
Chivers T. - A Guide to Chalcogen-Nitrogen Chemistry (2005)(en)
.pdfCyclic Chalcogen Imides |
121 |
23.R. Faggiani, R. J. Gillespie, C. J. L. Lock and J. D. Tyrer, Inorg. Chem., 17, 2975 (1978).
24.M. B. Hursthouse, K. M. A. Malik and S. N. Nabi, J. Chem. Soc., Dalton Trans., 355 (1980).
25.G. Schmid, R. Greese and R. Boese, Z. Naturforsch., 37B, 620 (1982).
26.T. Chivers, F. Edelmann, U. Behrens and R. Drews, Inorg. Chim. Acta, 116, 145 (1986).
27.K. Bergemann, M. Kustos. P. Krüger and R. Steudel, Angew. Chem., Int. Ed. Engl.,
34, 1330 (1995).
28.R. Steudel, O. Schumann, J. Buschmann and P. Luger, Angew. Chem., Int. Ed. Engl., 37, 492 (1998).
29.R. Jones, D. J. Williams and J. D. Woollins, Angew. Chem., Int. Ed. Engl., 24, 760 (1985).
30.R. Steudel, K. Bergemann, J. Buschmann and P. Luger, Angew. Chem., Int. Ed. Engl., 35, 2537 (1996).
31.R. Steudel, Topics Current Chem., 102, 149 (1982).
32.T. Maaninen, H. M. Tuononen, G. Schatte, R. Suontamo, J. Valkonen, R. Laitinen and T. Chivers, Inorg. Chem., 43, 2097 (2004).
33.T. Maaninen, T. Chivers, R. Laitinen, G. Schatte and M. Nissinen, Inorg. Chem., 39, 5341 (2000).
34.T. Maaninen, T. Chivers, R. Laitinen and E. Wegelius, Chem. Commun., 759 (2000).
35.H. W. Roesky, K-L. Weber and J. W. Bats, Chem. Ber., 117, 2686 (1984).
36.T. Chivers, X. Gao and M. Parvez, J. Am. Chem. Soc., 117, 2359 (1995).
37.A. Maaninen, J. Konu, R. S. Laitinen, T. Chivers, G. Schatte, J. Pietikäinen and M. Ahlgrén, Inorg. Chem., 40, 3529 (2001).
Chapter 7
Metal Complexes
7.1 Introduction
Investigations of the coordination chemistry of simple sulfur–nitrogen ligands have produced a wide variety of complexes.1,2 The first metal complexes, MoCl5.S4N4 and TiCl4.S4N4 were described more than 100 years ago. Although the topic enjoyed some popularity in the 1950s, primarily due to the pioneering work of Becke-Goehring and co-workers, progress was slow. The advent of NMR spectroscopy in the 1960s had little impact on the subject. However, in recent years X-ray crystallography has played a important role in providing key information about the bonding modes and structural parameters of metal complexes. Several aspects of the coordination chemistry of chalcogen–nitrogen ligands warrant attention: (a) the ability of metals to stabilize unstable neutral and anionic binary S–N ligands, (b) the applications of metal complexes as reagents for the preparation of other S–N compounds, (c) comparison of the behaviour of of S–N ligands with valence isoelectronic ligands, e.g., NO, NO3-, and (d) the possible incorporation of metals into sulfur-nitrogen chains to produce conducting materials.
This chapter will begin with a discussion of metal complexes of binary sulfur nitrides. The ring systems S2N2 and S4N4 form typical Lewis acid–Lewis base adducts in which the metal is bonded to the ligand through a nitrogen atom and these have been described in Sections 5.2.4 and 5.2.6, respectively. Consequently, the first section will focus on complexes of the thionitrosyl (NS) ligand. This will be followed by a discussion of complexes formed with binary anionic S–N ligands in
122
Metal Complexes |
123 |
which the metal is part of an S–N ring system. These complexes, known as cyclometallathiazenes, are represented by the general formula MSxNy. The next, short section recounts metal complexes of the monomeric sulfur imide (SNH) ligand, which bonds in a Œ–fashion to metal centres. The final sections compare the behaviour of monomeric thiazyl halides, NSX (X = F, Cl, Br) and the valence isoelectronic thionyl imide [NSO]- and sulfur diimide anions, [NSNR]- and [NSN]2-, as ligands in metal complexes. Although the vast majority of metal–chalcogen–nitrogen complexes involve sulfur as the chalcogen site in the ligand, there is some information about analogous seleniumand tellurium-containing complexes that will be included in the appropriate sections.3,4 A discussion of metal complexes of C-N-E (E = S, Se) heterocycles is included in Sections 11.3.1 and 12.4.1; P-N-E (E = S, Se) ligands are considered in Section 13.2.1. The coordination chemistry of the chalcogen diimides RN=S=NR and tBuNTe(µ-NtBu)2TeNtBu, is covered in Section 10.4.3.
7.2 Thionitrosyl and Selenonitrosyl Complexes
Unlike nitric oxide, NO, the monomeric radical sulfur nitride, NS, is only known as a short-lived intermediate in the gas phase. Nevertheless the properties of this important diatomic molecule have been thoroughly investigated by a variety of spectroscopic and other physical techniques (Section 5.2.1). The NS molecule is stabilized by coordination to a transition metal and a large number of complexes, primarily with metals from Groups 6, 7, 8 and 9, are known. Several detailed reviews of the topic have been published.5-7
A variety of routes is available for the preparation of metal– thionitrosyl complexes. The most common of these are (a) reaction of nitride complexes with a sulfur source, e.g., elemental sulfur, propylene sulfide or sulfur halides, (b) reaction of (NSCl)3 with transition-metal complexes, and (c) reaction of [SN]+ salts with transition-metal complexes. An example of each of these approaches is given in Eq. 7.1, 7.2 and 7.3, respectively. The second method was employed to generate the first thionitrosyl complex.8 Halide abstraction from complexes of thiazyl halides has also been used in a few cases. Surprisingly, the use of
124 |
A Guide to Chalcogen–Nitrogen Chemistry |
S4N4 as a source thionitrosyl-metal complexes is limited to a single example. The first selenonitrosyl-metal complex TpOs(NSe)Cl2 (Tp = hydrotris(1-pyrazolyl)borate) was prepared recently by method (a).9
MoN(S2CNR2)3 + é68 : 0R 16 62CNR2)3 |
(7.1) |
(R = Me, Et)
Na[Cr(CO)3Cp] + • 16&O 3 : &U &2 2Cp(NS) + NaCl + CO (7.2)
[Re(CO)5SO2][AsF6] + [SN][AsF6] :
[Re(CO)5NS][AsF6]2 + SO2 |
(7.3) |
The thionitrosyl ligand is generally coordinated as a terminal, linear unit in which the bond angle <MNS is in the range 169-180°.6 The only known selenonitrosyl–metal complex has a bond angle <MNSe of 165°.9 In contrast to transition-metal nitrosyl complexes, for which both bent and bridging NO ligands are well known, these bonding modes are uncommon for the NS ligand. Complexes containing more than one thionitrosyl ligand, e.g., cis-MCl4(NS)2 (M = Ru, Os), are also rare.10 The characteristic NS stretching vibration in the infrared spectrum is lowered from 1437 cm-1 in the cation [SN]+ to somewhere in the range 1050-1400 cm-1 in metal complexes (cf. 1205 cm-1 in neutral NS). The charge of the complex has a marked influence on the metal–NS back donation as indicated by the trend observed for the isoelectronic half-sandwich complexes of the type [CpM(CO)2MS]n+ (Table 7.1).11
Table 7.1 IR stretching frequencies for [CpM(CO)2]n+
|
16 >FP-1] |
CpCr(CO)2(NS) |
1154 |
[CpMn(CO)2(NS)][AsF6] |
1284 |
[CpFe(CO)2(NS)][AsF6]2 |
1388 |
The nitrogen atom in the (almost) linear metal–thionitrosyl complexes is sp-hybridized and the NS ligand behaves as a three-electron donor. The N–S bond distances in metal complexes vary between 1.45
Metal Complexes |
125 |
and 1.59 Å (cf. 1.44 and 1.495 Å for [SN]+ and NS, respectively). The metal–nitrogen distances are short indicating that, in addition to A, the resonance form B is an important contributor to the overall bonding in these complexes. The N–Se bond length of 1.63 Å in TpOs(NSe)Cl2 is slightly shorter that that in gas phase NSe (1.65 Å).9
M–N+A6- |
< M-=N+=S |
A |
B |
Comparison of the photoelectron spectra and electronic structures of M-NS and M-NO complexes, e.g., [CpCr(CO)2(NX)] (X = S, O), indicates that NS is a better 1-donor and a stronger Œ-acceptor ligand than NO.12 This conclusion is supported by 14N and 95Mo NMR data, and by the UV-visible spectra of molybdenum complexes.13
A few reactions of metal complexes that involve the NS ligand rather than the metal centre have been reported. For example, the reaction of the dication [Re(CO)5(NS)]2+ with cesium halides CsX (X = Cl, Br) converts the NS ligand to a thiazyl halide NSX.14 Oxygen transfer from an NO2 to an NS ligand on the same metal centre to give a thiazate (NSO) complex occurs in ruthenium porphyrin complexes.15 Treatment of an osmium thionitrosyl complex with triphenylphosphine converts the NS ligand to an NPPh3 group with the formation of Ph3PS (Eq. 7.4).16 Mechanistic studies of this transformation indicate that it occurs via the intermediate formation of an NSPPh3 ligand, which reacts rapidly with a second equivalent of PPh3. The NSe ligand is deselenized almost
instantly at room temperature by PPh3.9 |
|
|
[Os(tpm)Cl2(NS)]+ + 2PPh3 : >2V WSP &O2(NPPh3)]+ + SPPh3 |
(7.4) |
|
|
(tpm = tris(pyrazol-1-yl)methane) |
|
7.3 |
Cyclometallathiazenes |
|
7.3.1 |
Introduction |
|
The term cyclometallathiazene refers to ring systems that contain only sulfur, nitrogen and one (or more) metal atoms. As indicated in Table 7.2,

126 |
|
|
|
|
|
|
|
|
|
|
|
|
|
|
|
|
A Guide to Chalcogen–Nitrogen Chemistry |
||||||||
|
|
|
|
|
|
|
|
|
|
|
|
|
|
|
|
|
|
|
|
|
|
Table 7.2 |
Cyclometallathiazenes |
|
|
|
|
|
|
|
|
|
|
|
|
|
|
|
|
|
|
|
|
|
|
|
|
|
|
|
|
|
|
|
|
|
|
|
|
|
|
|
|
|
|
|
|
|
|
|
|
|
|
Corresponding |
Metal |
Year of Discovery |
|
|
|
|
|
|
|
|
|
|
|
|
|
|
|
|
|
|
|
|
|
|
|
S-N anion |
(first X-ray structure) |
||
|
|
|
|
|
|
|
|
|
|
|
|
|
|
|
|
|
|
|
|
|
|
|
|||
|
|
|
|
|
|
|
|
|
|
|
|
|
|
|
|
|
|
||||||||
|
|
|
|
|
|
|
|
|
|
S |
|
|
N |
|
2- |
|
|
||||||||
|
|
|
M |
|
|
|
|
|
|
|
|
Co, Ir, Ni, Pd, Pt (Pb) |
1982 (1982) |
||||||||||||
|
|
|
|
|
|
|
N |
|
|
S |
S2N2 |
||||||||||||||
|
|
|
|
|
|
|
|
|
|
|
|
|
|||||||||||||
|
|
|
|
|
|
|
|
|
|
|
|
|
|
|
|
|
|
||||||||
|
|
|
M N S |
S2N24- |
Re |
1984 (1984) |
|||||||||||||||||||
|
|
|
|
|
|
|
N |
|
|
S |
|
|
|
|
|||||||||||
|
|
|
|
|
|
|
|
|
|
|
|
|
|
|
|
|
|
||||||||
|
|
|
|
|
|
|
|
|
|
H |
|
|
|
|
|
|
|
|
|
|
|
||||
|
|
|
|
|
|
|
|
|
|
N |
|
|
S |
[S N H]- |
Co, Ni, Pd, Pt |
1953 (1958) |
|||||||||
|
|
|
M |
|
N |
2 |
2 |
|
|
||||||||||||||||
|
|
|
|
|
|
|
S |
|
|
|
|
|
|||||||||||||
|
|
|
M S N |
S3N- |
Fe, Ru, Co, Rh, Ni, |
1958 (1966) |
|||||||||||||||||||
|
|
|
|
|
|
|
S |
|
|
S |
|
|
Pd, Pt, Cu, Ag, Au |
|
|||||||||||
|
|
|
|
|
|
|
|
|
|
|
|
|
|
|
|
|
|
||||||||
|
|
|
|
|
|
N |
|
|
|
|
S |
S N |
- |
Pd |
1992 (1992) |
||||||||||
|
|
|
|
|
|
|
|
|
|||||||||||||||||
|
M |
|
|
|
|
|
|
|
|
|
|
|
|
|
N |
||||||||||
|
|
|
|
|
|
|
|
|
|
|
|
|
|
2 |
3 |
|
|
||||||||
|
|
|
|
|
|
N |
|
|
|
|
S |
|
|
|
|
||||||||||
|
|
|
|
|
|
|
|
|
|
|
|
|
|||||||||||||
|
|
|
|
|
|
N |
S |
S2N33- |
|
|
|||||||||||||||
|
M |
|
|
|
|
|
|
|
|
|
|
|
|
|
N |
V, Mo, W |
1983 (1983) |
||||||||
|
|
|
|
|
|
N |
|
|
|
|
S |
|
|
|
|
||||||||||
|
|
|
|
|
|
|
|
|
|
|
|
|
|||||||||||||
|
|
N S N |
S N 2- |
Ti |
1983 (1983) |
||||||||||||||||||||
|
M |
|
|
|
|
|
|
|
|
|
|
|
|
|
|
|
S |
||||||||
|
|
|
|
|
|
|
|
|
|
|
|
|
|
|
|
3 |
4 |
|
|
||||||
|
|
|
N S N |
|
|
|
|
||||||||||||||||||
|
|
|
|
S |
|
|
|
|
|
|
|
|
|
|
|
||||||||||
N |
|
|
|
|
|
|
|
M |
|
|
|
|
S |
|
- |
Pt |
1981 (1981) |
||||||||
|
|
|
|
|
|
|
|
|
|||||||||||||||||
|
S |
|
|
|
|
|
N |
|
|
|
|
|
|
N |
S4N3 |
||||||||||
|
|
|
|
|
|
|
|
|
|
|
|
|
|
|
|
||||||||||
|
|
|
|
|
|
|
|
|
|
|
|
|
|
|
|
S |
|
|
|
|
|||||
|
N |
|
|
|
|
|
M |
|
|
|
|
N |
SN2 |
2- |
Hg, Zr, Hf |
1904 (1984) |
|||||||||
|
|
|
|
|
|
|
|||||||||||||||||||
S |
|
|
|
|
|
|
|
|
|
|
|
|
|
|
|
S |
|||||||||
|
|
|
|
|
|
|
|
|
|
|
|
|
|
|
|
||||||||||
|
|
|
|
|
|
|
|
|
|
|
|
|
|
|
|
|
|||||||||
|
N |
|
|
|
|
M |
|
|
|
|
N |
|
|
|
|
||||||||||
|
|
|
|
|
|
|
|
|
|
|
|
||||||||||||||
M |
|
|
S |
|
|
N |
S N |
2- |
Pd |
1966 (1982) |
|||||||||||||||
|
|
|
|
||||||||||||||||||||||
|
|
|
|
|
|
|
|
|
|
|
|
|
|
|
|
|
|
|
S |
||||||
|
|
|
|
|
|
|
|
|
|
|
|
|
|
|
|
|
|
|
3 |
2 |
|
|
|||
M |
|
|
S |
|
|
N |
|
|
|
|
|||||||||||||||
|
|
|
|
|
|
|
|
||||||||||||||||||
N |
S |
|
|
|
|
|
|
|
S4N42- |
Ir, Pt |
1970 (1986) |
||||||||||||||
|
|
|
|
|
|
|
|
|
|
|
|
|
S |
||||||||||||
S N N N |
|
|
|
|
|||||||||||||||||||||
|
|
|
M |
|
|
|
|
|
|
|
S |
|
|
|
|
||||||||||
|
|
|
|
|
|
|
|
|
|
|
|
|
|
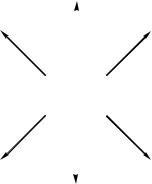
Metal Complexes |
127 |
these heterocycles can formally be considered as complexes between cationic metal fragments and binary S–N anions in which, with the exception of the [S2N2H]- and [S4N4]2- ions, both the sulfur and nitrogen atoms are two-coordinate. Table 1 indicates which metals are known to form complexes with individual S–N anions, as well as the year of discovery and first X-ray structure for each type of cyclometallathiazene.
The unknown selenium–nitrogen anions [Se3N]-, [Se2N2]2- and [Se2N2H]- have also been stabilized by coordination to platinum.17-19 A few examples of complexes involving anionic
ligands with two different chalcogens, e.g., [ESN2]2- and [ESN2H]- (E = Se, Te), are known.20, 21
Since only two of these chalcogen-nitrogen anions, [S3N]- (Section 5.4.2) and [SN2]2- (Section 5.4.1), have been isolated as ionic salts, the synthesis of cyclometallathiazenes usually employs S4N4 (or other S–N ring systems) as a source of the S–N fragment. Some representative examples of these redox transformations are shown in Scheme 7.1. The
explosive regent Se4N4 has also been used to generate complexes of the [Se3N]- and [Se2N2H]- anions.17, 19 A safer route for the in situ generation
of the anions [Se2N2]2- and [Se2N2H]- involves the use of SeCl4 or, preferably, SeOCl2, in liquid ammonia.18
|
|
CpCo(S2N2) |
|
|
|
|
|
|
IrCl(CO)(PPh3)(S4N4) |
|
CpCo(CO)2 |
[Cl2V(S2N3)]2 |
|||||
|
||||||||
|
|
|
|
|
|
|
|
|
IrCl(CO)(PPh3)2 |
|
VCl4 |
|
|
|
|
||
|
|
S4N4 |
|
|
|
|
|
|
(PhCN)2PtCl2 |
|
Pd |
Cl |
2- |
|
|
|
|
|
|
|
|
|||||
|
|
|
2 |
|
6 |
|
|
|
|
|
|
Cp2Ti(CO)2 |
|
|
|
|
|
(S N )PtCl |
|
|
Pd Cl (S N )2- |
|||||
|
|
|||||||
4 |
3 |
|
|
|
2 |
4 |
3 |
2 |
Cp2TiS3N4
Scheme 7.1 Preparation of cyclometallathiazenes from S4N4
128 |
A Guide to Chalcogen–Nitrogen Chemistry |
7.3.2 Complexes of the [E2N2H]- and [E2N2]2- anions (E = S, Se)
Complexes containing the [S2N2H]- ion comprise the best studied group of cyclometallathiazenes. The dark green nickel(II) derivative is readily obtained in good yields from the reaction of S4N4 with anhydrous NiCl2 in methanol.22 The individual MS2N2H rings in this spirocyclic complex are planar. The platinum(II) complexes [Pt(S2N2H)(PR3)]X (X = halide) (and their selenium analogues) are especially interesting since they form continuous stacking structures.23 The NH groups in M(S2N2H)2 complexes are readily deprotonated to give monoor di-anions,24a which can be converted to organic derivatives by treatment with alkyl halides.2 An interesting application of these dianionic metal complexes involves the preparation of the trinuclear complex [Ni3(N2S2)4]2- (7.1) by the reaction of two equivalents of [Ni(N2S2)2]2- with NiCl2.24b
In addition to S4N4, a variety of S-N reagents, including [S3N3]-, S4N4H4/diazobicycloundecene, [R2Sn(S2N2)]2 (R = Me, nBu), and solutions of [S4N3]Cl in liquid ammonia, provide a source of [S2N2]2- for metal complexes. Although X-ray structural data for MS2N2 complexes indicate a partial localization of Œ-bonding within the –N=S=N– unit, delocalization over the entire ring system has been inferred for Cp2CoS2N2 on the basis of molecular orbital calculations.25 This cyclometallathiazene undergoes an electrochemically reversible oneelectron reduction; the monoanion [Cp2CoS2N2]- can also be generated by chemical reduction with cobaltocene.26
The MS2N2 complexes and their selenium analogues are readily protonated at the nitrogen attached to the metal (Eq. 7.5). Competitive studies show that the selenium complex is a stronger base than its sulfur analogue.
Pt(E2N2)(PR3)2 + HBF4 : >3W (2N2H)(PR3)2]BF4 |
(7.5) |
(E = S, Se)
Differences are also observed in the reactivity of platinum(II) complexes of [S2N2]2- and [Se2N2]2- towards halogens. In the former case oxidative addition to give platinum(IV) complexes occurs, with retention

Metal Complexes |
129 |
of the S–N ligand. By contrast, the Se–N ligand is removed in the form of Se4N4.
7.3.3 Complexes of the [E3N]- anion (E = S, Se)
Complexes of the [S3N]- anion with a wide variety of transition metals are known (see Table 7.2).6 Although S4N4 is not an efficient reagent for the production of these complexes, the first representative, Ni(S3N)2 was obtained in a minuscule yield (9 mg, 0.04%) from the reaction of NiCl2 and S4N4 in methanol and correctly identified on the basis of analytical data.27 Such complexes are now obtained in good yields via deprotonation of cyclo-S7NH in the presence of metal halides or by using mercury(II) complexes Hg(S7N)2 or PhHg(S7N)2 as a source of [S3N]-. The [SSNS]- ligand is invariably chelated to the metal via the two terminal sulfur atoms.28 The formation of the trigonal bipyramidal anion {[Ni(S3N)]3S2}- (7.2) by the former process is of particular interest.29 In the anionic copper(I) complex [Cu(S3N)(S7N)]- (7.3) an S7N ring is preserved and coordinated to the metal through the nitrogen atom.30
|
|
|
|
|
|
|
|
|
- |
|
|
|
|
2- |
|
|
|
S |
N |
N |
S |
S |
N |
|
|
|
S |
||
|
|
|
S |
|
|||||
|
|
|
|
|
|
|
|
||
|
|
|
|
|
|
|
Ni |
|
|
S |
N |
N |
S |
|
|
N |
S |
S |
|
|
Ni |
Ni |
Ni |
|
|
S |
Ni |
|
S |
S |
N |
N |
S |
|
|
|
|||
|
|
|
S |
Ni |
N |
||||
|
|
|
|
|
|
|
|||
|
|
|
|
|
|
|
|
||
N |
S |
S |
N |
|
|
|
S |
S |
S |
|
|
|
|
|
|
|
|
||
|
|
7.1 |
|
|
|
|
|
7.2 |
|
|
|
S |
S |
|
|
|
|
- |
|
|
|
|
|
|
S |
|
|
||
|
|
|
N |
|
S |
|
|
||
|
|
|
Cu |
S |
|
|
|||
|
|
N |
|
|
S |
S |
|
|
|
|
|
S |
|
S |
S |
|
|
||
|
|
|
|
|
|
|
|||
|
|
|
|
|
7.3 |
|
|
|
|
130 |
A Guide to Chalcogen–Nitrogen Chemistry |
Cyclic selenium imides, e.g., Se7NH, are not available as a source of Se–N anions (Section 6.3). The only example of a metal complex of the
[Se3N]- anion, Pt(Se3N)Cl(PMe2Ph), was obtained by the reaction of Se4N4 with [PtCl2(PMe2Ph)]2.17
7.3.4 Complexes of the [S2N3]3- anion
The trianionic chelating ligand [S2N3]3- stabilizes early transition metals in high oxidation states. The known complexes are limited to V(V), Mo(VI) and W(VI). The MS2N3 ring, e.g., [VCl2(S2N3)]\,31 is generated in reactions of high oxidation state early transition-metal halides with S4N4, (NSCl)3 or [S3N2Cl]Cl. The thiazene segment of the ring is usually planar but, in some cases, the metal is tilted out of this plane. The electronic structures of six-membered cyclometallathiazenes (MS2N3) have been investigated by density functional theory calculations.32 For anions of the type [Cl4MS2N3]- (M = Mo, W), in which a sulfur atom in [S3N3]- is formally replaced by the MCl4 group, the HOMO-LUMO gap is predicted to be small. The closed shell (singlet) state is preferred over the open shell (triplet) state by only ca. 7 kcal mol-1. The HOMO is derived from the combination of an S–N antibonding (Œ*) orbital and the dxy orbital of the transition metal. Charge transfer from the ligand to the M(VI) centre results in a strengthening of the S–N bonds compared to those in [S3N3]-.
Complexes of the monoanion [S2N3]- can be obtained by the reactions of [PPh4][Pd2Cl6] with S5N633a or, preferably, Me3SiNSNSNSNSiMe3.33b
7.3.5 Complexes of the [S3N4]2-, [S4N3]- and [S4N4]2- anions
The only example of the eight-membered MS3N4 ring system, in which one of the sulfur atoms in S4N4 is replaced by a transition metal, is Cp2TiS3N4 obtained in low yield by reaction with Cp2Ti(CO)2 (Scheme 7.1).34 There is also only a single representative of the MS4N3 ring system, ClPtS4N3, in which the hypothetical [S4N3]- ligand acts as a tridentate (S,N,S') ligand.35
Coordination of the dianion [S4N4]2- as a tridentate ligand has been established in a few cases. The complexes IrCl(CO)(S4N4)PPh336 and