
Recent Progress in Bioconversion of Lignocellulosics
.pdf164 |
N.W.Y. Ho et al. |
1 Introduction . . . . . . . . . . . . . . . . . . . . . . . . . . |
. . . . . . 164 |
2Design of an Ideal Yeast for Effective Cofermentation of Glucose
and Xylose Present in Cellulosic Biomass . . . . . . . . . . . . . . . . |
167 |
3Construction of Recombinant Plasmids Containing XR-XD-XK Three-Gene Cassette Capable of Transforming Saccharomyces Yeasts
|
Able to Effectively Coferment Glucose and Xylose . . . . . . . . . . . |
168 |
4 |
Importance of Cloning the Xylulokinase Gene . . . . . . . . . . . . . |
174 |
5 |
Importance of Making the Genetically Engineered Yeasts Able |
|
|
to Coferment Glucose and Xylose . . . . . . . . . . . . . . . . . . . . |
178 |
6Effective New Integration Method for the Development
of Super-stable Genetically Engineered Saccharomyces Yeasts
|
Containing Multiple Copies of the XR-XD-XK Cassette Integrated |
|
|
into the Yeast Chromosome . . . . . . . . . . . . . . . . . . . . . . . . |
181 |
7 |
Genetically Engineered Saccharomyces Yeasts Able to Coferment |
|
|
Glucose and Xylose Present in Crude Hydrolysates of Various |
|
|
Cellulosic Biomass by Batch or Continuous Process . . . . . . . . . . |
183 |
8 |
Development of Genetically Engineered Saccharomyces Yeasts |
|
|
Able to Coferment Glucose, Xylose, and L-Arabinose . . . . . . . . . |
188 |
9Further Improvement of Glucose-Xylose-Cofermenting Saccharomyces Yeasts for Effective, Economical Conversion
of Sugars from Cellulosic Biomass to Biofuel Ethanol . . . . . . . . |
. 189 |
10 Concluding Remarks Particularly on the Importance of Using |
|
Safe and Effective Microorganisms for Biofuel Ethanol Production |
. 191 |
References . . . . . . . . . . . . . . . . . . . . . . . . . . . . . . . . . . . . 192
1 Introduction
Numerous studies have proven that ethanol as a transportation fuel produces far less air pollutants than gasoline, and particularly since it contributes no net carbon dioxide to the atmosphere. This environmentally friendly liquid fuel can be used directly as a neat fuel (100%) or as a blend with gasoline at various concentrations.The raw material used for the production of ethanol fuel is renewable and abundantly available domestically in most countries. Thus, the use of ethanol to supplement or replace gasoline not only reduces air pollution, ensures a cleaner environment, and eases the threat of global warming, but also reduces the dependency of many nations on imported foreign oil and protects their energy security.
Successful Design and Development of Genetically Engineered Saccharomyces Yeasts |
165 |
Ethanol has traditionally been and is currently still produced from glucosebased food crops, such as cane sugar, corn starch, and other starch-rich grains, via fermentation of glucose present in these feedstocks by Saccharomyces yeasts. However, these agricultural crops are expensive and in limited supply.
More than twenty years ago, it was recognized that cellulosic biomass, including agricultural residues (such as corn stover, rice and wheat straws, and sugarcane bagasse), municipal wastes (such as yard and paper wastes), and industrial wastes (such as wastes from paper mills), is an attractive feedstock for ethanol-fuel production by fermentation because cellulosic biomass is not only renewable and available domestically in most countries but also available at very low cost and in great abundance.
However, there are problems that must be solved before such ideal feedstocks can be economically converted to ethanol. One serious problem has been that the Saccharomyces yeasts traditionally used and still the most effective microorganisms for fermenting glucose-based feedstocks to ethanol, are found to be unable to effectively ferment all the major sugars from cellulosic biomass to ethanol. This is because the major sugars derived from cellulosic biomass are not just glucose but also xylose with a ratio of glucose to xylose being approximately 2 or 3 to 1. It is generally agreed that unless both glucose and xylose from the cellulosic biomass can be fermented, the economics of converting cellulosic biomass to ethanol is unfavorable. However, nearly all of the fermentative Yeasts, including the Saccharomyces, are found to be unable to ferment xylose to ethanol or utilize the pentose sugar for growth. This is because these yeasts are missing certain enzyme(s) that are responsible for the conversion of xylose to ethanol. Thus, up to 1993, the major obstacle that prevented cellulosic biomass from being economically converted to ethanol had been the lack of safe and effective microorganisms, particularly the Saccharomyces yeasts, that can effectively and efficiently convert both glucose and xylose to ethanol.
In order to solve the above described problem, lots of research efforts, particularly in the period between 1970 to 1980, were devoted to searching for new yeasts, especially the Saccharomyces yeasts, that might be able to effectively ferment both glucose and xylose to ethanol. However, no such Saccharomyces yeasts were found. Nevertheless, a few other yeasts such as Pichia stipitis,
Candida shehatae, and Pachysolen tannophilus were found to be able to ferment xylose to ethanol and also able to utilize the sugar for growth. Unfortunately, these naturally occurring xylose-fermenting yeasts have severe drawbacks that limit their commercial applicability [1].
Yeasts and bacteria metabolize xylose by following slightly different pathways as showing in Fig. 1. Yeasts rely on xylose reductase and xylitol dehydrogenase, but bacteria rely on xylose isomerase, to convert xylose to xylulose [2, 3]. Although the Saccharomyces yeasts as well as other fermentative yeasts are not able to ferment xylose, Saccharomyces yeasts are able to ferment xylulose to ethanol [4]. Furthermore, they are also able to ferment xylose when a bacterial xylose isomerase is present in the medium [5]. This indicates that Saccharomyces yeasts lack only the enzymes for the conversion of xylose to xylulose.
More than 15 years ago, nearly ten groups worldwide including our group – the then newly established Molecular Genetics Group at the Laboratory of

166 |
N.W.Y. Ho et al. |
Fig. 1. The xylose metabolic pathways in various microorganisms
Renewable Resources Engineering of Purdue University – were all attempting to transfer (clone) a bacterial xylose isomerase gene into various species of Saccharomyces yeasts to develop genetically engineered yeasts for fermenting xylose (Fig. 1). However, all those earlier efforts failed to produce a genetically engineered yeast that could ferment xylose [6–10].
After the unsuccessful attempts to clone a xylose isomerase gene in yeast, we were one of the remaining four known groups that continued to pursue this dif-
Successful Design and Development of Genetically Engineered Saccharomyces Yeasts |
167 |
ficult task.Although all the groups now turned to clone the xylose reductase (XR) and xylitol dehydrogenase (XD) genes from P. stipitis to provide the Saccharomyces yeasts the capability of converting xylose to xylulose [11–14], there are major differences between our approach and those of the three other groups in the design and development of the ideal yeast for fermenting the cellulosic sugars (fermentable sugars derived from cellulosic biomass) [15]. To the best of our knowledge, we are the only group even to this date that has succeeded in genetic engineering of the Saccharomyces yeasts for effective fermentation of xylose to ethanol. In addition, our genetically engineered yeasts can also effectively coferment simultaneously both glucose and xylose present in the same medium to ethanol [15]. The latter property proves to be extremely critical for yeast to be effective for fermenting both major cellulosic sugars to ethanol. In this paper,we report our rationales and strategies as well as our methods and approaches that led to the ingenious design and successful development of our genetically engineered yeasts for effective fermentation of glucose and xylose to ethanol.
2
Design of an Ideal Yeast for Effective Cofermentation of Glucose and Xylose Present in Cellulosic Biomass
The uniqueness of our approach is that we have taken considerations in our design not simply to engineer a yeast to be able to ferment xylose but also to be able (1) to effectively direct the carbon flow towards the production of ethanol rather than the production of byproducts such as xylitol, (2) to effectively coferment both glucose and xylose simultaneously so that the mixed sugars will be fermented as fast as possible, (3) to easily convert most Saccharomyces strains, particularly the superior glucose-fermenting industrial strains to coferment xylose in addition to glucose, and (4) to use rich medium for growth and fermentation to make the engineered yeast grow and ferment sugars faster and to solve the potential waste problems by recycling used yeast cells for the production of crude yeast extracts for culturing new yeast cells. Furthermore, the final genetically engineered yeasts should be stable and able to use either batch or continuous process for ethanol production without requiring the use of any special chemicals functioning as the selection pressure to maintain the cloned genes.
In order to accomplish all those described above, we believed that we needed not only to clone a xylose reductase gene (XR) and a xylitol dehydrogenase gene (XD) but also to clone a third gene, the xylulokinase gene (XK), even though all the Saccharomyces yeasts do contain a functional XK. Furthermore, we also needed to replace the signal sequences that controls the expression of the three cloned genes with the sequences that control the expression of yeast glycolytic genes. We expected that an engineered Saccharomyces yeast containing sufficient copies of the above described three genes should be able not only to effectively ferment xylose but also to effectively coferment both glucose and xylose present in the same medium.
168 |
N.W.Y. Ho et al. |
3
Construction of Recombinant Plasmids Containing XR-XD-XK Three-Gene Cassette Capable of Transforming Saccharomyces Yeasts Able to Effectively Coferment Glucose and Xylose
According to our plan, we first synthesized the promoterless structural genes of XR [16] and XD [17] from Pichia stipitis chromosomal DNA and the XK gene [18, 19] from S. cerevisiae DNA by PCR techniques. The promoterless structural genes of XD and XK were then fused to the promoter of S. cerevisiae pyruvate kinase gene [20] by the technique of site specific mutagenesis and the resulting recombinant genes were designated as KD and KK. The promoterless structural gene of XR was fused to the promoter of S. cerevisiae alcohol dehydrogenase gene [21] by the same technique and the resulting fusion was designated as AR or A*R . The 5¢ control region of AR consisted of the original 5¢ noncoding sequence of P. stipitis XR from –1 to –50, followed by the S. cerevisiae ADC1 5¢ control region (–14 to –1500 noncoding sequence) (ADC1 promoter fragment cloned on pMA56) [22]. Because it was uncertain what effect the nucleotide sequence of –1 to –50 from P. stipitis XR might have on the expression of XR in Saccharomyces yeasts, the A*R gene was also constructed, in which the 5¢ control region of XR was totally replaced by the intact 5¢ control region of the ADC1 gene (–1 to –1500 noncoding sequence) [21].
Since we might need to clone the three xylose-metabolizing genes ( referred to below as the XYL genes) on different plasmids, for the convenience of the subsequent work we first cloned the three XYL genes on the E. coli pBluescript KS (–) [23] (referred to below as pKS) in such a way that the three genes would form a cassette which could be excised from the pKS plasmid together as a fragment by a single digestion with restriction enzyme Xho I. As a result, the threegene cassette could be easily inserted together into various yeast-E. coli shuttle plasmids for simultaneous expression of all three genes in yeasts. Totally four such plasmids were constructed and they were designated as pKS-KK-A*R-KD- 1, pKS-KK-A*R-KD-2, pKS-KK-AR-KD-3, and pKS-KK-AR-KD-4 (referred to below as pKRD-1,-2,-3, and –4, respectively) [15]. The four plasmids were different only in their AR gene orientation and in the promoter of the AR gene described above. Two such plasmids are shown in Fig. 2.
Although our goal was eventually to integrate sufficient copies of the XYL gene cassette into the yeast chromosome, we planned first to clone the XYL gene cassette into a high copy number 2m-based yeast – E. coli shuttle plasmid. This would allow us to test our hypothesis, particularly whether the cloning of the three genes would make it possible to convert many Saccharomyces yeasts being unable to ferment xylose to recombinant yeasts that are not only able to effectively ferment xylose, but also able to simultaneously coferment both glucose and xylose to ethanol effectively.
We chose first to clone the XhoI restriction fragments containing the XYL gene cassette from pKRD-1,-2,-3,-4 into the SalI site of the 2m-based pUCKm10 yeast -E. coli shuttle plasmid, resulting in the construction of pLNH-31,-32,-33, and –34 (Fig. 3) [15]. These plasmids were collectively designated as the pLNH

Successful Design and Development of Genetically Engineered Saccharomyces Yeasts |
169 |
Fig. 2. The E. coli plasmids, pKRD-2 and pKRD-3, containing the three xylose-metabolizing gene cassette, KK-AR (or A*R)-KD
Fig. 3. The yeast-E. coli shuttle plasmids containing the three xylose-metabolizing gene cassette, KK-AR (or A*R)-KD) [15]

170 |
N.W.Y. Ho et al. |
Fig. 4. Fermentation of glucose and xylose by genetically engineered Saccharomyces yeast strain 1400 (pLNH32) [15]
plasmids. These four plasmids were used to transform various Saccharomyces yeasts. The yeast transformants were initially selected by their resistance to certain levels of antibiotic geneticin and by their ability to form a halo on the ampicillin test plate [15].Yeast transformants containing any one of the four pLNH plasmids can effectively coferment both glucose and xylose to ethanol as shown in Figs. 4 and 5. On the contrary, the parent yeast that does not contain one of the pLNH plasmids can only ferment glucose but not xylose to ethanol as shown in Fig. 6.

Successful Design and Development of Genetically Engineered Saccharomyces Yeasts |
171 |
Fig. 5. Fermentation of glucose and xylose by genetically engineered Saccharomyces yeast strain 1400 (pLNH33)
In searching for an ideal selection system to maintain the xylose-fermenting capability of the plasmid-mediated transformants such as 1400 (pLNH32) or 1400 (pLNH33) without relying on expensive or toxic chemicals such as antibiotics, we discovered that most Saccharomyces yeasts are unable to grow on rich medium such as YEP (1% yeast extract, 2% peptone) without the presence of a carbon source (Fig. 7). This allows the genetically engineered yeasts to maintain their plasmids in YEPX (YEP with 2% xylose) medium without the use of antibiotic geneticin [15]. This discovery has made it possible for our en-
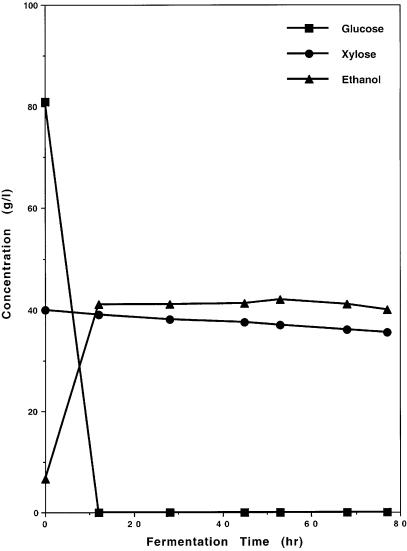
172 |
N.W.Y. Ho et al. |
Fig. 6. Fermentation of glucose and xylose by the unengineered parent Saccharomyces yeast strain 1400 [15]
gineered yeasts to grow in rich medium (YEPX) without losing their xylose-fer- menting capability. Since the pLNH plasmids are high-copy-number plasmids, this also allows our engineered Saccharomyces yeasts cultured in YEPX during early stage(s) of growth to be cultured in YEPD for the last stage of growth before fermentation to take place as described in the captions of Figs. 4 and 5.
We also found that each plasmid can transform the same yeast strains to coferment glucose and xylose with approximately the same efficiencies. For ex-

Successful Design and Development of Genetically Engineered Saccharomyces Yeasts |
173 |
Fig. 7. Culturing of yeast Saccharomyces cerevisiae strain AH22 in rich medium YEP (yeast extract and peptone) with a carbon source (Glucose) or YEP alone without a carbon source (glucose) [15]
ample, the difference in efficiency between 1400 (pLNH31), 1400 (pLNH32), 1400 (pLNH33), and 1400 (pLNH34) in cofermenting glucose and xylose to ethanol is not more than 5–10% as shown in Figs. 4 and 5. This indicates that both the orientation of AR, KD, and KK on the plasmid and the promoter of AR or A*R do not affect much the efficiency of the yeast transformants in cofermenting glucose and xylose.