
Cundari Th.R. -- Computational Organometallic Chemistry-0824704789
.pdf98 |
Diedenhofen et al. |
FIGURE 3 Continued
not precisely known. Sunderlin and Squires (74) recently carried out gas-phase experiments that made it possible to obtain thermochemical data for selected steps of the reaction cycle depicted in Figure 4. The published data are ideally suited for comparison with theoretical results, because the experimental values of the reaction energies and activation barriers refer to processes in the gas phase.
Torrent et al. optimized the geometries of the intermediates and transition states sketched in Figure 4 at the B3LYP/II level of theory (72). Single-point energies were then calculated at B3LYP and CCSD(T) levels using the larger
QM Methods for Calculating TM Compounds |
|
|
|
|
99 |
|||||
TABLE 13 Theoretically Predicted Bond Dissociation Energies De (kcal/ |
||||||||||
mol) of the P–S and TM–NH3 Bonds |
|
|
|
|
|
|
||||
|
|
|
|
|
|
|
|
|
|
|
|
|
|
|
De(S) |
|
|
|
|
De (NH3) |
|
|
|
|
|
|
|
|
|
|
|
|
Compound |
No. |
HF |
MP2 |
B3LYP |
CCSD(T)a |
|
HF |
MP2 |
B3LYP |
CCSD(T)a |
|
|
|
|
|
|
|
|
|
|
|
[Mo(PS)(NH2)3] |
3 |
28.2 |
34.6 |
57.9 |
47.6 |
|
— |
— |
— |
— |
[W(PS)(NH2)3] |
4 |
19.8 |
37.4 |
50.3 |
42.2 |
|
— |
— |
— |
— |
[Mo(P)(NH2)3(NH3)] |
5 |
— |
— |
— |
— |
8.6 |
10.3 |
7.2 |
15.8 |
|
[W(P)(NH2)3(NH3)] |
6 |
— |
— |
— |
— |
10.5 |
16.0 |
8.9 |
17.4 |
|
[Mo(PS)(NH2)3(NH3)] |
9 |
41.2 |
53.9 |
71.6 |
62.9 |
21.6 |
29.6 |
22.8 |
31.2 |
|
[W(PS)(NH2)3(NH3)] |
10 |
33.2 |
56.0 |
66.4 |
58.5 |
24.0 |
32.4 |
24.9 |
33.7 |
|
|
|
|
|
|
|
|
|
|
|
|
a Using B3LYP/II optimized geometries. Source: Ref. 65.
basis set II , which has additional diffuse s and p functions on hydrogen, carbon, and oxygen. The diffuse functions turned out to be crucial for the accuracy of the theoretically predicted energies, because some of the calculated species are anions. Table 14 shows the calculated and experimental reaction energies and activation barriers.
The data listed in Table 14 show that the calculated reaction energies at 298 K at the CCSD(T)//II level after zero-point energy (ZPE) and thermal corrections are in excellent agreement with the experimental values. The B3LYP/ II values show larger differences from the experimental values, but the relative reaction energies correspond with the observed data. Note the dramatic effect of the diffuse functions on the B3LYP reaction energies of reactions 2, 6, and 7. The theoretical values at B3LYP/II for reactions 2 ( 61.8 kcal/mol) and 7 (70.3 kcal/mol) are in perfect agreement with experiment, while the B3LYP/ II values have errors of 30 kcal/mol. The very large effect of the diffuse functions on the calculated reaction energies in reactions 2, 6, and 7 arises because the negative charge is constrained on OH on the adduct (reaction 2) or product (reactions 6 and 7) side of the reaction, while it is delocalized over the iron complex on the other side of the reaction. Note that the theoretically predicted activation barrier for the reaction 12a → 13 CO2, which is nearly the same at the three levels of theory, is also in reasonable agreement with experiment.
Olefin Addition to Transition Metal Oxides: [2 2]
Versus [3 2] Cycloaddition
The question whether the addition of TM oxides to olefins proceeds as a [3 2] cycloaddition yielding a dioxylate or via a [2 2] addition forming a metallaoxetane, which then may rearrange to the dioxylate (Scheme 1), has been
100 |
Diedenhofen et al. |
FIGURE 4 Calculated reaction steps in the water-gas shift reaction. (From Ref. 72.)
the topic of several theoretical studies. Most investigations focused on the osmylation reaction (74–80). The unequivocal answer to our question was that the barriers for the two-step mechanism are much higher than for the [3 2] addition (76–80). A recent theoretical study by Deubel and Frenking also investigated the addition of LReO3 (L O , Cl, Cp) to ethylene (81). The results were compared with theoretical data for the osmylation reaction using different basis sets.
Table 15 shows that the B3LYP energies with basis set III are in some cases considerably different from the B3LYP/II values. The largest differences are found for the relative energy of the dioxylate with respect to TM oxide and ethylene. The B3LYP/III values for the relative energy of the dioxylate are
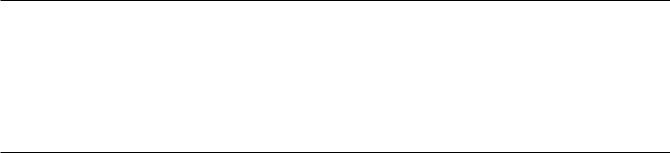
TABLE 14 Calculated and Experimental Reaction Energies and Activation Barriers ∆E (in kcal/mol) for Some |
|||||
Reactions Related to the Catalytic Cycle of the WGSR |
|
|
|
||
|
|
|
|
|
|
No. |
Reaction |
∆E (B3LYP/II) |
∆E (B3LYP/II ) |
∆E (CCSD(T))/II ) |
Experimental |
1 2 3 4 4TS 5 6 7 8 9 10 11
CO H |
O |
→ CO |
2 |
H |
2 |
|
|
|
|
|
|
|
|
||||||
|
2 |
|
|
|
|
|
|
|
|
|
|
|
|
|
|
|
|
||
Fe(CO) (11) OH |
|
→ (CO) |
|
|
|
|
|
(12a) |
|
||||||||||
|
FeCOOH |
|
|||||||||||||||||
|
5 |
|
|
|
|
|
|
|
|
|
4 |
|
|
|
|
|
|
|
|
(CO) |
|
|
|
|
(12a) |
→ (CO) |
|
|
|
|
|
(12b) |
|||||||
FeCOOH |
|
FeOCOH |
|||||||||||||||||
4 |
|
|
|
|
|
|
|
|
|
|
|
4 |
|
|
|
|
|
|
|
(CO) |
FeCOOH |
|
(12a) |
|
→ (CO) |
FeH |
|
(13) |
CO |
2 |
|||||||||
|
|
|
|||||||||||||||||
4 |
|
|
|
|
|
|
|
|
|
|
|
4 |
|
|
|
|
|
|
|
TS (12a → |
13 CO |
) |
|
|
|
|
|
|
|
|
|
|
|||||||
|
|
|
|
|
|
|
|
2 |
|
|
|
|
|
|
|
|
|
|
|
(CO) |
FeH |
|
(13) |
|
|
||||
4 |
|
|
|
|
(CO) |
FeH |
|
H |
O |
|
||||
4 |
|
|
2 |
|
(CO) |
FeH |
|
(13) |
|
|
||||
4 |
|
|
|
|
(CO) |
FeH |
2 |
(15) |
|
4 |
|
|
|
|
(CO) |
FeH |
2 |
(16) |
|
4 |
|
|
|
|
(CO) |
FeH |
2 |
(15) |
|
4 |
|
|
|
|
Fe(CO) (17) |
||||
|
4 |
|
|
|
H2O → (CO)4FeH H2O (14) → (CO)4FeH2 (15)
H2O → (CO)4FeH2 (15)
→(CO)4FeH2(16)
→Fe(CO)4(17) H2
→Fe(CO)4 (17) H2
CO → Fe(CO) |
(11) |
5 |
|
(14) OHOH
19.0 |
( 21.9) |
24.9 |
11.3 |
( 14.2) |
17.2 |
3.7 |
( 6.6) |
9.6 |
9.8 |
|
|
106.5 |
( 101.8) |
97.8 |
70.5 |
( 65.8) |
61.8 |
71.1 |
( 66.4) |
62.4 |
60.8 |
|
3.4 |
3.4 |
( 4.4) |
5.0 |
3.4 |
( 4.4) |
5.0 |
|
— |
|
— |
|
|
5.2 |
(8.6) |
12.5 |
3.9 |
( 7.3) |
11.2 |
6.4 |
(3.0) |
0.9 |
4 |
|
7 |
33.6 |
(29.3) |
24.8 |
33.7 |
(29.4) |
24.9 |
33.8 |
(29.5) |
25.0 |
18.9 |
|
3 |
8.5 |
( 6.9) |
4.8 |
7.0 |
( 5.4) |
3.3 |
|
— |
|
— |
|
|
115.9 |
(111.8) |
106.9 |
82.6 |
(78.5) |
73.6 |
|
— |
|
— |
|
|
107.4 |
(104.9) |
102.1 |
75.6 |
(73.1) |
70.3 |
71.9 |
(69.4) |
66.6 |
71.5 |
|
|
8.4 |
(8.2) |
8.3 |
7.9 |
(7.7) |
7.8 |
12.0 |
(11.8) |
11.9 |
— |
|
|
17.1 |
(12.8) |
9.7 |
17.5 |
(13.2) |
10.1 |
24.4 |
(20.1) |
17.0 |
— |
|
|
25.5 |
(21.0) |
18.0 |
25.4 |
(20.9) |
17.9 |
36.4 |
(31.9) |
28.9 |
26 2 |
||
40.2 |
( 37.4) |
34.7 |
37.9 |
( 35.1) |
32.4 |
47.3 |
( 44.5) |
41.9 |
41.5 |
|
Numbering of the species as in Fig. 4. Numbers in parentheses include the ZPE correction computed at B3LYP/II. Numbers in italics include ZPE thermal corrections computed at B3LYP/II. Source: Ref. 72.
Compounds TM Calculating for Methods QM
101
102 |
Diedenhofen et al. |
SCHEME 1 Schematic representation of the one-step [3 2] addition and the two-step reaction via [2 2] addition of transition metal oxides to olefins.
uniformly 13–15 kcal/mol higher than the B3LYP/II data. Note that the formal oxidation state of the metal changes from 8 to 6 for Os and from 7 to 5 for Re when the dioxylate is formed. It is our general experience that the relative energies of TM compounds are particularly sensitive to the theoretical level of the calculation when the oxidation state or the coordination number of the molecule changes. This finding holds for DFT calculations and, to a less extent, also for CCSD(T) results. Table 15 shows that the CCSD(T) values for the relative energy of the osmium dioxylate using basis sets III and II differ only by 6 kcal/mol.
Reductive Elimination and Oxidative Addition
Reductive elimination and oxidative addition are ubiquitous reaction steps in many TM-catalyzed processes. A recent study by Beste and Frenking (82) may serve as example for the general finding that relative energies of TM complexes with different coordination numbers may be subject to systematic errors at the DFT level of theory. Table 16 shows calculated energies at the CCSD(T)/II level and at B3LYP using three different basis sets, II–IV, for platinum complexes

TABLE 15 Calculated (B3LYP/II, B3LYP/III //B3LYP/II, CCSD(T)/II//B3LYP/II, and CCSD(T)/III //B3LYP/II) |
|
||||
Relative Energies E |
rel |
(kcal/mol) for the Stationary Points of the [3 |
2] and [2 |
2] Additions of TM Oxides LTMO |
3 |
to Ethylene |
|
|
|
|
|
|
|
|
|
|
|
LTMO3
OsO4
OsO4
OsO4
OsO4
ReO4
ReO4
ClReO3
ClReO3
CpReO3
CpReO3
Cp*ReO3
Cp*ReO3
Method |
|
B3LYP/II |
a |
|
|
B3LYP/III //B3LYP/II |
|
CCSD(T)/II//B3LYP/II |
a |
|
|
CCSD(T)/III //B3LYP/II |
|
B3LYP/II |
|
B3LYO/III //B3LYP/II |
|
B3LYP/II |
|
B3LYP/III //B3LYP/II |
|
B3LYP/II |
|
B3LYP/III //B3LYP/II |
|
B3LYP//II |
|
B3LYP/III //B3LYP/II |
LTMO |
3 |
C H |
4 |
|
2 |
||
0.0 |
|
||
0.0 |
|
||
0.0 |
|
||
0.0 |
|
||
0.0 |
|
||
0.0 |
|
||
0.0 |
|
||
0.0 |
|
||
0.0 |
|
||
0.0 |
|
||
0.0 |
|
||
0.0 |
|
TS [3 2] |
Dioxylate |
5.032.3
11.819.1
9.621.2
11.015.2
36.015.5
44.830.8
21.81.4
30.614.7
13.621.0
20.7 |
8.7 |
16.8 |
|
23.0 |
|
TS [2 2] |
|||
44.0 |
|
|
|
47.9 |
|
|
|
44.7 |
|
|
|
46.2 |
|
|
|
50.6 |
|
|
|
30.0; 35.2 |
|
||
34.5; 39.1 |
|
||
25.4; 33.3 |
|
||
29.9; |
37.8 |
b |
|
|
|
||
b |
40.3 |
b |
|
41.5 ; |
|
Oxetane
5.0 12.7 11.1
19.1 27.2 5.9; 2.2 13.1; 9.22.2; 6.3 4.7; 0.9
TS [rear] |
|
|||
36.2 |
|
|||
45.4 |
|
|||
41.8 |
|
|||
95.4 |
|
|||
108.1 |
|
|||
|
c |
; 49.8 |
||
52.2 |
|
|||
61.8; 60.0 |
|
|||
49.3 |
c |
; 49.6 |
c |
|
|
|
|||
58.3; 60.0 |
|
a In cases where cis and trans isomers are found, the cis isomer is given first and the trans isomer is given second.
b From a geometry taken from the analogous Cp system, only internal Cp* coordinates and Re–C(Cp*) distances were optimized. c Instability of the restricted wave function. The unrestricted ansatz leads to an energy correction lower than 0.1 kcal/mol. Source: Ref. 81.
Compounds TM Calculating for Methods QM
103

104
TABLE
16 Relative Energies (kcal/mol) of Platinum Complexes with Different Coordination Numbers
Compound |
|
|
|
|
|
|
|
|
|
|
|
|
|
|
|
|||
cis-[Pt(CH )(SiH )(PH ) ] |
C |
H |
2 |
|
||||||||||||||
|
|
3 |
|
3 |
|
3 |
2 |
|
|
|
|
2 |
|
|
||||
[Pt(CH )(SiH )(PH ] |
C |
H |
2 |
PH |
3 |
|||||||||||||
3 |
|
|
|
3 |
3 |
|
|
|
|
2 |
|
|
|
|
|
|
||
[Pt(CH )(SiH )(PH ) (C H |
)] |
|
|
|
|
|
||||||||||||
3 |
|
|
|
3 |
3 |
2 |
|
2 |
|
2 |
|
|
|
|
|
|
|
|
[Pt(CH )(SiH )(PH )(C H )PH |
3 |
|
|
|
||||||||||||||
3 |
|
|
|
3 |
3 |
|
2 |
|
2 |
|
|
|
|
|
|
|
||
[Pt(PH )(C H )] |
CH |
SiH |
|
PH |
|
|||||||||||||
3 |
|
2 |
|
2 |
|
|
3 |
|
|
|
|
3 |
|
|
|
|
3 |
|
[Pt(PH ) (C |
2 |
H )] CH |
|
SiH |
3 |
|
|
|
|
|||||||||
3 |
2 |
|
2 |
|
|
|
3 |
|
|
|
|
|
|
|
|
|||
Source: |
Ref. 82. |
|
|
|
|
|
|
|
|
|
|
|
|
|
|
E
|
Coordination |
|
|
No. |
no. |
CCSD(T)/II |
B3LYP/II |
18 |
4 |
0.0 |
0.0 |
19 |
3 |
21.1 |
14.1 |
20 |
5 |
1.8 |
11.1 |
21 |
4 |
7.5 |
6.3 |
22 |
2 |
10.9 |
2.0 |
23 |
3 |
16.0 |
16.5 |
rel
B3LYP/III B3LYP/IV
0.00.0
14.413.5
10.614.0
6.3 |
8.3 |
1.8 |
3.1 |
16.9 |
14.2 |
.al et Diedenhofen
QM Methods for Calculating TM Compounds |
105 |
with the formal coordination numbers 2–5. The calculations are part of a theoretical study of the reductive elimination of CH3EH3 from cis-[Pt(CH3)(EH3)(PH3)2] (E Si, Ge) (82).
The energy of the four-coordinate complex 18 and free acetylene serves as a reference. Table 16 shows that the relative energy of the three-coordinate complex 19, which gives the Pt-PH3 BDE, is higher than that of 18 C2H2. The energy difference at CCSD(T) is 21.1 kcal/mol, but it is only 14.1 kcal/mol at B3LYP/II. The B3LYP value hardly changes when the basis set becomes larger. The five-coordinate complex 20 is predicted at CCSD(T)/II to be only 1.8 kcal/ mol higher in energy than 18 C2H2, while B3LYP gives an energy difference of over 10 kcal/mol. Thus, the three-coordinate complex 19 is calculated too low in energy and the five-coordinate complex 20 is predicted too unstable at B3LYP. The relative energies of the four-coordinate complexes 18 and 21 at CCSD(T)/ II and B3LYP/II-IV are very similar.
The same trend is found for the relative energies of 22 and 23 with respect to 18. Complexes 22 and 23 are formally two-coordinate and three-coordinate species, respectively. However, the acetylene ligand may serve as a four-electron donor in electron-deficient species (83). Therefore, 22 should be considered as three-coordinate and 23 as four-coordinate. The relative energies given in Table 16 show that CCSD(T)/II and B3LYP/II-IV give similar values for 23, while 22 is predicted too stable at the B3LYP level compared with CCSD(T)/II.
Epoxidation of Ethylene with Rheniumperoxide Complexes
Another TM-catalyzed reaction that has been studied at the DFT and ab initio levels of theory is the epoxidation of ethylene with rhenium peroxo complexes. Table 17 shows calculated reaction energies at the MP2, B3LYP, and CCSD(T) levels of theory using basis set II (84).
The energies of reactions 1–3 in Table 17 give the strength of the Lewis acidity of the Re(VII) complexes with respect to water. All methods agree that the water complexation energy of the diperoxo complex is clearly higher than the binding energies of the monoperoxo and trioxo complexes. Reactions 4 and 5 are peroxidation reactions. CCSD(T) and B3LYP agree that the second step of the peroxidation (reaction 5) is energetically less favorable than the first step (reaction 4), while MP2 gives the opposite result. Thus, MP2 may be qualitatively wrong in predicting reaction energies involving high-valent TM compounds. Reactions 6 and 7 are the analogous peroxidation reactions of the rhenium complexes with an additional water ligand. It is interesting that all three methods concur that the second peroxidation step (reaction 7) is thermodynamically more favored than the first reaction (reaction 6). B3LYP energies are not available for the energies of the epoxidation reactions 8 and 9, which are predicted by MP2 and CCSD(T) to be strongly exothermic.

106
TABLE 17 |
Reaction Energies (kcal/mol) for the Trioxorhenium-Catalyzed Oxidation of Olefins |
||
Reaction |
No. |
∆E |
∆E |
|
|
MP2 |
B3LYP |
|
1 |
11.1 ( 8.9) |
9.4 |
∆ECCSD(T)
11.8 ( 9.2)
2 |
12.7 |
( 10.1) |
8.5 |
11.8 ( 8.9) |
|
3 |
16.0 |
( 13.2) |
16.3 |
21.5 |
( 18.7) |
4 |
2.4 |
(1.7) |
1.6 |
2.4 |
( 3.1) |
5 |
3.4 |
( 4.4) |
5.2 |
2.0 |
(1.0) |
.al et Diedenhofen

ZPE (MP2) corrected values in parentheses. Source: Ref. 84.
6 |
0.8 |
(0.6) |
2.5 |
2.0 |
( 2.3) |
7 |
6.8 (7.5) |
2.6 |
8.0 |
( 6.6) |
|
8 |
54.5 |
( 52.9) |
— |
45.4 |
( 43.8) |
9 |
46.9 |
( 44.9) |
— |
39.4 |
( 37.4) |
Compounds TM Calculating for Methods QM
107