
History of Modern Genetics in Germany
.pdf224 |
G.C. Sahoo, N.N. Dutta |
is maximized by irreversibly reacting the solute with the reagent into an impermeable form in the receiving phase (usually internal phase) thereby maintaining the permeate concentration at effectively zero in this phase. In Type II facilitation, a carrier is incorporated in the membrane phase and it carries the diffusing solute across the membrane to the receiving phase, a mechanism commonly known as “carrier-mediated” facilitated transport.
Emulsion liquid membrane extraction of cephalosporins conform to the type II facilitated transport. Here the solute transport is either associated with a cotransport or counter-transport of an anionic species depending on whether ionpair or ion-exchange extraction is exploited in the ELM system.
Various models have been proposed to describe the facilitated mass transfer phenomena, although five basic categories of models have mostly been reported in the literature [29]. The same models can essentially be applicable for Type II facilitated transport.
The mathematical model essentially involves rate equations capable of describing these phenomena. Although there are number of models for unfacilitated transport [42], there exist only a few quantitative models for facilitated transport in emulsion liquid membrane. In addition to mass transfer in emulsion liquid membrane, the chemical reaction between the solute, carrier, and the internal reagent should be considered. In such a system there are three possible rate controlling steps: mass transfer through the external phase, chemical reaction(s), and diffusion through the membrane phase. The aim of the modeling study is to simulate experimental data and identify which, if any, of these three steps controls the extraction rate. Table 2 shows the reported modeling studies for b-lactams and related biomolecules.Phenomenologically the models include mass transfer in the external phase, the solute-carrier chemical reaction, diffusion in the emulsion globule as described by a shrinking core model, and the stripping reaction [70, 71].
The following steps are considered for the transport process in developing such model which has been adopted for cephalosporins permeation study at the authors’ laboratory [25]:
1.Diffusion of cephalosporin anion (P–) from the bulk feed phase (III) to feedmembrane (III–II) interface of the emulsion globule
2.Reaction of P– ion with the carrier QCl at the external interface releasing the counter-ion Cl–
3.Diffusion of the solute-carrier complex, QP into the emulsion globule
4.Stripping of the complex at the internal interface between the membrane (II) and internal (I) phase
5.Back diffusion of the carrier to the feed (III) and membrane (II) phase interface
The following assumptions are inherent in the above process:
1.The extraction and stripping reactions are located at the interfaces and the amount of carrier dissolved in the aqueous phases is negligible.
2.Negligible transport resistance exists in the internal phase (I) because the phase I droplets are very small (r1< 5 mm): a quasi-homogeneous state.
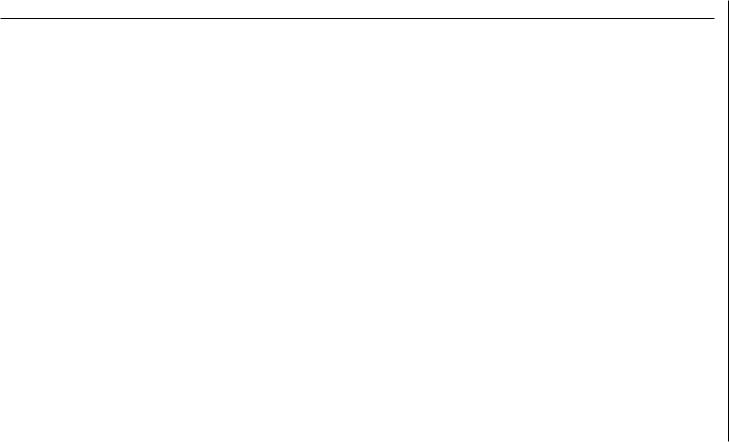
Table 2. Reported studies on facilitated transport in liquid membrane for b-lactam antibiotics and related biomolecules
Species/Solute |
Carrier |
Internal |
Co/Counter |
LM type |
Mathematical |
Inference |
Reference |
||
|
|
reagent |
|
transport |
|
model |
|
|
|
|
|
|
|
|
of ion |
|
|
|
|
|
|
|
|
|
|
|
|
||
Lactic acid |
Secondary |
Sodium |
Co transport |
ELM |
Not done |
Selectivity studies in relation to |
[46] |
||
|
amine LA-2 |
carbonate |
of H+ |
ELM |
Not done |
acetic, malic, gluconic acid, etc. |
|
||
|
Tertiary |
-do- |
|
-do- |
ELM |
Shrinking core |
Effect of convective transport and |
[70] |
|
|
amine |
|
|
|
|
|
model |
swelling accounted for modeling |
|
|
Alamine-336 |
|
|
|
|
|
|
and model verification |
|
Lactic acid |
Amberlite LA-2 |
Na2CO3 |
|
Co-transport |
ELM |
Simplified reac- |
Reaction controlled transport |
[46] and |
|
and Leucine |
and Aliquat-336 |
and NaCl |
of OH– counter- |
|
tion-diffusion |
mechanism |
references |
||
|
|
|
|
|
transport of Cl– |
|
model |
|
cited therein |
Lactic acid |
Secondary amine |
Na2CO3 |
|
Co-transport |
ELM |
Model of mass |
pH variation occurred |
[71] |
|
|
|
and NaOH |
of OH– |
|
transfer in exter- |
|
|
||
|
|
|
|
|
|
|
nal layer and in- |
|
|
|
|
|
|
|
|
|
ternal diffusion |
|
|
|
|
|
|
|
|
|
in globule |
|
|
Lactic acid |
Aliquat-336 |
NaCl, |
|
Counter-trans- |
BLM |
Not done |
NaCl provides highest recovery |
[33] |
|
|
|
Na |
CO |
, |
port of Cl– |
|
|
and fastest kinetics of lactic acid |
|
|
|
2 |
3 |
|
|
|
|
|
|
|
|
Na2SO4 |
|
|
|
|
pre-concentrated in the aqueous |
|
|
|
|
|
|
|
|
|
|
solution containing 0.5 mol/L |
|
|
|
|
|
|
|
|
|
of NaCl |
|
Amino acid |
-do- |
– |
|
|
Counter-trans- |
ELM |
– |
Chiroselective transport |
[93] |
|
|
|
|
|
port of OH– |
|
|
|
|
Mendalic acid |
Optically active |
– |
|
|
Counter-trans- |
BLM |
– |
– |
[94] |
|
benzyl derivative |
|
|
|
port of H+ |
BLM |
|
|
|
L-Phenyl- |
Di-2-ethylhexyl |
H2SO4 |
|
Counter-trans- |
ELM |
– |
Concentration as cation |
[47] |
|
alanine |
phosphoric acid |
|
|
|
port of H+ |
|
|
|
|
(D2EHPA)
Antibiotics Cephalosporin of Extraction Membrane Liquid in Perspectives
225

Table 2 (continued)
Species/Solute |
Carrier |
Internal |
Co/Counter |
LM type |
Mathematical |
Inference |
Reference |
|
|
reagent |
transport |
|
model |
|
|
|
|
|
of ion |
|
|
|
|
|
|
|
|
|
|
|
|
L-phenyl- |
Aliquat-336 |
KCl and |
Counter-trans- ELM |
– |
Concentration as anion carrier |
[45, 95| |
|
alanine and |
|
KOH |
port of Cl– |
|
|
specificity for various aminoacids |
|
various other |
|
|
|
|
|
depends on hydrophobicity scale |
|
amino acids |
|
|
|
|
|
|
|
Phenylalanine |
D2EHPA |
KCl in re- |
Counter-trans- |
BLM (RFP) |
– |
Continuous extraction and |
[96] |
and Lysine |
|
ceiving phase |
port of K+ |
|
|
concentration |
|
Diand tri- |
D2EHPA |
HCl |
Counter-trans- ELM |
– |
Concentration and permeation |
[97] |
|
peptides |
|
|
port of H+ |
|
|
depends on hydrophobicity scale |
|
Amino acids |
Crown ether and |
– |
– |
SLM |
– |
Highly selective separation and |
[98, 99] |
in general |
open chain poly- |
|
|
|
|
enantiomeric resolution |
|
|
mer of transtetra- |
|
|
|
|
|
|
|
hydro furan, |
|
|
|
|
|
|
|
2,5-bis acetylene |
|
|
|
|
|
|
|
unit |
|
|
|
|
|
|
Dipeptide and |
Kryptafix2 |
HCl in re. |
Counter-trans- |
SLM in HF |
– |
Stereoselective permeation of 1–1 |
[100] |
phosphono di- |
(crown ether) |
ceiving phase port of Cl– |
|
|
dipeptide |
|
|
peptide and |
|
|
|
|
|
|
|
several amino |
|
|
|
|
|
|
|
acids |
|
|
|
|
|
|
|
Penicillin-G |
Amberlite LA-2 |
Na2CO3 |
Co-trans- |
ELM |
Simple model of |
Optimum extraction condition |
[50, 53, 54, |
|
and dioctylamine |
|
port of H+ |
ELM |
permeation rate |
and procedure for determining |
100] |
|
|
|
|
|
accounting for pro- |
the internal reagent worked out. |
|
|
|
|
|
|
duct decomposition |
DOA is a better carrier |
|
|
|
|
|
|
|
|
|
226
Dutta .N.N Sahoo, .C.G

Table 2 (continued)
Amberlite LA-2 -do- |
-do- |
SLM |
Simple flux equation |
Optimal condition determined |
[57, 58] |
|
|
|
based on film theory |
with respect to pH of the aqueous |
|
|
|
|
considering aqueous |
phase |
|
|
|
|
boundary layer re- |
|
|
|
|
|
sistance and mem- |
|
|
|
|
|
brane diffusion |
|
|
7-ACA, cepha- |
Aliquat-336 |
NaCl |
Counter-trans- BLM |
Simple mass |
|
lexin, CPC |
|
|
port of Cl– |
|
transfer model |
CPC |
Aliquat-336 |
NaCl |
-do- |
SLM (poly- |
Analysis of per- |
|
|
|
|
prop-ylene |
meation controlling |
|
|
|
|
flat sheet) |
steps from mass |
|
|
|
|
|
transfer model |
|
|
|
-do- |
HF |
Mass transfer model |
|
|
|
|
extraction |
|
Cepalexin with |
-do- |
-do |
-do |
ELM |
Model incorporat- |
7-ADCA and |
|
|
|
|
ing external mass |
CPC including |
|
|
|
|
transfer and diffu- |
broth |
|
|
|
|
sion in globule |
pH gradient or Cl– counter trans |
[23, 24, 26] |
port can provide the driving force |
|
Membrane diffusion controlling |
[56] |
Simultaneous extraction and strip- [27] ping possible in two HF modules [25, 26] Selective separation of cephalexin
from mixture with 7-ADCA and of CPC from deacetylCPC of broth
Antibiotics Cephalosporin of Extraction Membrane Liquid in Perspectives
227

228 |
G.C. Sahoo, N.N. Dutta |
3.Resistance in the oil layer (surfactant monolayer) at the external interface is negligible.
4.The stripping reaction is very fast due to large surface area of the phase I.
Figure 5 shows the concentration profile of cephalosporin anion, P– in the emulsion globule. Applying two film theory, the mass transfer rate of P– from bulk of phase III through the boundary layer of phase III to the III – II interface is given by
dCP– = – |
1 km Sem (CP– – C*P–) |
(15) |
dt |
Vm |
|
8 |
6 |
|
where C is the solute concentration in the external phase, C* is the concentra-
P– P–
tion at the phase III–II interface, kIII is the external phase mass transfer coefficient, Sem is the total surface area of the emulsion globules, and VIII is the external phase volume.
Considering interfacial chemical reaction at III–II interfaces, the following equation holds:
dCP– |
= – Sem k1C*P– C*QCl |
(16) |
dt |
VIII |
|
8 |
6 |
|
= RF = extraction reaction rate per unit area of III–II interface, where k1 is the forward reaction rate constant for the extraction reaction.
The diffusion of the solute-carrier complex in the emulsion is described by Fick’s second law and is given by the following equation:
V |
VII |
dCQP |
d |
r2 |
dCQP |
– |
S1 |
(17) |
+ VIII |
dt |
= Deff r2 dr |
dr |
VI + VII k–1CQPCCl– |
||||
04I |
9 |
8 9 |
|
94 |
|
where S1 is the total surface area of the internal phase droplets (phase I), Deff is the effective diffusivity of the complex, k–1 is the rate constant for the backward
Fig. 5. Concentration profile of cephalosporin in an emulsion liquid membrane
Perspectives in Liquid Membrane Extraction of Cephalosporin Antibiotics |
229 |
reaction,and VI and VII are the phase volumes of the internal aqueous and membrane phases, respectively.
The equilibrium constant,Keq for the complexation reaction (Eq. 4) can be expressed as
Kcq = CQP CCl–
03C C*
QCl P–
where
CQCl = C0QCl – CQP
and from Eqs. (18) and (19)
C*– = CCl– |
C0QCl – 1 |
|
||||
P |
Keq |
CQCl |
|
|||
or |
7 |
8 |
|
|
|
|
|
|
CCl– CQP |
|
|
|
|
C*– = |
|
|
|
|
||
P |
K |
|
(C0 – C |
|
|
) |
|
006 |
|||||
|
|
eq |
QCl |
QP |
|
The initial and boundary conditions are given by:
t = 0, CP– = C0P–, CQP = 0, (0 £ r £ R)
Boundary conditions at the center of globule to have no flux is
r = 0, dCQP = 0, (t ≥ 0)
8dr
At the feed-membrane interface
r = R, D |
dCQP |
= k (C |
– |
– C* ) |
|
|
eff 8dr |
m P |
P |
– |
|
|
|
|
(18)
(19)
(20a)
(20b)
(21)
(22)
(23)
The following relationships may be used for the solution of the above model equations:
1. The number of emulsion globules, Nm is given by
VI + VII
Nm = (4/3)05pR3
where R is the mean globule radius, D32/2
with D32 = ∑nId3i /∑ni d2i (Sauter mean diameter) where ‘n’ is the number of globules of diameter of ‘d’
2. Surface area of emulsion globule, Sem is given by
Sem = 3 (VI + VII)
08R
3. The total surface area of internal droplets of phase I
SI = 3VI
7rI
(24)
(25)
(26)
230 |
G.C. Sahoo, N.N. Dutta |
In order to solve the mathematical model for the emulsion liquid membrane,the model parameters, i.e., external mass transfer coefficient (KIII), effective diffusivity (Deff), and rate constant of the forward reaction (k1) can be estimated by well known procedures reported in the literature [72–74]. The external phase mass transfer coefficient can be calculated by the correlation of Calderback and Moo-Young [72] with reasonable accuracy. The value of the solute diffusivity (Da) required in the correlation can be calculated by the well-known WilkeChang correlation [73]. The value of the diffusivity of the complex involved in the procedure can also be estimated by Wilke-Chang correlation [73] and the internal phase mass transfer co-efficient (surfactant resistance) by the method developed by Gu et al. [75].
The kinetic constant, k1 can be calculated from Lewis cell experiments [46] under the condition of higher carrier concentration compared to that of solute in aqueous phase, so that the mass transfer rate is controlled by the transport of the solute to the interface and the rate of reaction at the interface.
Equations (15)–(23) can be solved numerically using a combination of IMSL and NAG library subroutines. The DIVPAG subroutine of IMSL for ordinary differential equations and the D03PAF subroutine of NAG library for partial differential equations may serve the purpose and we applied this method for simulating the results for ELM extraction of CPC [26] and cephalexin [25]. The agreement between model prediction and experimental data was found to be quite reasonable.
4.3
Supported Liquid Membrane
The permeation of cephalosporin antibiotics in an SLM system under reactive extraction condition can be considered a coupled transport phenomenon which can describe metal ion permeation behavior. Various steps are involved in the coupled transport in the SLM system:
–Step 1: the P– ion, after diffusing to the feed-SLM interface, reacts with QCl (Eq. 4) forming a solute-carrier complex, QP: Cl– ions are simultaneously released into the feed solution (coupled transport).
–Step 2: QP diffuses across the membrane because of negative concentration gradient.
–Step 3: at the SLM-stripping interface, QP releases P– ions into the aqueous phase, Cl– ions replace P– ions in the membrane (coupled transport).
–Step 4: the uncomplexed carrier, QCl returns across the membrane, the uncomplexed solute P– cannot diffuse back because of low ionic solubility in the membrane phase.
Since the ion exchange reactions are fast, decomposition of CPC does not occur at the pH range used [50]. In the stripping side, the presence of buffer anion (B–) of the buffer acid (BH) is likely to cause another ion exchange reaction according to
B– + QCl ¤ QB + Cl– |
(27) |
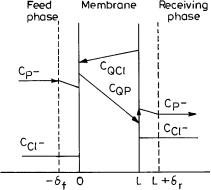
Perspectives in Liquid Membrane Extraction of Cephalosporin Antibiotics |
231 |
This reaction is suppressed by using a large excess of Cl– ion in the stripping phase. Furthermore,the extraction equilibrium constant of the above reaction is much lower than that of reaction given by Eq. (4). The overall distribution coefficient of CPC,‘mg’ (defined as CQP/CP–) is given by [50]
[Keq C0BH – {(1 – Kd) CH/Ka + 1} CB–] |
(28) |
mg = |
|
KACB– |
|
000000 |
|
with |
|
log (mg) = log (Keq) + log (CQCl /CCl–) |
(29) |
where Keq and KA are the extraction equilibrium constants of the reaction given by Eqs. (4) and (27), respectively and Kd and Ka are the distribution equilibrium constant (between organic and aqueous phases) and dissociation constant, respectively, for the buffer acid.
The CPC present in the aqueous phase is distributed between the aqueous and organic phases at the SLM-liquid interface. By maintaining low Cl– ion concentration in the feed phase and high Cl– ion concentration in the stripping phase, the distribution ratio of CPC (P– ion form) at the aqueous feed-SLM interface can be made much higher than that at the aqueous strip-SLM interface. Under this condition, the steady-sate overall CPC flux across the membrane can be obtained from Fick’s distribution law applied to aqueous diffusion film as well as the membrane itself and from interfacial reaction kinetics which describe the interfacial flux.
The typical concentration profile of solute in an SLM system with quaternary ammonium salt as carrier is schematically shown in Fig. 6. To model the facilitated transport within a supported liquid membrane [58, 59], the following assumptions are usually made:
–Isothermal process.
–Carrier and solute-carrier complex dissolve in organic phase only.
–The concentration gradient in liquid membrane and aqueous film are linear.
–The mass transfer resistance of chloride ion in feed and stripping solution may be neglected.
Fig. 6. Typical concentration profile in a supported liquid membrane system
232 |
G.C. Sahoo, N.N. Dutta |
–The concentration of solute-carrier complex on the M/R interface is much lower than on F/M interface.
–The decomposition of cephalosporins is negligible.
Thus, the mass transfer flux of solute in feed, liquid membrane, and stripping phases can be expressed by the following equations:
Df |
(CP–, f |
– CP–, 0) = kf (CP–, f – CP–, 0) |
|
(30) |
|||||
Jf = |
|
||||||||
5df |
|
|
|
|
|
|
|
|
|
J = eDm (C |
QP, 0 |
– C |
QP, L |
) = k (C |
QP, 0 |
– C |
) |
(31) |
|
m |
|
|
m |
|
QP, L |
|
|||
8tL |
|
|
|
|
|
|
|
|
|
Dr |
(CP–, L – CP–, r) = kr (CP–, L – CP–, r) |
|
(32) |
||||||
Jr = |
|
||||||||
5dr |
|
|
|
|
|
|
|
|
|
where kf, km, and kr are the mass transfer coefficients in feed, membrane, and receiving phases, respectively; Df and Dr represent the diffusivity of solute in feed and in stripping phase; Dm is diffusivity of the formed complex in liquid membrane phase; L is the thickness of liquid membrane; and e and t are the porosity and tortuosity of the microporous membrane, respectively.
By introducing the distribution coefficients at the two interfaces, i.e., mf and mr ,
Jf = kf (CP–, f – CQP,0 /mf) |
(33) |
Jr = kr (CQP,L/mr – CP–, r) |
(34) |
Under pseudo steady-state condition, the total or overall flux (J) is equal to the flux in each phase, i.e., J = Jr = Jf = Jm.
By eliminating the undetermined carrier concentrations (CQP,O and CQP,L) at the boundaries of the SLM and under the usually valid condition of mr mf , the mass transfer rate of the solute can be deduced as [54]
dcP–, f |
= KLSCP–, f |
|
JS = – Va 9dt |
(35) |
where S represents the cross-sectional area of the membrane and KL is an overall mass transfer coefficient defined as
1 |
1 |
1 mr |
+ |
1 |
= |
+ |
kf mf |
(36) |
|
KL |
kf |
|
mf km |
5 5 5 5 9
The distribution coefficient is a function of solute concentration and Eq. (35) cannot be solved easily. However, solution exists for the following two limiting cases:
– Case 1: CQCl CP–, g
In case of very low solute concentration, the complex concentration in the liquid membrane is almost equal to zero. Thus, the transport process is mainly controlled by aqueous film resistance, and KL is equal to kf, which is independent of time. By integrating Eq. (35), the concentration variation of solute in feed phase
Perspectives in Liquid Membrane Extraction of Cephalosporin Antibiotics |
233 |
at any time (Cp–, t) can be obtained as |
|
Cp–, t |
(37) |
ln Cp–, g = – (S/V) KLt |
|
8 |
|
The permeation or the mass transfer rate in aqueous film can be obtained from the slope of time course of solute concentration in the feed phase.
– Case 2: CQCl CP–,g
In this case, the solute concentration in feed phase is so high that all of the carrier in the liquid membrane are in a complex form and the complex concentration is equal to the overall carrier concentration. So, mf and mr become
mf, = |
CQCL |
(38) |
Cp– |
||
|
8 |
|
Because mr is much smaller than mf and the second term in the denominator of Eq. (36) is neglected, KL is expressed by the following relation:
J |
= |
mf, |
= |
CQCL |
(39) |
|
KL = |
|
|
|
|||
5 06 08 |
||||||
Cp– |
mf, |
+ |
1 |
CQCl |
+ |
1 |
|
kf |
km |
CP– kf |
km |
||
|
6 5 8 5 |
and when CP– is large enough the flux can be expressed as
JS = – V |
dcP–, f |
= CQCl km S = constant |
(40) |
|
9dt |
||||
|
|
|
Integrating Eq. (40), the concentration of solute in feed phase at any time (CP–, t) can be shown as
CP–, t |
= 1 – |
(CQCl km) S |
(41) |
8 |
t |
||
|
04 4 |
|
|
CP–, g |
|
CP–, g V |
|
The mass transfer coefficient in liquid membrane, km can be obtained from the plot of concentration vs. time.
This simple mass transfer model based on simplified film theory has been proposed to describe the process of facilitated transport of penicillin-G across a SLM system [53]. In the authors’ laboratory, CPC transport using Aliquat-336 as the carrier was studied [56] using microporous hydrophobic polypropylene membrane (Celgard 2400) support and the permeation rate was found to be controlled by diffusion across the membrane.
4.4
Dispersion Free Extraction in Hollow Fiber Membrane
In the case of non-dispersive extraction of cephalosporins by reversible chemical complexation taking place across hydrophobic microporous hollow fiber (MHF) modules and co-current flow of the two phases, the solute in the aqueous
- #
- #15.08.20134.04 Mб15Hastie T., Tibshirani R., Friedman J. - The Elements of Statistical Learning Data Mining, Inference and Prediction (2002)(en).djvu
- #
- #
- #
- #
- #
- #
- #
- #15.08.201315.44 Mб23Hudlicky M, Pavlath A.E. (eds.) - Chemistry of Organic Fluorine Compounds 2[c] A critical Review (1995)(en).djvu
- #