
Chivers T. - A Guide to Chalcogen-Nitrogen Chemistry (2005)(en)
.pdf
Chalcogen–Nitrogen Oxides |
171 |
6RNSO + 12LiNHtBu : ^/L2[OS(NtBu)(NR)]}6 + 6H2NtBu |
(9.12) |
(R = tBu, SiMe3) |
|
The corresponding reaction of SO2 with lithium derivatives of primary amines yields dilithium derivatives of the azasulfite anions [O2SNR]2- (9.13) that have not been structurally characterized.43 The reaction of hexameric magnesium anilide [(thf)MgNPh]6 with SO2 results in twelvefold insertion of SO2 into the Mg–N bonds to form a forty-eight atom (Mg6S12N6O24) cluster that contains the azadisulfite dianion [O2S( -NPh)SO2]2- (9.14), which acts as a bis-chelating ligand towards the Mg2+ centres.44
|
2- |
|
|
2- |
|
Ph |
|
|
|
|
O |
|
O |
|
|
|
|
||
|
|
|
|
N |
|
|
|||
|
|
|
|
|
|
|
|
||
|
S |
|
S |
|
S |
|
S |
|
|
|
|
|
- |
|
- |
O |
|||
RN |
NR' |
RN |
O |
O |
O O |
||||
|
|
||||||||
|
9.12 |
|
9.13 |
|
|
9.14 |
|
|
9.7 S-Nitrosothiols RSNO
S-Nitrosothiols RSNO are structural isomers of thionyl imines RNSO. The formal reversal of the order of the S and N atoms in these organic derivatives of ternary S/N/O anions results in completely different properties. S-Nitrosothiols, several of which occur naturally, e.g., S- nitrosocysteine and S-nitrosoglutathione, have been the subject of intense investigation because of their important role in NO transport and regulation in biological systems. Potential applications of RSNO compounds under scrutiny include their use as vasodilators in the treatment of angina, and in the search for a cure for male impotence.2
The most convenient route to S-nitrosothiol formation is the nitrosation of thiols by nitrous acid (Eq. 9.13).
RSH + HNO2 < 5612 +2O |
(9.13) |

172 |
A Guide to Chalcogen–Nitrogen Chemistry |
S-nitrosothiols may adopt either cis (9.15a) or trans (9.15b) conformations with respect to the S–N bond. S-Nitrosocaptopril45 adopts a cis arrangement, whilst the trans isomer pertains in the solid state for S- nitroso-N-acetylpenicillamine46 and Ph3CSNO47 as determined by X-ray crystallography. The S–N bond lengths are in the range 1.76-1.79 Å typical for a single bond. Nevertheless, there is a significant barrier to rotation about the S–N bond of 11-12 kcal mol-1. The S–N bond dissociation energies are estimated to be about 20-30 kcal mol-1.45, 47-49
|
|
|
|
|
O |
S |
|
N |
S |
|
N |
|
|
||||
R |
|
O |
R |
|
|
9.15a (cis) |
9.15b |
(trans) |
In solution the cis and trans isomers may co-exist, as demonstrated by 15N NMR and UV-visible spectra. The 15N NMR chemical shift of the trans isomer is shifted ca. 60 ppm downfield relative to the cis isomer.45 The visible absorption band of S-nitrosothiols corresponds to a weak n : Œ* transition in the 520-590 nm region. The absorption maxima of trans conformers are red-shifted by ca. 30 nm relative to those of the cis isomer. Two absorptions are observed in the 520-590 nm region in the experimental spectra of RSNO derivatives.45
Stable S-nitrosothiols have also been generated by attaching bowlshaped aryl substituents to the nitrogen centre.50a This approach has also been successful for the isolation of the first S-nitrososelenol as stable purple crystals which, like the sulfur analogue, adopts a cis conformation.50b The related alkyl derivative (Me3Si)3CSeNO is prepared by nitrosation of (Me3Si)3CSeH with tert-butylnitrite.50c This nitrosated selenium species is much less stable that the corresponding S- nitrosothiol. It is a red compound, which decomposes above –78°C. EPR spectra indicate that the formation of the radical [(Me3Si)3CSe(NO)2]• occurs during this decomposition, which produces the corresponding diand tri-selenides.
Chalcogen–Nitrogen Oxides |
173 |
The ability of S-nitrosothiols to mimic many of the biological properties of NO itself may emanate from in vivo decomposition to generate NO. This decomposition is catalyzed by Cu2+,2 and it has been demonstrated that PVC or polyurethane films doped with a lipophilic copper(II) complex are capable of catalytically decomposing endogenous RSNO species to NO. This observation may be important in the development of thrombo-resistant devices used in kidney dialysis or coronary by-pass surgery.51 It is also possible that direct transfer of NO from RSNO may occur in biological systems, since this process has been established to take place at an iron centre with the use of model nonhaem iron systems.52
9.8 Cyclic Chalcogen-Nitrogen Oxides
Five-, sixand eight-membered chalcogen-nitrogen ring systems in which oxygen is attached to one or more of the chalcogen atoms, as well as bicyclic species, have been structurally characterized. The discussion of these heterocycles is arranged into two sections: (a) neutral compounds and (b) anions. The neutral systems are limited to those that
contain sulfur while the anions include the selenium-nitrogen oxo-anion [Se3N3O6]3-.
9.8.1 Neutral compounds
The sulfur-nitrogen oxide S3N2O (thiodithiazyl oxide) is a red liquid. It was first prepared in 1975 by the reaction of (Me2SnS2N2)2 with SOF2,53a
but it is more conveniently prepared from [S3N2Cl]Cl (Eq. 9.14).53b
[S3N2Cl]Cl + HCOOH : 63N2O + 2HCl + CO (9.14)
The 15N NMR spectrum of S3N2O exhibits two resonances consistent with the five-membered ring structure (9.16).54 Lewis acids such as SnCl4 form 2:1 adducts with 9.16, in which the ligands are coordinated to tin in a cis configuration through their oxygen atoms.54 The neutral compound S3N2O5 is obtained when S4N4 or S(NSO)2 is treated with an

174 |
A Guide to Chalcogen–Nitrogen Chemistry |
excess of SO2.55 It has a cyclic structure (9.17) in which the ring oxygen atom is displaced by ca. 0.6 Å out of the mean plane of the S3N2 unit.
Cyclotetrathiazene dioxide S4N4O2 (9.18) is obtained as orangeyellow needles by the condensation of [S3N2Cl]Cl with sulfamide SO2(NH2)2.56 The S–N bond lengths in 9.18 indicate a structure in which two –N=S=N– units bridge a sulfur atom and an SO2 group to form a boat-shaped eight-membered ring. Sodium methoxide attacks 9.18 at the sulfur atom opposite to the SO2 group to give [S4N4O2(OMe)]-, an eightmembered ring with a transannular S•••S interaction.57 Other nucleophiles promote ring contraction. For example, the reaction of 9.18 with AsPh3 produces the five-membered ring S3N2=NSO2N=AsPh3, which undergoes a self-condensation to give the eleven-atom sulfurnitrogen chain Ph3As=N–SO2–N=S=N–S–N=S=N–SO2–N=AsPh3.58
O |
|
|
S |
|
|
N |
|
|
|
|
|
N |
N |
|
S |
N |
O |
||
S S |
|
|
|
|
|
|
|
||
|
O |
|
|
O |
S |
|
|
S |
|
|
|
S |
S |
|
|
||||
N |
N |
|
|
|
S |
|
O |
||
|
O |
|
|
N |
N |
||||
S |
|
|
|
O |
|
|
|||
|
|
O |
|
|
|
|
|
||
9.16 |
|
|
9.17 |
|
|
|
9.18 |
|
|
9.8.2The anions [S3N3Ox]- (x = 1, 2, 4), [E3N3O6]3- (E = S, Se) and [S4N5Ox]- (x = 1,2)
An early application of 15N NMR spectroscopy for monitoring the reactions of sulfur-nitrogen compounds involved the oxidation of the anion [S3N3]-.59 It was shown that controlled air oxidation allows the introduction of one or two oxygens on one of the sulfur atoms to give red [S3N3O]- (9.19) and purple [S3N3O2]- (9.20), respectively. The [S3N3O2]- anion is also formed by ring contraction of 9.18 under the influence of azide anion. Another member of the series of cyclic N-S-O anions, the yellow anion [S3N3O4]- (9.21), is produced as the [S6N4]2+ salt from the treatment of 9.18 with SO3.60 The six-membered rings in the anions 9.19 and 9.20 exhibit significant differences in chemically equivalent bond lengths and bond angles. The structural features of the anion 9.21 are similar to those of the isoelectronic neutral molecule 9.17.
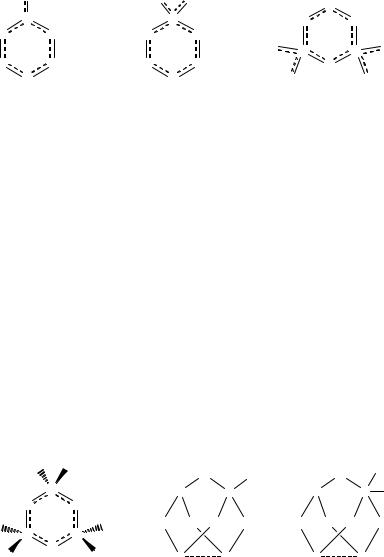
|
|
Chalcogen–Nitrogen Oxides |
|
175 |
|
|
O |
O |
O |
|
S |
|
|
|
|||
|
S |
|
S |
|
|
|
|
N |
N |
||
N |
N |
N |
N |
O S |
- |
|
- |
|
- |
S O |
|
S |
S |
S |
S |
|
N |
|
N |
|
N |
O |
O |
|
9.19 |
|
9.20 |
|
9.21 |
The [NSO2]- anion, isoelectronic with SO3, may be obtained as the ammonium salt by the reaction of SO2(NH2)2 with sulfuryl chloride followed by treatment with ammonia. It forms a trimer in the form of a chair-shaped, six-membered ring [N3S3O6]3- (9.22a), which exhibits mean S–N bond lengths of 1.60 Å and approximately equal S–O bond lengths.61 The selenium analogue [N3Se3O6]3- (9.22b) adopts a similar
conformation in the tripotassium salt with mean Se–N bond lengths of 1.77 Å.62
The yellow [S4N5O]- anion (9.23) is obtained as the water-soluble ammonium salt from the reaction of SOCl2 with liquid ammonia.63 The structure of 9.23 is related to that of the binary anion [S4N5]- (Section 5.4.7); the oxygen atom in 9.23 is attached to a sulfur that is connected to three nitrogen atoms and the unbridged S•••S distance is 2.63 Å.63 The [S4N5O2]- ion (9.24) has been prepared from 9.20 by oxidative-addition with PhICl2, followed by treatment with Me3SiNSNSiMe3.64 Mild heating converts 9.24 back to 9.20 via extrusion of an –NSN- bridging unit.
|
O |
O |
|
N |
O |
|
N |
|
O |
|
E |
|
|
S |
|
|
|||
|
|
S |
- |
S |
- |
S |
O |
||
|
N |
N |
|
|
|
|
|
||
|
|
|
|
|
|
|
|
||
O |
3- |
O |
N |
N N |
N |
N N |
N |
|
N |
E |
|
||||||||
|
E |
|
|
|
|
|
|
|
|
|
N |
O |
S |
|
S |
S |
|
S |
|
|
O |
|
|
|
|||||
|
9.22a (E = S) |
|
9.23 |
|
|
9.24 |
|
|
|
|
9.22b (E = Se) |
|
|
|
|
|
|
|
176 |
A Guide to Chalcogen–Nitrogen Chemistry |
References
1.F. Seel, Sulfur in History: The Role of Sulfur in “Black Powder”, in A. Müller and B. Krebs (ed.) Sulfur: Its Significance for Chemistry, for the Geo-, Bioand Cosmophere and Technology”, Elsevier, Amsterdam, pp. 55-66 (1984).
2.D. L. H. Williams, Acc. Chem. Res., 32, 869 (1999).
3.A. F. Hill, Adv. Organomet. Chem., 36, 159 (1994).
4.A. Haas, Adv. Heterocycl. Chem., 71, 115 (1998).
5.D. A. Armitage and J. C. Brand, J. Chem. Soc., Chem. Commun., 1078 (1979).
6.S. Mann and M. Jansen, Z. Anorg. Allg. Chem., 621, 153 (1995).
7.W. Heilemann and R. Mews, Chem. Ber., 121, 461 (1988).
8.S. Mann and M. Jansen, Z. Naturforsch., 49B, 1503 (1994).
9.S. P. So, Inorg. Chem., 28, 2888 (1989).
10. F. Seel, R. Kuhn, G. Simon, M. Wagner, B. Krebs and M. Dartmann,
Z.Naturforsch., 40B, 1607 (1985).
11.F. Seel, G. Simon. J. Schuh, M. Wagner, B. Wolf, I. Ruppert and A. B. Wieckowski,
Z.Anorg. Allg. Chem., 538, 177 (1986).
12.(a) K. O. Christe, J. A. Boatz, M. Gerken, R. Haiges, S. Schneider. T. Schroer, F. S. Tham. A. Vij, V. Vij, R. I. Wagner and W. W. Wilson, Inorg. Chem., 41, 4275 (2002); (b) A. Kornath, O. Blecher and R, Ludwig, Z. Anorg. Allg. Chem., 628, 183 (2002).
13.D. A. Armitage and A. W. Sinden, Inorg. Chem., 11, 1151 (1972).
14.A. Haas, J. Kasprowski, K. Angermund, P. Betz, C. Kruger, Y-H. Tsay and
S.Werner, Chem. Ber., 124, 1895 (1991).
15.A. Haas, Chem.-Ztg., 106, 239 (1982).
16.J. Weiss, Z. Naturforsch., 16B, 477 (1961).
17.R. Gleiter and R. Bartetzko, Z. Naturforsch., 36B, 492 (1981).
18.(a) G. MacLean, J. Passmore, P. S. White, A. Banister and J. A. Durrant, Can. J. Chem., 59, 187 (1981); (b) R. Steudel, J. Steidel and N. Rautenberg, Z. Naturforsch.,
35B, 792 (1980).
Chalcogen–Nitrogen Oxides |
177 |
19.A. Haas and R. Pohl, Chimia, 43, 261 (1989).
20.(a) H. W. Roesky, M. Thomas, P. G. Jones, W. Pinkert and G. M. Sheldrick,
J.Chem. Soc., Dalton Trans., 1211 (1983): (b) H. W. Roesky, M. Thomas, J. W. Bats and H. Fuess, Inorg. Chem., 22, 2342 (1983).
21.H. W. Roesky, J. Anhaus and W. S. Sheldrick, Inorg. Chem., 23, 75 (1984).
22.J. Weiss, R. Mews and O. Glemser, J. Inorg. Nucl. Chem., Suppl., 213 (1976).
23.T. Chivers, F. Edelmann, U. Behrens and R. Drews, Inorg. Chim. Acta, 116, 145 (1986).
24.A. Haas, J. Kasprowski and M. Pryka, Chem. Ber., 125, 789 (1992).
25.R. Boese, J. Dworak, A. Haas and M. Pryka, Chem. Ber., 128, 477 (1995).
26.P. W. Schenk, Ber. Dtsch. Chem. Ges., 75, 94 (1942).
27.M. Herberhold, B. Distler, H. Maisel, W. Milius, B. Wrackmeyer and P. Zanello,
Z.Anorg. Allg. Chem., 622, 1515 (1996).
28.(a) E. Parkes and J. D. Woollins, Inorg. Synth., 25, 48 (1989); (b) J. F. Davis and
L.D. Spicer, Inorg. Chem., 19, 2191 (1980).
29.W. Verbeek and W. Sundermeyer, Angew. Chem., Int. Ed. Engl., 8, 376 (1969).
30.K. Vrieze and G. van Koten, J. R. Netherlands Chem. Soc., 99, 145 (1980).
31.W. H. Kirchoff, J. Am. Chem. Soc., 91, 2437 (1969).
32.B. Beagly, S. J. Chantrell, R. G. Kirby and D. G. Schmidling, J. Mol. Struct., 25, 319 (1975).
33.K. O. Christe, M. Gerken, R. Haiges, S. Schneider, T. Schroer, F. S. Tham and
A.Vij, Solid State Sci., 4, 1529 (2002).
34.M. Herberhold and W. Jellen, Z. Naturforsch., 41B, 144 (1986).
35.T. Maaninen, R. Laitinen and T. Chivers, Chem. Commun., 1812 (2002).
36.T. Maaninen, T. Chivers, R. Laitinen, G. Schatte and M. Nissinen, Inorg. Chem., 39, 5341 (2000).
37.T. Chivers and G. Schatte, Abstract P-40, 10th International Symposium on Inorganic Ring Systems, Burlington, Vermont, U.S.A. (2003).
178 |
A Guide to Chalcogen–Nitrogen Chemistry |
38.A. F. Hill, G. R. Clark, C. E. F. Richard, W. R. Roper and M. Herberhold,
J.Organomet. Chem., 401, 357 (1991).
39.(a) S. J. Laplaca and J. A. Ibers, Inorg. Chem., 5, 405 (1966); (b) L. Vaska and
S.S. Bath, J. Am. Chem. Soc., 88, 1333 (1966).
40.G. Hartmann, R. Hoppenheit and R. Mews, Inorg. Chim. Acta, 76, L201 (1981).
41.F. Pauer and D. Stalke, J. Organomet. Chem., 418, 127 (1991).
42.J. K. Brask, T. Chivers, M. Parvez and G. Schatte, Angew. Chem., Int. Ed. Engl., 36, 1986 (1997).
43.J. K. Brask, T. Chivers, B. McGarvey, G. Schatte, R. Sung and R. T. Boeré, Inorg. Chem., 37, 4633 (1998).
44.J. K. Brask, T. Chivers and M. Parvez, Angew. Chem., Int. Ed. Engl., 39, 958 (2000).
45.M. Bartberger, K. N. Houk, S. C. Powell, J. D. Mannion, K. Y. Lo, J. S. Stamler, and E. J. Toone, J. Am Chem. Soc., 122, 5889 (2000).
46.G. E. Carnahan. P. G. Lenhert and R. Ravichandran, Acta Crystallogr., B34, 2645 (1978).
47.N. Arulsamy, D. S. Bohle, J. A. Butt, G. I. Irvine, P. A. Jordan and E. Sagan, J. Am. Chem. Soc., 121, 7115 (1999).
48.J-M. Lü, J. M. Wittbrodt, K. Wang, Z. Wen, H. B. Schegel, P. G. Wang and J-P. Cheng, J. Am. Chem. Soc., 123, 2903 (2001).
49.D. Bartberger, J. D. Mannion, S. C. Powell, J. S. Stamler, K. N. Houk and
E.J. Toone, J. Am. Chem. Soc., 123, 8868 (2001).
50.(a) K. Goto, Y. Hino, Y. Takahashi, T. Kawashima, G. Yamamoto, N. Takagi and
S.Nagase, Chem. Lett., 1204 (2001); (b) K. Goto, K. Shimada and T. Kawashima, Abstract IL-3, IXth International Conference on the Chemistry of Selenium and Tellurium, Bombay, India, 2004; (c) C. Wismach, W-W. du Mont, P. G. Jones,
L.Ernst, U. Papke, G. Mugesh, W. Kaim, M. Wanner and K. D. Becker, Angew. Chem., Int. Ed. Engl., 43, 3970 (2004).
51.B. K. Oh and M. E. Meyerhoff, J. Am. Chem. Soc., 125, 9552 (2003).
52.A. R. Butler, S. Elkins-Daukes, D. Parkin and D. L. H. Williams, Chem. Commun., 1732 (2001).
Chalcogen–Nitrogen Oxides |
179 |
53.(a) H. W. Roesky and H. Wiezer, Angew. Chem., Int. Ed. Engl., 14, 258 (1975);
(b) H. W. Roesky and M. Witt, Inorg. Synth., 25, 49 (1989).
54.H. W. Roesky, M. Kuhn and J. W. Bats, Chem. Ber., 115, 3025 (1982).
55.E. Rodek, N. Amin and H. W. Roesky, Z. Anorg. Allg. Chem., 457, 127 (1979).
56.H. W. Roesky, W. Schaper, O. Petersen and T. Müller, Chem. Ber., 110, 2695 (1977).
57.H. W. Roesky, M. Witt, B. Krebs and H. J. Korte, Angew. Chem., Int. Ed. Engl., 18, 415 (1979).
58.M. Witt, H. W. Roesky, M. Noltemeyer, W. Clegg, M. Schmidt and G. M. Sheldrick, Angew. Chem., Int. Ed. Engl., 20, 974 (1981).
59.T. Chivers, A. W. Cordes, R. T. Oakley and W. T. Pennington, Inorg. Chem., 22, 2429 (1983).
60.H. W. Roesky, M. Witt, J. Schimkowiak, M. Schmidt, M. Noltemeyer and G. M. Sheldrick, Angew. Chem., Int. Ed. Engl., 21, 538 (1982).
61.C. Leben and M. Jansen, Z. Naturforsch., 54B, 757 (1999).
62.V. Kocman and J. Rucklidge, Acta Crystallogr., B30, 6 (1974).
63.R. Steudel, P. Luger and H. Bradaczek, Angew. Chem., Int. Ed. Engl., 12, 316 (1973).
64.R. T. Boeré, R. T. Oakley and M. Shevalier, J. Chem. Soc., Chem. Commun., 110 (1987).
Chapter 10
Acyclic Organo Chalcogen–Nitrogen
Compounds
10.1 Introduction
In this chapter the chemistry of acyclic organic derivatives containing a chalcogen–nitrogen functional group as the central feature will be discussed. The simplest examples are thionitrosyls RNS isovalent with nitroso compounds RNO. Little is known about the Se and Te analogues RNE (E = Se, Te) although the cyclic trimers have been characterized (Section 6.3). Sulfur–nitrogen compounds with the composition RNS2 exist as N-thiosulfinylamines RN=S=S with unbranched structures rather than the branched structures that are typical of the corresponding nitro compounds RNO2. Chalcogen diimides RN=E=NR (E = S, Se, Te) represent a very widely studied class of chalcogen-nitrogen compounds for which detailed comparisons between the behaviour of the three chalcogens can be made. These compounds are isoelectronic with SO2, (SeO2)\ and (TeO2)\. Investigations of their reactivity have led to the synthesis of imido analogues of other sulfur-oxygen species such as SO3, [SO3]2- and [SO4]2-, i.e., S(NR)3, [S(NR)3]2- and [S(NR)4]2-, respectively. Hybrid systems of the type RN=E=O (sulfinylamines) are covered under the rubric of chalcogen-nitrogen oxides in Section 9.6.
The foregoing compounds all contain imido groups (NR) attached to the chalcogen centre. A second, very important class of acyclic organo chalcogen–nitrogen compounds involves those in which an amido group (NR2) is linked to the chalcogen, e.g., E(NR2)2 (E = S, Se, Te). These compounds have a well-developed chemistry and notable differences
180