, that the prototropic equilibrium between the N-nitrosoamine (30) and the diazenol (31)† has been determined semiquantitatively for the analogous diazotization of an aliphatic amine. Fishbein and coworkers100 determined an upper limit for the nitrosoamine equilibrium concentration (<1.5%).
† The new Guide to Nomenclature of IUPAC99 includes a major change for naming diazo compounds of the general type R N2 X. These compounds are considered as derivatives of diazene (HNDNH). Therefore diazohydroxides (or diazotic acids) and diazoates acquired the names diazenols and diazenolates, respectively.

646 |
|
|
|
Heinrich Zollinger |
|
|
|
|
|
|
|
|
|
|
|
||||||||
N O |
|
|
|
|
N |
+ |
|
|
|
|
|
|
|
|
|
|
|
|
|
|
|
||
|
+ H+ |
|
|
OH |
|
− H+ |
|
|
|
|
|
|
|
|
|
|
|
||||||
Ar N |
|
|
Ar N |
|
|
|
|
|
Ar |
|
|
N2 |
|
|
OH |
(Z or E) |
|||||||
|
|
|
|
|
|
|
|
|
|
|
|
|
|
||||||||||
|
|
|
|
|
|
|
|
|
|
|
|
|
|
||||||||||
|
|
|
|
|
|
|
|
||||||||||||||||
H |
|
|
|
|
H |
|
|
|
|
|
|
|
|
|
|
|
|
|
|
|
|
|
|
(30) |
|
|
|
|
|
|
|
|
|
|
|
|
|
|
|
(31) |
|
|
|
|
|
||
|
|
|
|
|
|
|
|
|
|
|
|
|
|
|
|
|
|
+ H− |
|
||||
|
|
|
|
|
|
|
|
|
|
|
|
|
|
|
|
|
|
|
|||||
|
|
|
+ |
|
|
|
|
|
|
|
|
|
|
|
|
|
+ |
|
|||||
|
|
|
|
|
|
|
|
|
|
|
|
|
|
|
|
|
|||||||
|
|
|
|
Ar |
|
N |
|
N |
|
|
|
|
|
Ar |
|
|
N2 |
|
|
OH2 |
(Z or E) |
||
|
|
|
|
|
|
|
|
|
|
|
|||||||||||||
|
|
|
|
|
|
|
|
|
|
|
|
|
|
|
|||||||||
|
|
|
|
|
|
|
|
−H2 O |
|
||||||||||||||
|
|
|
|
|
|
|
|
|
|
|
|
|
|
|
|
|
|
|
|
|
|
SCHEME 7
Nevertheless, it is interesting that in the diazotization of a series (probably the majority) of five-membered heteroaromatic amines, at least one of the intermediates can be observed as a relatively long-lived transient species in appreciable concentration. Our understanding of the reaction conditions under which such intermediates are formed in detectable amounts is due mainly to Goerdeler and coworkers around 1960. Ginsberg and Goerdeler101 nitrosated 5-amino-1,2,4-thiadiazole (32) in apolar solvents (e.g. ether) and isolated a compound, which was shown to be the corresponding nitrosoamine (33) on the basis of its IR spectrum. Addition of acetic acid and BF3 transformed this intermediate into the diazonium ion (34). The reaction in equation 29 is consistent with the general pattern of Scheme 7. Even under aqueous conditions the nitrosoamine 33 can be obtained if a relatively low concentration of H2SO4 (1 M) is used.
|
N |
|
N |
NH2 |
HNO2 |
|
||
|
S |
|
|
(32) |
|
|
|
N |
|
|
N |
|
NHNO |
HOA c |
|
S |
BF3 |
|||
|
|
|||
|
|
|
||
|
(33) |
|
|
|
N |
|
N |
N2 + |
(29) |
|
S |
|
(34) |
|
|
More recently, other N-nitrosoamines of heteroaromatic compounds were obtained and characterized by the groups of Butler and of Stepanov102. Therefore, at least the nitrosoamine 33 does appear to be reasonably well documented as an intermediate on the pathway to the diazonium ion. A more extended review on the intermediates of Scheme 7 was published recently7e.
III. DEDIAZONIATIONS
As mentioned in the introductory section of this chapter, dediazoniation was reviewed in a volume of The Chemistry of Functional Groups series relatively recently (1983)2 and again in more detail in a monograph in 19947f. Therefore, we will concentrate here on a selection of major results published since the early 1980s on mechanisms of dediazoniations and on applications of dediazoniations in organic synthesis.
A. Mechanisms of Dediazoniations
Basically, diazonium ions can lose dinitrogen by three mechanisms:
(1) By heterolytic dissociation into carbocations and dinitrogen followed by the addition of a nucleophile (called DN C AN mechanism in the new IUPAC nomenclature103, SN1 in the former Ingold nomenclature).