
Recent Progress in Bioconversion of Lignocellulosics
.pdf
174 |
N.W.Y. Ho et al. |
Table 1. Comparison of the growth of recombinant Saccharomyces and the parent yeast in glucose and xylose media. The units used are Klett Units, i.e. the optical density units measured by a Klett-Surnmerson photoelectric colorimeter
Strains |
Medium |
Time (h) |
|
|
|
|
|
|||
|
|
|
|
|
|
|
|
|
|
|
|
|
|
0 |
2 |
4 |
6 |
8 |
11 |
15 |
|
|
|
|
|
|
|
|
|
|
||
1400(pLNH33) |
YEPDa |
21 |
22 |
44 |
120 |
289 |
455 |
472 |
||
|
|
YEPXb |
18 |
21 |
37 |
68 |
122 |
500 |
550 |
|
1400(LNH-ST) |
YEPD |
17 |
19 |
35 |
87 |
205 |
400 |
425 |
||
|
|
YEPX |
17 |
18 |
30 |
59 |
116 |
482 |
520 |
|
Parent Yeast |
YEPD |
17 |
17 |
39 |
120 |
290 |
433 |
460 |
||
|
|
YEPX |
54 |
66 |
72 |
75 |
75 |
79 |
79 |
|
|
|
|
|
|
|
|
||||
a |
1% yeast extract, 2% peptone, 2% glucose. |
|
|
|
|
|
||||
b |
Same as a except 2% xylose instead of glucose. |
|
|
|
|
|
By testing our 1400 (pLNH32) or 1400 (pLNH33), several groups have confirmed that our genetically engineered yeasts can effectively coferment glucose and xylose simultaneously as we reported above [24,25]. In particular, Moniruzzaman et al. [24] have shown that our engineered yeast ferments both glucose and xylose effectively under anaerobic conditions in a well controlled fermenter. Since our fermentations were carried out in shaker flasks sealed with Saran Wrap and limited amounts of oxygen were introduced to the fermentation flasks during sampling and possibly also during fermentation, the results of Moniruzzaman et al. demonstrate that our engineered yeast does not require the presence of oxygen in order to ferment xylose.
In addition, our genetically engineered yeasts can also very efficiently utilize xylose for aerobic growth as shown in Table 1. Furthermore, as we expected, the pLNH plasmids can transform most of the Saccharomyces yeasts to be able to effectively coferment glucose and xylose to ethanol. However, it may require adjusting the level of geneticin to boost the copy number of the pLNH plasmid in some of the Saccharomyces strains in order to achieve the optimal results in cofermenting glucose and xylose as shown in Fig. 8. Nevertheless, this does not affect the development of stable glucose-xylose-cofermenting strains containing multiple copies of the integrated XYL gene cassettes in the yeast chromosome as described below.
4
Importance of Cloning the Xylulokinase Gene
One of the major differences between our genetically engineered Saccharomyces yeasts and those reported by others (Kotter et al. [11], Walfridsson et al. [12] and Tantirungkij et al. [13] is that in addition to cloning the XR and XD genes, we also cloned the XK genes, even though nearly all Saccharomyces yeasts have an active xylulokinase gene and produce an active xylulokinase enzyme in the presence of xylose. As a result, our genetically engineered yeast can effec-
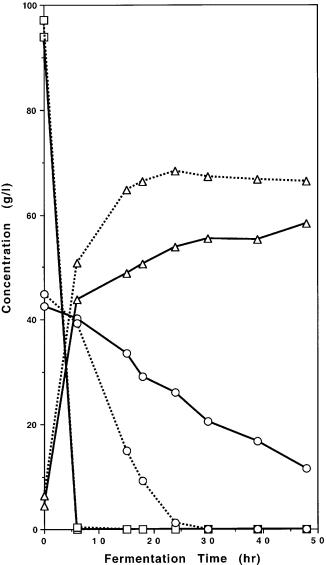
Successful Design and Development of Genetically Engineered Saccharomyces Yeasts |
175 |
Fig. 8. Fermentation of glucose and xylose by 259A(pLNH32) in the presence (100 mg/ml) (dotted line) or absence of geneticin (solid line). Symbols: square glucose; circle xylose; triangle ethanol
tively utilize xylose for growth and also can effectively ferment xylose to ethanol. On the contrary, those developed by Kotter et al. [11] and Walfridsson et al. [12] are neither able to utilize xylose for growth nor able to ferment xylose to ethanol. Although Tantirungkij et al. [13] reported that their engineered yeast can grow with xylose as the sole carbon source, their yeast still cannot ferment
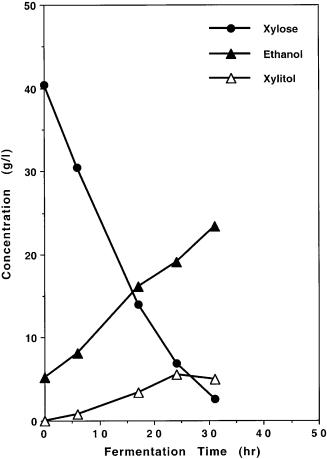
176 |
N.W.Y. Ho et al. |
xylose to ethanol. We believed that the cloning and overexpression of the xylulokinase gene is extremely important in order to make yeasts ferment xylose effectively, particularly to ferment both glucose and xylose simultaneously when these two sugars are present in the same medium. In our view, cloning and overexpression of the xylulokinase gene contributed at least the following for making the Saccharomyces yeast capable of effective cofermentation of glucose and xylose:
(1) Most Saccharomyces yeasts have very low levels of xylulokinase activity [26]. Cloning and overexpression of a xylulokinase gene in the Sac-
A
Fig. 9A, B. Comparison of fermentation of xylose under identical conditions by A genetically engineered Saccharomyces yeast strain 1400(pLNH32) which contains the cloned and genetically modified XR, XD, and XK genes and by B 1400 (pXR-XD) which contains only the same cloned XR and XD genes, but not the cloned XK gene. These results demonstrate the importance of cloning the XK gene to enable the Saccharomyces yeasts such as 1400 (pLNH32) to ferment xylose to ethanol

Successful Design and Development of Genetically Engineered Saccharomyces Yeasts |
177 |
B
Fig. 9B (Continued)
charomyces yeasts will provide the necessary high levels of xylulokinase activity for effective metabolizing of xylose either for growth or for the production of ethanol anaerobically .
(2)The Pichia xylitol dehydrogenase gene chosen by us to be cloned into the Saccharomyces yeast is known to produce a xylitol dehydrogenase that catalyzes a reversible reaction between xylitol and xylulose as shown in Fig. 1 but favors the formation of xylitol rather than xylulose. Thus, an extremely strong xylulokinase activity will help to direct the carbon flux towards the production of ethanol rather than the formation of byproduct xylitol.
178 |
N.W.Y. Ho et al. |
(3)According to our plan, we must alter the genetic signals controlling the expression of any genes in yeasts that will be inhibited by the presence of glucose (catabolic repression) and also require the presence of xylose for induction. The promoter of the yeast xylulokinase gene falls into these categories and therefore we must replace it with another promoter whose function will not be affected by the presence of glucose or by the absence of xylose. Thus, even a yeast containing a very effective xylulokinase gene and producing high levels of xylulokinase still needs to replace its promoter with a glycolytic promoter.
To demonstrate the effect of cloning XK on metabolizing xylose by the Saccharomyces yeast, we constructed a new plasmid pUCKm10-XR-XD which is identical with pLNH33 except without the cloned XK gene. Saccharomyces strain 1400 transformants containing pUCKm10-XR-XD utilize xylose for growth at much slower rates, less than one 15th of the rate of the same yeast strain containing pLNH33. Furthermore, the genetically engineered 1400 yeast containing pUCKm10-XR-XD, designated 1400(pXR-XD), only can convert xylose to xylitol, but not ethanol as compared in Fig. 9A, B.
5
The Importance of Making the Genetically Engineered Yeasts Able to Coferment Glucose and Xylose
Nearly all naturally occurring microorganisms including the Saccharomyces yeasts, only metabolize glucose when a mixture of sugars, such as glucose and xylose, are present in their media. Furthermore, microorganisms also require to induce new enzymes to use other specific sugars, for example xylose, after glucose is depleted from their media. Thus, there usually is a long lag period after glucose has been depleted before the second sugar can be actively used by a microorganism and this phenomenon is generally known as the “glucose effect”. In our view, the time for microorganisms, including yeasts, to convert a feedstock containing mixed sugars to ethanol would be much shortened if the microorganisms could be made free from the glucose effect and also not require the presence of xylose for the induction of the xylose-specific enzymes. We believed that this could be made by replacing the genetic signals controlling gene expression present within the 5¢ noncoding sequences of those genes specifically required for the metabolism of xylose. Besides the XR and XD genes, the xylulokinase gene is the third gene also subject to the control of the glucose effect and also requiring the presence of xylose for induction of the synthesis of its protein. Thus, this is the third reason mentioned above that we must also clone the xylulokinase gene even if the parent yeasts contain high levels of xylulokinase activity.
In order to prove that cloning KD, AR or A*R, and KK (XD, XR, and XK structural genes fused to glycolytical promoters) is extremely important for cofermentation of feedstocks (or media) containing mixtures of glucose and xylose, we compared the fermentation of a mixture of glucose and xylose by 1400 (pLNH 32) and Pichia stipitis. As described above, the Pichia yeast is a na-
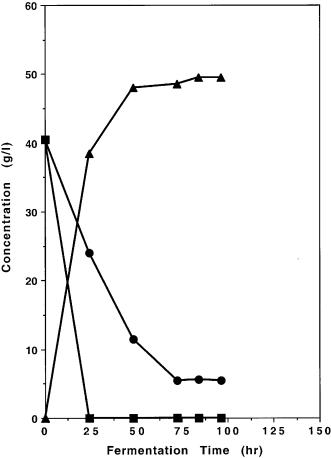
Successful Design and Development of Genetically Engineered Saccharomyces Yeasts |
179 |
turally occurring xylose-fermenting yeast that can ferment xylose with similar efficiency at the laboratory scale under well controlled conditions as the genetically engineered 1400 (pLNH32) except that the expression of Pichia’s XD, XR, and XK genes is subjected to glucose inhibition and also requires the presence of xylose for induction. As shown in Fig. 10A, B, when these yeasts were cultured in YEPX and used to ferment a mixture of glucose and xylose, 1400 (pLNH32) can effectively coferment both glucose and xylose to ethanol and P. stipitis can ferment only glucose to ethanol but not xylose. Furthermore, our engineered yeast cultured in 50 ml YEPD medium with 2 ml of YEPX pregrown cells served as the seed culture is also able to ferment a mixture of glu-
A
Fig. 10A, B. Comparison of cofermentation of glucose and xylose present in the medium under identical conditions by A genetically engineered Saccharomyces yeast strain 1400 (pLNH32) and by B Pichia stipitis. These results demonstrate that our genetically engineered 1400(pLNH32) can effectively coferment glucose and xylose to ethanol but not P. stipitis. Symbols: square glucose; circle xylose; triangle ethanol
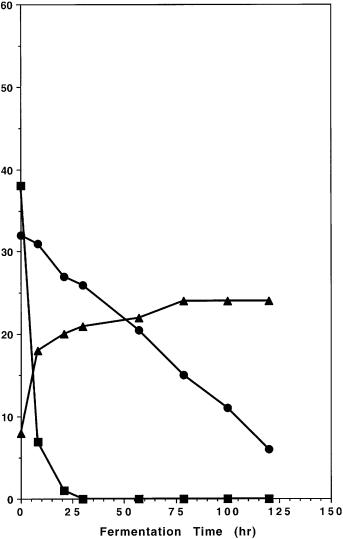
180 |
N.W.Y. Ho et al. |
B
Fig. 10B. (Continued)
cose and xylose to ethanol with similar efficiency as shown in Fig. 9. Thus, our results demonstrated that it is absolutely essential to make a microorganism, for example a Saccharomyces yeast, able to synthesize xylose-metabolizing enzymes in the presence of glucose in order for a microorganism (yeast) to be effective in cofermenting both glucose and xylose to ethanol.
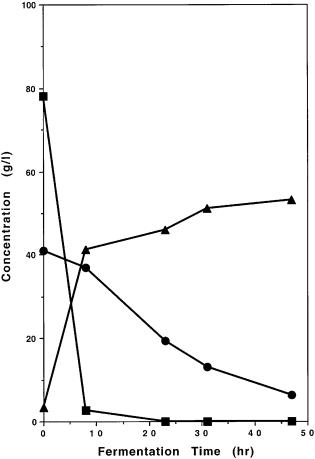
Successful Design and Development of Genetically Engineered Saccharomyces Yeasts |
181 |
6
Effective New Integration Method for the Development of Super-stable Genetically Engineered Saccharomyces Yeasts Containing Multiple Copies of the XR-XD-XK Cassette Integrated into the Yeast Chromosome
Even though 1400(pLNH32) and related plasmid-mediated genetically engineered xylose-fermenting Saccharomyces yeasts are very stable and their xy- lose-fermenting ability can be maintained by an ideal selection mechanism, a perfect yeast for fuel-ethanol production should be completely stable without the need for using selection pressure to maintain its xylose-fermenting ability at any stage of growth or fermentation. In 1995, we successfully developed the first super-stable strain of genetically engineered glucose-xylose-cofermenting Saccharomyces yeast, 1400 (LNH-ST), which is completely stable without the
Fig. 11A. Comparison of fermentation of glucose and xylose under identical conditions by A genetically engineered Saccharomyces yeast strain 259A(LNH-ST)-2 and by …
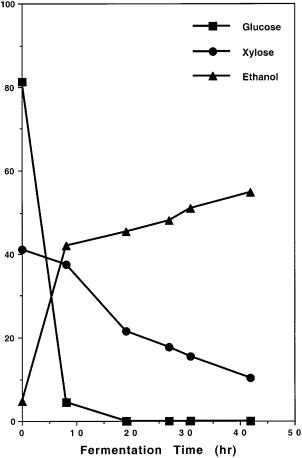
182 |
N.W.Y. Ho et al. |
Fig. 11B. (Continued) B 259A(LNEI-ST)-2 after being cultured in non-selective medium (YEP+glucose) for more than 50 generations. These results demonstrate that the Saccharomyces yeast strain 259A(LNH-ST)-2 is stable and retain its ability to effectively ferment xylose without relying on the presence of any selection pressure. On the contrary, genetically engineered yeast 259A(pLNH32), with the xylose metabolizing genes cloned on the high copy number plasmid pLNH32, will lose its ability to ferment xylose after being cultured in the same non selective medium for even just 20 generations (data not shown). Also note that 259A(LNH-ST)-2 is one of the 259A strain containing the integrated xylose metabolizing genes that was chosen to carry out these studies but not the one that is most effective for fermenting xylose. Symbols: square glucose; circle xylose; triangle ethanol
need to apply any selection pressure to maintain its ability to ferment xylose to ethanol (data not shown) or utilize the sugar for growth as shown in Table 1. Furthermore, these superstable xylose-fermenting genetically engineered yeasts also coferment glucose and xylose to ethanol as effectively as or better than the plasmid-mediated recombinant yeasts such as 1400 (pLNH32) (data
Successful Design and Development of Genetically Engineered Saccharomyces Yeasts |
183 |
not shown). These superstable genetically engineered Saccharomyces yeasts contain multiple copies of the same three gene cassette integrated into the host chromosome. Also our superstable xylose-fermenting yeast was developed by using a much improved new method for integrating multiple copies of genes into the yeast chromosome. This method for integrating multiple copies of gene(s) into the host chromosome is easy to accomplish and more importantly, it is also easy to control the copy-number of the integrated genes. Up to now, we have completed the development of three stable glucose-xylose-cofermenting Saccharomyces yeast strains 1400 (LNH-ST), 259A (LNH-ST), and 424A(LNHST) derived from three different parent strains of Saccharomyces yeasts. We are in the process of developing several more such stable glucose-xylose-coferment- ing yeast strains, including strains derived from special yeasts used by industry for the production of ethanol from starch or wood hydrolysates. Figure 11A,B demonstrate that the genetically engineered 259A (LNH-ST) cultured in nonselective medium for more than 50 generations still can ferment xylose as effective as 259A (LNH-ST) that has not been cultured in non-selective medium. The development of these stable genetically engineered xylose-fermenting Saccharomyces yeasts and the method of integrating multiple copies of gene(s) into the yeast chromosome have been briefly described previously [27] and the details in the development of these yeasts will be reported elsewhere.
7
Genetically Engineered Saccharomyces Yeasts Able to Coferment Glucose and Xylose Present in Crude Hydrolysates of Various Cellulosic Biomass by Batch or Continuous Process
Our genetically engineered Saccharomyces yeasts also can ferment glucose and xylose present in crude cellulosic hydrolysates very effectively as demonstrated in Figs. 12–15. The crude hydrolysates were prepared by different processes and provided to us by others. The hydrolysates were used directly for fermentation without any treatments to remove toxic inhibitors normally present in most hydrolysates. For fermentation, pregrown yeast cells were added to the hydrolysates to a cell density similar to those used for fermentation of pure sugars shown in Figs. 4 and 5. Yeast extracts were added to the hydrolysates to a final concentration of 1% and the pH of the hydrolysates was adjusted to 7.0 prior to fermentation but without further adjustment during fermentation. However, effective fermentation of both glucose and xylose was also obtained with our genetically engineered yeasts by initially adjusting the pH of the hydrolysate to 5.5 and by supplementing only 0.2% yeast extract for fermentation (data not shown). Independent studies were carried out by Moniruzzaman et al. to use 1400 (pLNH32 ) for fermenting sugars from hydrolysates of corn fiber and excellent results were also obtained [24].
The stable strain 1400 (LNH-ST) was confirmed to be able to effectively coferment glucose and xylose by a continuous process in a 9000-L pilot scale fermentor with both pure sugars and sugars from crude corn biomass hydrolysates as the feedstocks [25].