
Drug Targeting Organ-Specific Strategies
.pdf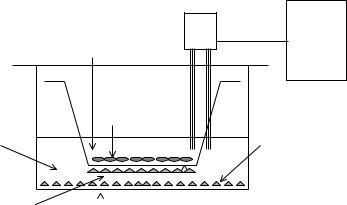
34 2 Brain-Specific Drug Targeting Strategies
2.3.2.2 In Vitro Models
Freshly isolated capillaries from different species are a valuable model system of the BBB [51], because they retain the native repertoire of receptors and enzymes. In particular, receptor binding and carrier-mediated uptake or receptor-mediated endocytosis can be conveniently studied. On which side binding or uptake takes place cannot be easily determined, as both the luminal and abluminal side are exposed in such a preparation.
Numerous modifications of in vitro culture systems have been developed for the estimation of BBB transfer [52]. Culture systems in use are either primary cultures of brain microvessel endothelial cells (BMEC) or immortalized endothelial cell lines. BMEC may be grown in co-culture with astrocytes or in astrocyte-conditioned medium. Astrocyte-derived factors increase the tightness of the barrier as measured by transendothelial electrical resistance (TEER) and by the permeability of hydrophilic markers such as sucrose. They also upregulate the expression of BBB-enriched enzymes such as γ-glutamyl transpeptidase (γ-GTP) and alkaline phosphatase. A setup of the in vitro technique in a transwell system for transport studies is depicted in Figure 2.5.
|
Millicell® |
|
Luminal upper chamber |
ERS |
|
TEER |
||
|
||
|
(ohms•cm2) |
|
BBMECs |
|
|
Abluminal |
C6 Astroglioma |
|
|
lower chamber
C6 Astroglioma |
|
|
Microporous membrane |
|
|
||
|
|
||
|
|
|
(0.4 M pore size) |
|
6 well cluster plate |
Figure 2.5. Setup for in vitro measurement of blood–brain barrier permeability with a co-culture of bovine brain microvascular endothelial cells (BBMEC) and an astroglioma cell line, C6. The BBMEC are grown on top of a filter insert. The C6 cells are either grown on the opposite side of the filter or on the bottom of the wells. Transport across the BBMEC monolayer is measured by adding the test substance to the upper chamber and sampling from the lower chamber. The tightness of the monolayer is also characterized by the transendothelial electrical resistance (TEER). Courtesy of T. Abbruscato.
2.4 Drug Delivery Strategies
The approaches for developing strategies for the delivery of drugs to the brain are shown in groups in the scheme in Figure 2.6. Pharmacological-based and physiological-based methods

2.4 Drug Delivery Strategies |
35 |
utilize noninvasive, systemic administration and a range of methods to enhance delivery through the BBB. Physical strategies in contrast rely on invasive (neuro-)surgical methods of drug administration.
STRATEGIES FOR
BRAIN DELIVERY OF
DRUGS / GENES
PHYSICAL |
PHARMACOLOGIC |
/ PHYSIOLOGIC |
|||
intra- |
intra- |
small |
nano- |
pseudo- |
cationic |
ventricular |
cerebral |
||||
infusion |
implant |
molecules |
particles |
nutrients |
antibodies |
BBB
disruption liposomes chimeric peptides
viral vectors
Figure 2.6. Strategies for drug delivery across the blood–brain barrier (BBB). The physical, pharmacological and physiological approaches are discussed in the text. Present experimental attempts at viral gene delivery would also be classified as invasive because of the intracerebral administration of the vector.
2.4.1 Small Molecule Drug Delivery
In accordance with the structure of the BBB as a double lipid bilayer, classical neuroactive drugs such as benzodiazepines, neuroleptics and tricyclic antidepressive agents, are all small lipophilic molecules. These small molecular weight neuropharmaceuticals were selected by a trial and error approach because their structural characteristics allow for diffusion-mediated, passive penetration through the BBB.
With the development of combinatorial chemistry and (ultra)high throughput screening methods, drug discovery is currently undergoing a rapid evolution. However the drug delivery aspect, in particular with regard to neuropharmaceuticals, is an area, which is lagging behind in efforts to integrate the overall drug development process [53].
The use of prodrugs with higher lipophilicity compared to the parent molecule is realized in the classical example of heroin and morphine. Heroin, the di-acetyl derivative of morphine, penetrates the BBB by one log order better than morphine and is cleaved by tissue esterases to release the active parent drug. As follows from the pharmacokinetic principles shown in Section 2.3.2.1 (Eq. 2.3), brain concentration is a function of both BBB permeability, reflected by Kin, and plasma area under the curve:
Cbrain = Kin x AUC |
(2.4) |
36 2 Brain-Specific Drug Targeting Strategies
While Kin increases with lipophilicity, AUC decreases due to higher uptake across all cell membranes including those of peripheral tissues. Therefore limits are imposed on the gain in brain delivery by the ‘lipidization’ strategy. In fact, for azidothymidine lipidization with the lipophilic adamantane moiety, CSF concentrations decreased by a factor of 10 as a result of a decrease in AUC [54].
Lipidization is also an integral part of the chemical delivery approach that is based on the concept of ‘retro-metabolism’ [55]. The underlying principle is the simultaneous derivatization of a drug with a ‘redox targeter’, e.g. dihydrotrigonellinate, and with a lipophilic moiety, e.g. cholesterol. This strategy has been applied to deliver a small enkephalin analogue to the brain [55]. Once inside the target tissue, the redox targeter will be enzymatically oxidized to a positively charged derivative and serve to lock-in the prodrug. Further enzymatic steps release the active drug by cleavage of the lipid modifier and the targeter. This strategy offers the potential of tissue-selective delivery, yet it requires simultaneous optimization of a multitude of rates, including the influx and efflux of the prodrug, biotransformation of the targeter and eventual release of the active drug.
An alternative delivery strategy for small molecules is based on the presence of the nutrient transporters. Drugs that are structurally similar to substrates of a carrier system can undergo facilitated brain uptake as pseudoneutrients. The best example of this is the therapeutic use of L-DOPA in Parkinson’s disease. Unlike the neurotransmitter dopamine itself, which cannot cross the BBB in significant amounts, its precursor L-DOPA is a substrate for LAT, the transporter of large neutral amino acids [56]. Its uptake by the brain is saturable, and subject to competition by the other substrates of the carrier present in plasma.
L-DOPA therapy is an example of rational drug design based on knowledge of BBB transport biology. A number of other small molecular weight drugs are known to undergo carrier-mediated transport at the BBB. These include the substrates of LAT, such as the anticancer drugs melphalan and acivicin, or the GABAB-agonist baclofen, and beta lactam antibiotics (e.g. benzylpenicilline, ceftriaxone, cefodizime) which are substrates for the organic acid carrier [31].
2.4.2 Macromolecular Drug Delivery
2.4.2.1 Intraventricular Route
At first glance, intracerebroventricular (i.c.v.) or intrathecal administration via catheters, typically connected to a subcutaneous drug reservoir, appears as a logical mode of drug delivery, because the percentage of the dose reaching the target organ would be 100%. The i.c.v. route is successfully used in disorders where pathogenetic events take place close to the brain surface. Clinical examples of intrathecal small drug delivery are the administration of glycopeptide and aminoglycoside antibiotics in meningitis [57], the i.c.v. treatment of meningeal metastasis [58], intrathecal baclofen injection for treatment of spasticity [59] and the infusion of opioids for severe chronic pain [60]. However, in addition to the invasive character of the procedure, it has to be taken into account that drug distribution into brain tissue is severely diffusion-limited and that the continuous production and reabsorption of the CSF results in rapid clearance from that compartment.
2.4 Drug Delivery Strategies |
37 |
Although there is no cellular barrier preventing diffusion from the ventricular surface into brain tissue (there are no tight junctions between the ependymal cells lining the ventricular surface), the low speed of diffusion severely restricts tissue uptake of even small molecular weight drugs and practically prevents the penetration of large molecules such as peptides and proteins into deep tissue layers. Possible enzymatic inactivation and binding or sequestration by brain cells along the diffusion path may even lower the actual drug concentrations in brain interstitial spaces to levels lower than predicted from the molecular size and diffusion coefficients [61]. Figure 2.7a shows an example of a large molecule, the 26-kDa nerve growth factor (NGF), that could not penetrate into rat brain deeper than 1–2 mm from the infused ventricle. This might be expected, as even small drugs show very steep concentration gradients over a distance of only 2–3 mm from the ventricular surface (Figure 2.7b). Exceptions are found in areas to which retrograde transport occurs, e.g. into the neurons of the basal cholinergic nuclei in the case of NGF (Figure 2.7a).
After i.c.v. injection, the rate of elimination from the CNS compartment is dominated by cerebrospinal fluid dynamics. The CSF, which is secreted by the choroid plexus epithelium across the apical membrane, circulates along the surface and convexities of the brain in a rostral to caudal direction. It is reabsorbed by bulk flow into the peripheral bloodstream at the arachnoid villi within both cranial and spinal arachnoid spaces [62]. Of note is that the turnover rate of total CSF volume is species dependent and varies between approximately 1 h in rats and 5 h in humans. In adult human brain, the total CSF volume amounts to 100–140 ml and the production rate is 21 ml h–1 [63]. Accordingly, the entire cerebrospinal volume is exchanged regularly 4–5 times per day. This rapid drainage of CSF into peripheral blood leads to relatively high drug concentrations in the peripheral circulation. For instance, the concentration of methotrexate in peripheral blood reaches 1% of the ventricular CSF concentration following intrathecal administration of the drug [64]. In Rhesus monkeys, parenchymal concentrations of methotrexate of 1% of the intraventricular concentration have been measured at a distance of 2 mm from the ependymal surface [65]. Therefore, the concentration of methotrexate in the blood is actually higher than at tissue regions beyond 2 mm from the ependymal surface following intrathecal application.
2.4.2.2 Intraparenchymal Route
Restricted diffusion also limits tissue distribution after intraparenchymal drug administration, as shown in Figure 2.7c and d. Distribution has been measured in the rat brain after implantation of polymer discs containing NGF [66]. Drug concentrations decreased to less than 10% of the values measured on the disc surface within a distance of 2–3 mm, even after prolonged periods of several days. Therefore, applying this approach in the larger human brain would require the stereotaxic placement of multiple intraparenchymal depots, as has been evaluated in rat brain [67], on a repetitive schedule.
The same pharmacokinetic limitation is true in principle for the implantation of encapsulated genetically engineered cells, which synthesize and release neurotrophic factors [68].

38 2 Brain-Specific Drug Targeting Strategies
(e)
(c)
(a)
2.0
|
|
12 hr |
1.5 |
|
|
2 hr 6 hr |
10, |
1.0 |
Distance, cm |
|
B |
|
0.5 |
|
|
A |
|
|
|
|
|
|
0 |
|
1.0 |
etivlaeRntioatrnecnoC |
0 |
(f) |
|
|
|
|||
|
|
|
|
2 day |
|
|
from polymer, mm |
|
|
|
|
Distance |
|
NGFconcentration,ng/ml |
|
|||
(d) |
||||
|
|
|
||
|
|
|
cm from ventr. surface |
|
tissue |
|
CSF |
(b) |
|
|
||||
C |
|
C |
||
|
|
|
|
2.4 Drug Delivery Strategies |
39 |
2.4.2.3 Convective Flow
Intraparenchymal high flow microinfusions with flow rates up to 4 l min–1 result in almost homogenous tissue concentrations of macromolecules (transferrin, mw 80 kDa) over a large volume and over a distance of > 10 mm from the catheter tip within an infusion period of 2 h [69]. Figure 2.7 demonstrates the principle in an experimental study (Figure 2.7e) and the expected tissue concentration profiles (Figure 2.7f). The plateau-like concentration profile at the front of the convective flow is in contrast to the concentration gradients associated with diffusion-mediated distribution (Figure 2.7b, d). The method has been applied in clinical trials for treatment of gliomas and metastatic brain tumours with a transferrin-targeted mutant diphtheria toxin,Tf-CRM107 [70]. Current efforts are directed at overcoming the dose-limit- ing toxicities found in these studies in the CNS such as petechial haemorrhage and small vessel thrombosis [71].
2.4.2.4 Delivery by Barrier Disruption
The temporary physico-chemical disruption of brain endothelial barrier integrity is also considered an invasive strategy for drug delivery, because it typically involves the intracarotid infusion of a barrier-opening agent. Barrier opening for low molecular weight tracers and macromolecules (e.g. Evans blue-albumin) was experimentally demonstrated with intracarotid infusions of membrane active agents such as bile salts, oleic acid, cytostatic drugs etoposide and melphalan, and cytochalasin B. Intracarotid infusion of a low pH buffer also opens the BBB.
Most studies were performed with hyperosmolar solutions. Hypertonic disruption is under clinical evaluation for enhanced delivery of small molecular weight cytostatic agents to brain tumours. Technically, the procedure is performed as a high-flow short-term infusion of 25% mannitol or arabinose under general anaesthesia.The underlying mechanism is a sequelae of endothelial cell shrinkage, disruption of tight junctions and vasodilatation by osmotic shift [72].
Figure 2.7. (a) Autoradiograph of a brain section showing the limited distribution of [125I]-NGF (nerve growth factor) in rat brain 18 h after injection into the lateral ventricle. Exception: cholinergic neurons with retrograde transport to the cell body (arrow). (b) Brain–cerebrospinal fluid (CSF) concentration gradient of four drugs relative to the distance from the ependymal surface. Curves from left to right: BCNU, thiotepa, cytosine arabinoside, hydroxyurea. Drugs were infused by the intracerebroventricular route in a Rhesus monkey for a 1-h period. The gradients are steepest for highly diffusible substances (thiotepa, BCNU). (c) Autoradiograph (top) and unstained photograph (bottom) of coronal sections of rat brains following implantation of [125I]-NGF-loaded polymers. Sections were obtained 2 days postimplanatation. Bar = 2.5 mm. (d) Concentration profile in the vicinity of the polymer implant in (c). (e) The convective-enhanced method allows homogenous delivery to precisely defined volumes, as demonstrated here in a coronal section of monkey brain stained for biotin (black region). The animal received an infusion of biotinylated albumin into the globus pallidus internus (Gpi). Gpe, globus pallidus externus; IC internal capsule; OT, optical tract; Put, Putamen. (f) Calculated concentration profiles for macromolecules in brain that can be achieved with high flow microinfusion. Dashed lines: steady-state profiles of molecules undergoing rapid metabolism with half-lives of 0.167 (A) or 1 h (B). Solid lines: profiles after 2, 6, 10 and 12 h for a molecule with long a half-life (33.5 h). The latter profiles show plateau-like tissue concentrations that extend with duration of infusion up to 1.5 cm from the tip of the infusion catheter. Reproduced with permission from references [61] (a), [65] (b), [66](c,d); [122] (e) and [123] (f).
40 2 Brain-Specific Drug Targeting Strategies
Morphological studies in rats, where the induction of neuropathological changes by osmotic opening was examined, provided evidence of uptake of macromolecules by the brain. The extravasation of plasma proteins such as fibrinogen and albumin was shown immunohistochemically at the light microscopic level. Electron microscopy revealed ultrastructural changes such as swelling of astrocytic processes and severe mitochondrial damage in neurons [73]. There was also evidence of prolonged (24 h) cellular stress or injury in neurons and glia as expressed by the induction of heat shock protein (HSP-70). While the nonspecific opening of the BBB to plasma proteins harbours a risk of eliciting neuropathological changes, osmotic disruption has been tested for its potential as a delivery method for macromolecular drugs such as monoclonal antibodies against various tumour antigens or their Fab fragments. In other studies, uptake after intracarotid administration of nanoparticles (20-nm iron oxide particles) by normal brain, and uptake of recombinant adenovirus or herpes virus by normal brain tissue and brain tumour xenografts in nude rats was postulated [74,75].
Compared to small molecules, barrier opening for high molecular weight compounds is of shorter duration [72]. Furthermore, a characteristic difference exists in the degree of barrier opening in tumour versus normal brain tissue. Barrier disruption was consistently found to be more pronounced for the normal BBB, which may limit the clinical applicability of hyperosmolar barrier opening, at least for cytotoxic drugs [76].
BBB opening may also be achieved by receptor-mediated mechanisms. The vasoactive compounds prostaglandins, histamine, serotonin, leukotriene C4 (LTC4), and bradykinin have all been shown to increase BBB permeability [16]. The effects of LTC4 and bradykinin are more pronounced on the blood–tumour barrier than on the normal BBB. In the case of LTC4 this effect is ascribed to the presence of an enzymatic barrier in normal brain tissue due to the endothelial expression of γ-GTP. The enzyme metabolizes and inactivates LTC4 to LTD4. In contrast, tumour vessels are unable to express equivalent activities of γ-GTP, a fact that may be exploited for selective opening of the tumour barrier by intracarotid administration of LTC4. However, the effect is restricted to small molecules, as there was no increase in the tumour accumulation of a dextran tracer of molecular weight 70 kDa. On the other hand, bradykinin also opens the barrier for the high molecular weight range. It acts on endothelial cells through B2-receptors located on the abluminal side. Normal brain tissue is protected from barrier opening by bradykinin in the vascular lumen because the peptide cannot access these receptors. In tumour vessels the barrier integrity is sufficiently compromised to allow for additional bradykinin-mediated opening at low peptide concentrations. While bradykinin itself requires intracarotid administration, an analogue with prolonged half-life (RMP-7) is effective after intracarotid or intravenous application. A 4–5-fold increase in the delivery of the cytokines interferon-γ , tumour necrosis factor α and interleukin-2 to experimental RG2 glioma in rats was demonstrated after intracarotid infusion of RMP-7 [77]. The drug is currently being evaluated in the therapy of human malignant gliomas to enhance delivery of carboplatin to the tumour [78].
2.4.2.5 Vector-mediated Delivery
In this approach,‘chimeric peptides’ [79] are generated as transportable drug derivatives targeting the receptor-mediated mechanism. Chimeric peptides are formed by linking a drug

2.4 Drug Delivery Strategies |
41 |
that is unable to cross the BBB to a vector (see Figure 2.8). Binding of the vector at the luminal membrane of brain capillary endothelial cells initiates receptor-mediated or adsorp- tive-mediated transcytosis (Figure 2.3c). Size and structure of the cargo may vary as long as binding and cellular uptake of the vector is not inhibited by the drug moiety, and may only be limited by the size of the endocytotic vesicles.
Initial studies of brain delivery based on the chimeric peptide strategy used the absorptivemediated uptake of cationized albumin which was chemically coupled to the opioid peptide β-endorphin [80] or its metabolically stabilized analogue [D-Ala2]β-endorphin. Tracer experiments in which the chimeric peptide was labelled in the endorphin moiety provided evidence of internalization by isolated brain capillaries and transport into brain tissue in vivo [81].
Endogenous ligands for receptor-mediated systems may be unsuitable as vectors due to competition for transport or undesirable pharmacological effects. For example, plasma concentrations of transferrin are in the range of 25 µM. Insulin as a vector would cause hypoglycaemia. A logical alternative as vectors are monoclonal antibodies specific to the extracellular domain of a peptide or protein receptor at the BBB. These antibodies can be designed as non-competitive, i.e. they bind to the receptors at a site distinct from the ligand binding site and do not interfere with the endocytosis process.
Brain uptake data for some vectors are compared in Table 2.1. Quantitative comparisons within the same species are possible for the rat with vectors derived from the anti-TfR monoclonal antibody OX26 and from cationized human serum albumin. To put the efficiency of brain delivery into perspective, the comparison to a classical neuroactive drug may be informative. In the rat, brain concentrations of morphine following systemic administration never exceed 0.08% of injected dose per gram [%ID g–1] [82]. In contrast, OX26 easily reaches concentrations in rat brain that are three to four times higher. Vectors based on cationized hu-
Vector |
Linker |
Drug |
||
|
OX26 |
|
(Strept)avidin - |
Peptides |
|
|
|||
|
|
VIP |
||
|
84-15 |
|
||
|
|
Biotin |
DALDA |
|
|
cHSA |
|
||
|
|
|
Proteins |
|
|
|
|
|
|
|
|
|
|
NGF |
|
|
|
|
BDNF |
|
|
|
|
Oligonucleotides |
|
|
|
|
PNA |
|
|
|
|
Genes |
|
|
|
|
luciferase |
|
|
|
|
-Gal |
|
|
|
|
Liposome |
|
|
|
|
encapsulated drugs |
|
|
|
|
|
Figure 2.8. Scheme of a chimeric peptide with examples for each of the distinct domains. OX26, anti-rat transferrin receptor monoclonal antibody (mAb); 84-15, anti-human insulin receptor mAb; cHSA, cationized human serum albumin; VIP, vasoactive intestinal polypeptide; DALDA, dermorphin analogue; NGF, nerve growth factor; BDNF, brain-derived neurotrophic factor; PNA, peptide nucleic acid; β-gal, β-galactosidase.

42 2 Brain-Specific Drug Targeting Strategies
Table 2.1. Brain concentration, blood–brain barrier PS product, and plasma AUC (0–60 min) of brain delivery vectors after i.v. bolus injection.
Vector (species) |
%ID g-1a |
PS (µl min-1 g-1) |
AUC 0–60 |
|
|
|
(%ID min ml-1) |
|
|
|
|
3H-OX26 (rat) |
0.27 ± 0.04 |
1.92 ± 0.06 |
132 ± 19 |
OX26-Avb (rat) |
0.041 ± 0.004 |
0.85 ± 0.02 |
49 ± 4 |
Anti-TfR IgG3-CH3-Avb,c |
0.25 ± 0.09 |
2.25 ± 0.65 |
134 ± 29 |
(rat, recombinant) |
|
|
|
OX26-NLAb (rat) |
0.17 ± 0.04 |
0.70 ± 0.10 |
232 ± 25 |
OX26-SAb (rat) |
0.20 ± 0.03 |
0.92 ± 0.10 |
216 ± 28 |
cHSA-Avb (rat) |
0.015 ± 0.006 |
0.26 ± 0.13 |
64 ± 7 |
cHSA-NLAb (rat) |
0.061 ± 0.012 |
0.20 ± 0.04 |
300 ± 14 |
125I-8D3 (mouse) |
3.1 ± 0.2 |
3.3 ± 0.1 |
932 ± 57 |
125I-HIR MAb (rhesus monkey) |
3.8 ± 0.4 (100 g brain)d |
5.4 ± 0.6 |
5.9 ± 1.2 (0–180 min) |
aPercentage injected dose per g brain 60 min after i.v. bolus injection.
bThese proteins were labeled at the avidin moiety with [3H]biotin.
cIgG3-CH3 fusion protein with variable region of OX26 and avidin.
d%ID in total brain tissue after 3 h.
AUC, area under curve of plasma concentration; Av, avidin; NLA, neutral avidin; SA, streptavidin; cHSA, cationized human serum albumin; HIR MAb, human insulin receptor mAb; PS product, permeability surface area product.
man albumin reach lower brain concentrations compared to OX26 vectors. The difference is mainly caused by a corresponding difference in the PS products, i.e. the rate of uptake by ab- sorptive-mediated transcytosis of cationized albumin at the BBB is lower than the rate of re- ceptor-mediated uptake of OX26 (Table 2.1).
With regard to transport capacity, the introduction of the anti-human insulin receptor antibody (HIR MAb) 83-14 as a vector indicates the potential for future improvements in brain-specific delivery vectors. Compared to anti-TfR monoclonal antibodies, the brain delivery in primates is over 7-fold higher due to the high PS product of the HIR MAb.
A thorough characterization of vectors for drug delivery needs to take into account the saturable character of receptor-mediated processes and therefore requires investigation of dose dependence of uptake. While non-competing antibodies avoid the problem of competition by endogenous ligands, the saturability of the antibody binding site remains. The values in Table 2.1 were obtained in uptake experiments with doses of vectors in the low g kg–1 range, corresponding to plasma concentrations in the low nM range. Linear pharmacokinetics cannot be expected at higher doses and hence changes in both plasma AUC and apparent PS product are likely to occur at higher doses. Experimental evidence of saturability in vivo was seen for the OX26 antibody in rats and the 8D3 antibody in mice. In the case of 8D3, for example, the brain uptake declined to virtually 0% ID g–1 brain at a dose of 4 mg kg–1 i.v. [83]. Proof of saturability in vivo is direct evidence for a specific uptake mechanism.
The coupling step between vector and drug moiety may be performed by either chemical or molecular biological approaches (see Chapter 11 for a more detailed discussion on conjugation strategies in drug targeting research). While the options offered by chemical methods
2.4 Drug Delivery Strategies |
43 |
provide rapid synthesis of conjugates, which is particularly suitable for animal experiments and ‘proof of concept’ studies, fusion proteins have the potential for bulk production of a defined molecular entity for future clinical development. With regard to chemical conjugation, the avidin–biotin technology as a linker strategy was introduced [84] as a highly versatile alternative to direct linkage of vector and drug moiety [85]. It exploits the broad availability of biotinylating reagents for a range of compounds and functional groups, and a single vector to be used for the delivery of different drugs. Moreover, the avidin–biotin bond is extremely stable. It proved advantageous for pharmacokinetic reasons to substitute the basic avidin with a chemically neutralized form of avidin, designated NLA [86] or with streptavidin (SA) [87].
Recently, a fusion protein between OX26 and avidin was engineered, in which the variable regions of an IgG3-antibody were substituted with those of OX26, and the CH3 region was fused to the avidin monomer (anti-TfR IgG3-CH3-Av). The fusion protein thus forms an avidin dimer, which displays high affinity biotin binding.The pharmacokinetics and brain uptake of the fusion protein were favourable compared to the chemical conjugate of OX26–avidin, as is evident from the values in Table 2.1 [88].
The biotin–avidin linker strategy is particularly suitable for synthetic peptide drugs. These can be designed to facilitate monobiotinylation at a site that does not interfere with bioactivity [89]. Monobiotinylation is recommended due to the multivalency of avidin. A 1 : 1 molar conjugate of vector and (strept)avidin can bind up to four biotin residues, and the genetically engineered fusion protein still has two biotin binding sites.Therefore, higher degrees of biotinylation of the drug moiety would result in the formation of high molecular weight aggregates, which are cleared rapidly from the circulation [90].
Chimeric peptides need to be stable in the circulation before brain uptake occurs, and either amide bonds, thioether or disulfide linkers fulfil that requirement in the plasma compartment. In addition, they must be stable during transcytosis through BBB endothelial cells. Finally they also need to retain binding affinity in their drug moiety. If binding of a peptide drug to the vector reduces binding affinity to the drug receptor on brain cells, then the release of free drug in the brain would be required. Disulfide reducing enzymes such as protein disul- fide-isomerase are present in tissues intracellularly and on the plasma membrane [91]. Cleavage of (-S-S-) linked chimeric peptides in brain in vivo is possible. A biotinylated opioid peptide analogue ([Lys7]dermorphin-amide) with a disulfide biotin linker, N-hydroxysuccin- imide dithiopropionate, was cleaved from the vector OX26-SA in brain but not in plasma [92].
An alternative coupling strategy that avoids potential steric hindrance of drug action and eliminates the need for cleavability utilizes long, flexible spacer arms, e.g. biotin-derivatized polyethylene glycol (PEG) linkers with molecular weights of 2000 or 5000 Da [93,94].
2.4.2.6 Pharmacological Effects of Chimeric Peptides
The cargo that is suitable for transport by chimeric peptides encompasses a wide array of substances. Table 2.2 gives examples of studies, which measured CNS effects after peptide drug delivery.
Among the peptide-based payloads that have been delivered is an analogue of the 28amino acid peptide Vasoactive Intestinal Polypeptide (VIP) [89,95]. VIP is suitable for the