
Chivers T. - A Guide to Chalcogen-Nitrogen Chemistry (2005)(en)
.pdf
Formation of Chalcogen–Nitrogen Bonds |
21 |
In a related cyclocondensation six-membered rings containing the NSeN functionality may be prepared by the reaction of imidoylamidines with SeCl4 (Eq. 2.10).26
Ph |
|
Ph |
|
|
NH2 |
SeCl4, Cl2 |
|
N |
Cl |
|
|
|
||
N |
120 oC |
N |
Se |
(2.10) |
NH.HCl |
|
N |
|
|
|
|
|
||
Ph |
|
Ph |
|
|
The cyclocondensation route is also effective for the synthesis of planar C2N4S2 rings from benzamidines and sulfur dichloride in the presence of a strong base (Eq. 2.11).27
PhC(NH)(NH2) + 3SCl2 + 6DBU : 3K& 161 2CPh + 6DBU.HCl
(DBU = diazabicycloundecane) |
+ 1/8S8 |
(2.11) |
Tellurium(VI)–nitrogen bonds can be generated by the reaction of hexamethyldisilazane with tellurium hexafluoride (Eq. 2.12).28 The product (Me3SiNH)TeF5 is a useful precursor for a variety of NTeF5 compounds. By contrast, SF6 is inert towards Si–N reagents.
(Me3Si)2NH + TeF6 : 0H3SiNH)TeF5 + Me3SiF (2.12)
2.3 From Azides
Reactions of ionic or covalent azides with chalcogen halides or, in the case of sulfur, with the elemental chalcogen provide an alternative route to certain chalcogen–nitrogen compounds. For example, the reaction of sodium azide with cyclo-S8 in hexamethylphosphoric triamide is a more convenient synthesis of S7NH than the S2Cl2 reaction (Section 6.2.1).29 Moreover, the azide route can be used for the preparation of 50% 15N- enriched S7NH.
The polymer (SN)x may also be made in a two-step process using azide reagents. Thus the reaction of S2Cl2 with sodium azide in
22 |
A Guide to Chalcogen–Nitrogen Chemistry |
acetonitrile followed by treatment of the [S3N2]Cl produced with Me3SiN3 yields (SN)x as a black solid.30 By contrast, the explosive and insoluble black compound Se3N2Cl2, which probably contains the [Se3N2Cl]+ cation, is prepared by the treatment of Se2Cl2 with trimethylsilyl azide in CH2Cl2 (Eq. 2.13).31
3Se2Cl2 + 2Me3SiN3 : 6H3N2Cl2 + 2Me3SiCl + N2 (2.13)
Chalcogen-nitrogen cations can be generated by the reactions of homopolyatomic chalcogen cations with azides. For example, the reaction of S82+ with sodium azide in liquid SO2 is a source of [NS2+].32 Remarkably, the reaction of Te42+ with potassium azide in SO2 gives the tris(azido)tellurium(IV) cation [Te(N3)3][SbF6] as a stable salt.33
Organosulfur(II) azides evolve N2 spontaneously at room temperature. For example, the reaction of CF3SCl with Me3SiN3 produces the, presumably cyclic, tetramer (CF3SN)4.34 Covalent arylselenium azides may be generated from arylselenium(II) halides and Me3SiN3 and used as in situ reagents, but they decompose to the corresponding diselenides ArSeSeAr at room temperature. A notable exception is the derivative 2-Me2NCH2C6H4SeN3, prepared by
using AgN3 instead of Me3SiN3, which is stabilized by intramolecular N:6H FRRUGLQDWLRQ 6HFWLRQ 35 In contrast to the thermal instability
of covalent organoselenium azides, a wide range of stable dialkyltellurium(IV) diazides and alkyl or aryl-tellurium(IV) triazides have been prepared by the metathesis of the corresponding fluorides with
Me3SiN3 in CH2Cl2 (Eq. 2.14).36a The binary tellurium(IV) azide Te(N3)4 is likewise obtained by metathesis of TeF4 (in CFCl3)36b or TeF6 (in
CH3CN)36c with trimethylsilyl azide (Section 5.2.9).
R2TeF2 + 2Me3SiN3 : 52Te(N3)2 + 2Me3SiF |
(2.14) |
(R = Me, Et, nPr, iPr, Cy) |
|
The cyclocondensation of trimethylsilyl azide with a bis(sulfenyl chloride) is an efficient synthesis of dithiazolium cations (Section 11.3.5) (Eq. 2.15).37

|
|
Formation of Chalcogen–Nitrogen Bonds |
23 |
|||||
SCl |
|
|
|
|
S |
|
|
|
|
Me3SiN3 |
|
|
- |
|
|||
|
|
+ |
N Cl |
(2.15) |
||||
|
|
|
|
|
+ Me3SiCl + N2 |
|||
|
|
|
|
|
SCl |
S |
|
The use of azide reagents is also important for the synthesis of cyclic sulfur(VI)–nitrogen systems. The reaction of SOCl2 with sodium azide in acetonitrile at –35°C provides a convenient preparation of the trimeric sulfanuric chloride [NS(O)Cl]3 (Eq. 2.16).38a Thionyl azide, SO(N3)2 is generated by the heterogeneous reaction of thionyl chloride vapour with silver azide (Eq. 2.17).38b This thermally unstable gas was characterized in situ by photoelectron spectroscopy. The phenyl derivative of the sixmembered ring [NS(O)Ph]3 can be prepared from lithium azide and PhS(O)Cl.39
3NaN3 + 3SOCl2 : >16 2 &O@3 + 3NaCl |
(2.16) |
2AgN3 + SOCl2 : 62 13)2 + 2AgCl |
(2.17) |
2.4 From Silicon–Nitrogen or Tin–Nitrogen Reagents
Condensation reactions involving the combination of chalcogen halides and reagents with one or more Si–N functionalities represent a very versatile approach to a wide range of cyclic and acyclic chalcogen– nitrogen compounds. The driving force for these reactions is normally the formation of volatile Me3SiCl , which is easily separated from the other reaction products.
The outcome of these reactions is often markedly dependent on the chalcogen. For example, the monofunctional iminophosphorane reagent Ph3P=NSiMe3 reacts with SCl2, in a 2:1 molar ratio, to give the sulfonium salt [S(NPPh3)3]Cl, presumably via a disproportionation process.40 By contrast, both mono and bis-N=PPh3 substituted derivatives
are obtained by the reaction of this reagent with ECl4 (E = Se, Te) (Eq. 2.18).41, 42
nPh3PNSiMe3 + ECl4 : 3K3PN)nECl4-n + nMe3SiCl (2.18) (n= 1, 2)
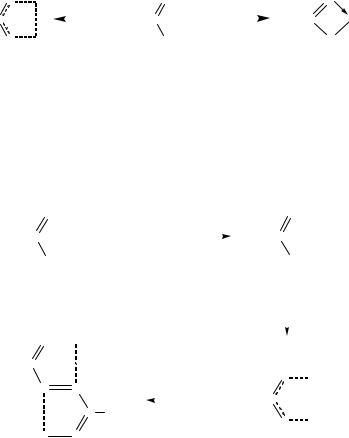
24 |
A Guide to Chalcogen–Nitrogen Chemistry |
The reactions of trifunctional Si–N reagents with chalcogen halides are especially fruitful. Trisilylated benzamidines ArCN2(SiMe)3 are very versatile reagents for the introduction of a carbon atom into a chalcogen– nitrogen ring system. The reactions of these reagents with SCl2 or SeCl2 (generated in situ) give rise to the five-membered cyclic cations [ArCN2E2]+ (E = S, Se) (Section 11.3.1)44 (cf. the formation of eightmembered C2N4S2 rings from the reactions of benzamidines with SCl2) (Section 12.4.1). In the reaction with TeCl4 only one mole of Me3SiCl is eliminated to give a four-membered CN2Te ring (Scheme 2.2).45
|
|
N |
|
E |
|
|
|
NSiMe3 |
|
|
|
|
SiMe3 |
||
|
|
|
|
|
|
|
|
|
|
N |
|||||
|
|
|
|
|
|
|
|
|
|
||||||
|
|
|
+ |
|
|
2 ECl2 |
TeCl4 |
TeCl3 |
|||||||
Ar |
|
C |
|
|
|
Ar |
|
C |
|
|
Ar |
|
C |
||
|
|
|
|
|
|
|
|
||||||||
|
|
|
|
|
|
E = S, Se |
|
|
|
|
N |
||||
|
|
N |
|
E |
|
|
|
N(SiMe3)2 |
|
|
|
|
|||
|
|
|
|
|
|
|
|
|
|
||||||
|
|
|
|
|
|
|
|
|
|
|
|
|
|
|
SiMe3 |
Scheme 2.2 Reactions of trisilylated benzamidines with chalcogen halides
The reactions of trisilylated benzamidines with benzenechalcogenyl halides are of particular interest. The initially formed trisubstituted derivatives are thermally unstable and decompose by a radical process to generate the dark blue diazenes trans-PhEN(Ph)CN=NC(Ph)NEPh (E = S, Se) (Scheme 2.3) (Section 15.2).46
|
|
|
|
NSiMe3 |
|
|
|
|
|
|
|
|
|
|
|
NEPh |
|
|
|||
Ph |
|
|
C |
|
|
+ 3 PhECl |
|
|
|
|
Ph |
|
|
C |
N(EPh)2 |
|
|||||
|
|
|
|
|
|
|
|
|
|||||||||||||
|
|
|
|
N(SiMe3)2 |
(E = S, Se) |
|
|
|
|
|
|
|
|
||||||||
|
|
|
|
|
|
|
|
|
|
|
|
|
|
|
|
|
|
|
|
|
|
|
|
|
|
|
|
|
|
|
|
|
|
|
|
|
|
|
|
- 1/2 Ph2E2 |
|||
|
|
|
|
|
|
|
|
|
|
|
|
|
|
|
|
|
|
||||
|
|
|
|
N |
|
EPh |
|
|
|
|
|
|
|
|
|
|
|
|
|
|
|
|
|
|
|
|
|
|
|
|
|
|
|
|
|
|
|
|
|
|
|
||
|
|
|
|
|
|
|
|
|
|
|
|
|
|
|
|
|
|
|
|
||
Ph |
|
|
C |
|
|
|
|
|
|
|
|
|
|
|
|
N |
|
EPh |
|||
|
|
|
|
|
|
|
|
|
|
|
|
|
|
|
|||||||
|
|
|
|
N |
|
N |
|
- 1/2 Ph2E2 |
|
|
|
|
|
|
|
||||||
|
|
|
|
|
|
|
|
|
|
|
|
. |
|||||||||
|
|
|
|
|
|
Ph |
|
|
C |
|
|
|
|||||||||
|
|
|
|
|
|
|
|
|
|
|
|
|
|||||||||
|
|
|
|
|
|
|
|
|
|
|
|
|
|
|
|
|
|||||
|
|
|
|
|
|
C |
Ph |
|
|
|
EPh |
||||||||||
|
|
|
|
|
|
|
|
|
|
|
|
N |
|
||||||||
|
|
|
PhE |
|
N |
|
|
|
|
|
|
|
|
|
|
|
|||||
|
|
|
|
|
|
|
|
|
|
|
|
|
|
|
|||||||
|
|
|
|
|
|
|
|
|
|
|
|
|
|
|
|
|
|
|
Scheme 2.3 Reactions of trisilylated benzamidines with benzenechalcogenyl halides

Formation of Chalcogen–Nitrogen Bonds |
25 |
Phosphorus(V) can also be introduced into chalcogen–nitrogen rings via cyclocondensation reactions using R2PN2(SiMe)3. In this case eightmembered rings of the type R2P(NEN)2PR2 (E = S, Se) with folded
structures (a cross-ring E•••E interaction) are formed. The reagents of choice are SOCl2 (E = S)47 or a mixture of SeCl4 and Se2Cl2 (E = Se)
(Section 13.2.1).48
In the case of tellurium four-membered PN2Te rings of the type Ph2P( -NSiMe3)2Te=NP(Ph2)NSiMe3 are obtained from the reaction of TeCl4 with Li[Ph2P(NSiMe3)2] (Eq. 2.19).49
|
SiMe3 |
SiMe3 |
|
|
|||||
|
N |
N |
|
|
|
+ 2 LiCl |
|
||
2 Ph2P |
Li |
TeCl4 |
Ph2P |
Te |
|
NP(Ph2)NSiMe3 |
(2.19) |
||
|
|
|
+ Me3SiCl |
||||||
|
N |
N |
Cl |
|
|||||
|
SiMe3 |
SiMe3 |
|
|
The trifunctional amines N(MMe3)3 (M = Si, Sn) are attractive alternatives to ammonia for the generation of chalcogen–nitrogen bonds by reaction with chalcogen halides. The use of (Me3Sn)3N instead of ammonia allows kinetic control of the reaction with S2Cl2 and results in the formation of a five-membered metallathiazene ring (Eq. 2.20),15 which can be converted into other S–N ring systems by metathesis with element halides (Section 7.3.2).
4S2Cl2 + 4(Me3Sn)3N : 0H2SnS2N2)2 + 2Me4Sn +
8Me3SnCl + 1/2S8 |
(2.20) |
Acyclic Se–N chlorides that are potential building blocks (as a source of the SeNSe unit) for cyclic Se–N systems are generated by the reactions of [SeCl3][AsF6] or SeCl4 with (Me3Si)3N as illustrated by the sequence of transformations that produce the five-membered [Se3N2Cl]+ cation (Scheme 2.4 and Section 8.4). 50-52

26 |
A Guide to Chalcogen–Nitrogen Chemistry |
|
|||||||||
|
SeCl4 |
+ |
N(SiMe)3 |
|
(i) |
Se2NCl3 |
|
||||
|
|
|
|
|
|
||||||
|
|
|
|
|
|
||||||
|
|
|
|
|
|
|
|
|
|
(ii) |
|
|
|
|
|
|
|
|
|
|
|
|
|
|
|
|
|
|
(iii) |
|
|
|
|
||
|
[Se3N2Cl][GaCl4] |
[N(SeCl)2][GaCl4] |
|
||||||||
|
|
|
|
|
|||||||
|
|
|
|
|
|||||||
Scheme |
2.4 Formation |
of |
acyclic and |
cyclic |
Se–N |
cations: (i) –3Me3SiCl, |
–Cl2 |
||||
(ii) GaCl3 (iii) Ph3Sb |
|
|
|
|
|
|
|
|
|
|
|
The |
major product |
obtained |
from |
the |
reaction of TeCl4 |
with |
(Me3Si)3N is determined by the stoichiometry. When approximately equimolar amounts of the two reagents in THF are used the dimeric cluster Te6N8 (stabilized by coordination to four TeCl4 molecules) is obtained in high yields (Section 5.2.8).53 When the same reaction is carried out in acetonitrile with a molar ratio of 2:1, followed by treatment of the product with AsF5 in SO2, [Te4N2Cl8][AsF6]2 is obtained Section 8.5).54 The dication [Te4N2Cl8]2+ in this salt is the dimer of the hypothetical tellurium(IV) imide [Cl3Te–N=TeCl]+.
References
1.H. G. Heal, The Inorganic Heterocyclic Chemistry of Sulfur, Nitrogen and Phosphorus, Academic Press, London (1980).
2.T. Chivers, Chem. Rev., 85, 341 (1985).
3.R. T. Oakley, Prog. Inorg. Chem., 36, 1 (1988).
4.T. Chivers, Formation of S–N, Se–N and Te–N Bonds, in J. J. Zuckerman and A. P. Hagen (ed.) “Inorganic Reactions and Methods”, Vol. 5. VCH Publishers, Inc., New York, pp. 57-74 (1991).
5.T. Chivers, Sulfur–Nitrogen Compounds, in R. B. King (ed.) Encyclopedia of Inorganic Chemistry, Vol.7, John Wiley, pp. 3988-4009 (1994).
6.T. Torroba, J. Prakt. Chem., 341, 99 (1999).
7.S. Apler, M. Carruthers and L. H. Sutcliffe, Inorg. Chim. Acta, 31, L455 (1978).
8.P. K. Gowik and T. M. Klapötke, Spectrochim. Acta, 46A, 1371 (1990).
Formation of Chalcogen–Nitrogen Bonds |
27 |
9.(a) W. L. Jolly and K. D. Maguire, Inorg. Synth., 9, 102 (1967); (b) T. Chivers,
R.T. Oakley, O. J. Scherer and G. Wolmershäuser, Inorg. Chem., 20, 914 (1981).
10.H. G. Heal and J. Kane, Inorg. Synth., 11, 192 (1968).
11.A. Maaninen, J. Siivari, R. S. Laitinen and T. Chivers, Inorg. Synth., 33, 196 (2002).
12.M. Björgvinsson, H. W. Roesky, F. Pauer, D. Stalke and G. M. Sheldrick, Inorg. Chem., 29, 5140 (1990).
13.J. Siivari, T. Chivers and R. S. Laitinen, Inorg. Chem., 32, 1519 (1993).
14.T. Murai, K. Nonomura, K. Kimura and S. Kato, Organometallics, 10, 1095 (1991).
15.C. P. Warrens and J. D. Woollins, Inorg. Synth., 25, 43 (1989).
16.F. Fockenberg and A. Haas, Z. Naturforsch., 41B, 413 (1986).
17.T. Chivers, N. Sandblom and G. Schatte, Inorg. Synth., 34, 42 (2004).
18.R. E. Allan, H. Gornitzka, J. Karcher, M. A. Paver, M-A. Rennie, C. A. Russell,
P.R. Raithby, D. Stalke, A. Steiner and D. S. Wright, J. Chem. Soc., Dalton Trans., 1727 (1996).
19.O. J. Scherer and G. Wolmershäuser, Z. Anorg. Allg. Chem., 432, 173 (1977).
20.R. Bussas, G. Kresze, H. Münsterer and A. Schwöbel, Sulfur Rep., 2, 215 (1983).
21.T. Maaninen, R. S. Laitinen and T. Chivers, Chem. Commun., 1812 (2002).
22.Y. Inagakin, T. Hosogai, R. Okazaki and N. Inamoto, Bull. Chem. Soc. Jpn., 53, 205 (1980).
23.A. Maaninen, T. Chivers, M. Parvez, J. Pietikäinen and R. S. Laitinen, Inorg. Chem.,
38, 4093 (1999).
24.T. Maaninen, T. Chivers, R. S. Laitinen, G. Schatte and M. Nissinen, Inorg. Chem.,
39, 5241 (2000).
25.V. N. Kovtonyk, A. Yu. Makarov, M. M. Shakirov and A. V. Zibarev, Chem. Commun., 1991 (1996).
26.R. T. Oakley, R. W. Reed, A. W. Cordes, S. L. Craig and J. B. Graham, J. Am Chem. Soc., 109, 7745 (1987).
27.I. Ernest, W. Holick, G. Rihs, D. Schomburg, G. Shoham, D. Wenkert and R. B. Woodward, J. Am. Chem. Soc., 103, 1540 (1981).
28 |
A Guide to Chalcogen–Nitrogen Chemistry |
28.K. Seppelt, Inorg. Chem., 12, 2837 (1973).
29.J. Bojes, T. Chivers and I. Drummond, Inorg. Synth., 18, 203 (1978).
30.F. A. Kennett, G. K. MacLean, J. Passmore and M. N. S. Rao, J. Chem. Soc., Dalton Trans., 851 (1982).
31.J. Siivari, T. Chivers and R. S. Laitinen, Angew. Chem., Int. Ed., 31, 1518 (1992).
32.A. J. Banister, R. G. Hey, G. K. MacLean and J. Passmore, Inorg. Chem., 21, 1679 (1982).
33.J. P. Johnson, G. K. MacLean, J. Passmore and P.S. White, Can. J. Chem., 67, 1687 (1989).
34.D. Bielefeld and A. Haas, Chem. Ber., 116, 1257 (1983).
35.T. M. Klapötke, B. Krumm and K. Polborn, J. Am. Chem. Soc., 126, 710 (2004).
36.(a) T. M. Klapötke, B. Krumm, P. Mayer, H. Piotrowski, O. P Ruscitti and
A. Schiller, Inorg. Chem., |
41, 1184 (2002); (b) T. M. Klapötke, B. Krumm, |
P. Mayer and I. Schwab, |
Angew. Chem., Int. Ed. Engl., 42, 5843 (2003); |
(c) R. Hiages, J. A. Boatz, |
A. Vij, M. Gerken, S. Schneider, T. Schroer and |
K.O. Christe, Angew. Chem., Int. Ed. Engl., 42, 5847 (2003).
37.G. Wolmershäuser, M. Schnauber and T. Wilhelm, J. Chem. Soc., Chem. Commun., 573 (1984).
38.(a) H. Klüver and O. Glemser, Z. Naturforsch., 32B, 1209 (1977); (b) Z. Xiaoqing,
L.Fengyi, S. Qiao, G. Maofa, Z. Jianping, A. Xicheng, M. Lingpeng, Z. Shijun and
W.Dianxun, Inorg. Chem., 43, 4799 (2004).
39.T. J. Maricich, J. Am. Chem. Soc., 90, 7179 (1968).
40.J. Bojes, T. Chivers, A. W. Cordes, G. K. MacLean and R. T. Oakley, Inorg. Chem.,
20, 16 (1981).
41.H. W. Roesky, K. L. Weber, U. Seseke, W. Pinkert, M. Noltemeyer, W. Clegg and
G.M. Sheldrick, J. Chem. Soc., Dalton Trans., 565 (1985).
42.(a) J. Münzenberg, M. Noltemeyer and H. W. Roesky, Chem. Ber., 122, 1915
(1989); (b) H. W. Roesky, J. Münzenberg, R. Bohra and M. Noltemeyer,
J. Organomet. Chem., 418, 339 (1991).
Formation of Chalcogen–Nitrogen Bonds |
29 |
43.H-U. Höfs, J. W. Bats, R. Gleiter, G. Hartman, R. Mews, M. Eckert-0DNVLü
H.Oberhammer and G. M. Sheldrick, Chem. Ber., 118, 3781 (1985).
44.P. Del Bel Belluz, A. W. Cordes, E. M. Kristof, P. V. Kristof, S. W. Liblong and
R.T. Oakley, J. Am. Chem. Soc., 111, 6147 (1989).
45.E. Hey, C. Ergezinger and K. Dehnicke, Z. Naturforsch., 44B, 205 (1989).
46.(a) V. Chandrasekhar, T. Chivers, J. F. Fait and S. S. Kumaravel, J. Am. Chem. Soc.,
112, 5373 (1990); (b) V. Chandrasekhar, T. Chivers, S. S. Kumaravel, M. Parvez and M. N. S. Rao, Inorg. Chem., 30, 4125 (1991).
47.T. Chivers, D. D. Doxsee and R. W. Hilts, Preparation and Some Reactions of the Folded P2N4S2 Ring, in J. D. Woollins (ed.) Inorganic Experiments, 2nd Edn., WileyVCH, pp. 287-290 (2003).
48.T. Chivers, D. D. Doxsee and J. F. Fait, J. Chem. Soc., Chem. Commun., 1703 (1989).
49.T. Chivers, D. D. Doxsee, X. Gao and M. Parvez, Inorg. Chem., 33, 5678 (1994).
50.M. Broschag, T. M. Klapötke, I. C. Tornieporth-Oetting and P. S. White, J. Chem. Soc., Chem. Commun., 1390 (1992).
51.R. Wollert, A. H. Ilwarth, G. Frenking, D. Fenske, H. Goesman and K. Dehnicke,
Angew. Chem., Int. Ed. Engl., 31, 1251 (1992).
52.R. Wollert, B. Neumüller and K. Dehnicke, Z. Anorg. Allg. Chem., 616, 191 (1992).
53.W. Massa, C. Lau, M. Möhlen, B. Neumüller and K. Dehnicke, Angew., Chem., Int. Ed. Engl., 37, 2840 (1998).
54.J. Passmore, G. Schatte and T. S. Cameron, J. Chem. Soc., Chem. Commun., 2311 (1995).
Chapter 3
Applications of Physical Methods
This chapter will provide an overview, illustrated with recent examples, of some applications of the most commonly used physical methods for the characterization of chalcogen–nitrogen compounds.
3.1 Diffraction Techniques
The structures of chalcogen–nitrogen compounds are frequently unpredictable. For example, the reactions of heterocyclic systems often result in substantial reorganization of their structural frameworks, e.g. ring expansion or contraction. The formation of acyclic products from ring systems (or vice versa) is also observed.
Spectroscopic techniques alone are rarely sufficient to provide decisive structural information. Consequently, the ability to establish atomic arrangements in the solid state by X-ray crystallography has been of paramount importance in the development of chalcogen-nitrogen chemistry. Although there are no reviews dedicated exclusively to structural studies of S–N compounds, an account that focuses on the structures of compounds containing Se–N or Te–N bonds was published in 1991.1 Since that time, the amount of structural information that is available for chalcogen–nitrogen compounds has increased dramatically, especially with the advent of CCD diffractometers that allow structure determinations to be completed in less than one day in many cases. X-ray crystallography also provides the details of intermolecular interactions, which are often significant in chalcogen–nitrogen compounds because of the polarity of the E–N bonds. In such compounds structural information is vitally important for understanding
30