
Cundari Th.R. -- Computational Organometallic Chemistry-0824704789
.pdf
198 |
Parrill et al. |
studies. They each also provide a relative ranking based on empirical interaction energies, either steric and electrostatic in the case of the MOE docking method, or van der Waals interaction energy in the case of the fast Fourier transform method. These rankings both fail to consider the importance of directionality in hydrogen bonding interactions as well as entropic contributions to the binding free energy from desolvation and loss of conformational freedom. Thus the docked configurations generated by the MOE program were additionally evaluated using the SCORE program (43). The SCORE program is an empirical method for calculation of binding affinities and was calibrated using crystallographic complexes from the Protein DataBank with known binding affinities (43). It was therefore not specifically developed to determine the actual binding site for a ligand given several potential complexes, but rather to compare binding affinities among correct geometries of different protein:ligand complexes. Thus this use of this program is outside the range of activities for which it was validated. Nevertheless, the SCORE program includes terms for desolvation, loss of ligand flexibility, metal ion interactions, and for three different strengths of hydrogen bonds. These terms are complementary to those utilized by the other tools, and SCORE provides a valuable additional tool for the determination of whether there is a single binding site that multiple methods agree upon.
Several salicylhydrazines with a range of biological activity were selected for use in docking studies. The structures and biological activities of these inhibitors are shown in Table 1. For each inhibitor studied, both docking methods generated complexes having the inhibitor in both regions of interest. The fast Fourier transform method, which scanned the entire protein surface, did not significantly populate other regions of the protein structure. The MOE method was also used to scan the entire protein surface for the largest salicylhydrazine (structure not shown) and also failed to significantly populate other regions. This indi-
TABLE 3 Relative Rankings for IN Complexes with Salicylhydrazines
|
|
|
Most |
|
|
|
structurally |
|
|
SCORE |
similar |
Inhibitor and location |
MOE rank |
rank |
FFT rank |
|
|
|
|
SH30—near catalytic loop (a) |
1 |
15 |
1 |
SH30—near catalytic loop (b) |
9 |
1 |
1 |
SH1—near catalytic loop |
2 |
3 |
9 |
SH2—near catalytic loop |
5 |
35 |
1 |
SH30—near metal ion |
2 |
37 |
7 |
SH1—near metal ion |
1 |
46 |
2 |
SH2—near metal ion |
1 |
30 |
1 |
|
|
|
|
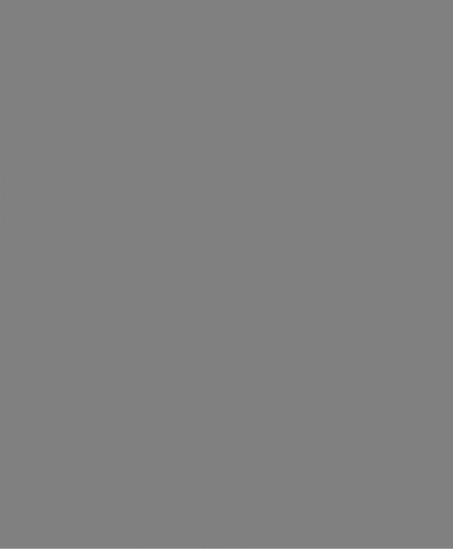
HIV IN Inhibitor Interactions with Active-Site Metal Ions |
199 |
FIGURE 8 Structures of docked salicylhydrazine inhibitors in the IN catalytic core. Salicylhydrazines positioned by the MOE program are shown as stick models, those positioned by the fast Fourier transform method are shown as ball and stick models. SH1, SH2, and SH30 are shown on the top left, top right, and bottom, respectively.

200 |
Parrill et al. |
cates that the choice of focus areas in the vicinity of the metal ion and above the catalytic loop was appropriate. The relative rankings of inhibitor interactions in these two sites of interest are shown in Table 3. The docked complexes are shown in Figure 8.
These results demonstrate that a better consensus among the evaluation methods is achieved for the two active inhibitors, SH30 and SH1, than for the inactive compound, SH2. The evaluation methods rate complexes having the inhibitor above the catalytic loop more consistently and as better ligands than those having the inhibitor near the metal ion. The ability of both docking methods to populate the site near the metal ion as well as above the catalytic loop does indicate that different inhibitors may show a preference for different sites based
FIGURE 9 Overlay of the HIV IN crystallographic complex with ClTEP (PDB entry 1QS4) on the highest-ranking docked complex of SH30 in the IN active site. Left: Ribbon model of the protein, with the metal ion shown as a spacefilling model, ClTEP shown as a ball-and-stick model, and SH30 shown as a stick model. Right: Close view of the metal ion (spacefilling), ClTEP (ball and stick), and SH30 (stick).
HIV IN Inhibitor Interactions with Active-Site Metal Ions |
201 |
on their structure. In fact, crystallographic evidence points to this possibility, for the crystal structure of ASV IN with 4-acetylamino-5-hydroxynaphthalene-2,7- disulfonic acid (Y3) (41) shows the inhibitor populating the site above the catalytic loop, whereas the crystal structure of HIV IN with 1-(5-chloroindol-3-yl)- 3-hydroxy-3-(2H-tetrazol-5-yl)-propenone (ClTEP) (16) shows the inhibitor populating the site near the metal ion.
Comparison of our docking results for the salicylhydrazines against the crystal structure of the HIV IN catalytic core with ClTEP shows some interesting correspondences. Figure 9 shows an overlay of this structure on our highestranking complex of SH30 in the metal ion site. The two inhibitors occupy overlapping regions of space and have weak π interactions with the metal ion. The
π ˚
distances observed for these interactions are 4.4 A for our SH30 complex and
˚
5.7 A in the ClTEP crystal structure. These are essentially the same, due to the resolution of the experimental structure. These π interactions are not optimal. Our optimizations of metal ion π complexes with both the thiazolothiazepines
˚
and the salicylhydrazines result in distances of less than 3 A. The apparent weakness of this interaction may demonstrate why our studies of inhibitor complexes with metal ions did not support a direct metal ion interaction. Studies are under way to dock the ClTEP inhibitor to the integrase catalytic core to evaluate whether the scoring methods used to rank the salicylhydrazine complexes are able to accurately provide a consensus that the metal ion site is more favorable for this inhibitor. The result of this evaluation will determine whether our apparent consensus favoring the site above the catalytic loop for the salicylhydrazines does indeed represent the preferred binding site for this class of inhibitors.
5. CONCLUSIONS
The multipronged approach toward elucidating the role of divalent metal cations in HIV IN inhibition has generated preliminary data that lend support to an allosteric involvement of the metal ion in inhibition by two chemical classes of HIV IN inhibitors, the salicylhydrazines and the thiazolothiazepines. First, both the metal-ion-sensitive salicylhydrazines and the metal-ion-insensitive thiazolothiazepines show different location preferences for chelation to Mg2 and Mn2 . Second, a series of 10 salicylhydrazines show a similar correlation between Mg2 or Mn2 binding affinity and biological activity determined in the presence of Mn2 . Third, different metal ions do confer minor structural differences on a model of the IN active site that may propagate into the surrounding region of the enzyme. Finally, docking results with the salicylhydrazines slightly favor salicylhydrazine binding above the catalytic loop rather than in the vicinity of the metal ion, although evaluation of our scoring methods on the recently crystallized HIV IN complex with ClTEP is still needed.
202 |
Parrill et al. |
ACKNOWLEDGMENTS
The generous support of the National Science Foundation (STI-9602656 for laboratory renovation and CHE-9708517 for computational resources) and the National Institute of Allergy and Infectious Diseases (1 R15 AI45984-01) are gratefully acknowledged. The support of the Chemical Computing Group in the form of the MOE program is also appreciated.
REFERENCES
1.MD Andrake, AM Skalka. J Biol Chem 271:19633–19636, 1996.
2.BG Turner, MF Summers. J Mol Biol 285:1–32, 1999.
3.JK Rockstroh, M Altfeld, B Kupfer, R Kaiser, G Fatkenheuer, B Salzberger, KE Schneweis, U Spengler. Eur J Med Res 4:271–274, 1999.
4.BA Larder, G Darby, DD Richman. Science 243:1731–1734, 1989.
5.WEJ Robinson. Infections Medicine 15:129–137, 1998.
6.JA Este, C Cabrera, D Schols, P Cherepanov, A Gutierrez, M Witvrouw, C Pannecouque, Z Debyser, RF Rando, B Clotet, J Desmyter, E De Clercq. Mol Pharmacol 53:340–345, 1998.
7.J Sakai, M Kawamura, J-I Sakuragi, S Sakuragi, R Shibata, A Ishimoto, N Ono, S Ueda, A Adachi. J Virol 67:1169–1174, 1993.
8.PM Cannon, ED Byles, SM Kingsman, A Kingsman. J Virol. 70:651–657, 1996.
9.F Dyda, AB Hickman, TM Jenkins, A Engelman, R Craigie, DR Davies. Science 266:1981–1986, 1994.
10.P Rice, R Craigie, DR Davies. Curr Opin Struct Biol 6:76–83, 1996.
11.G Bujacz, J Alexandratos, Q Zhou-Liu, C Cle´ment-Mella, A Wlodawer. FEBS Lett 398:175–178, 1996.
12.Y Goldgur, F Dyda, AB Hickman, TM Jenkins, R Craigie, DR Davies. Proc Natl Acad Sci USA 95:9150–9154, 1998.
13.M Cai, R Zheng, M Caffrey, M Craigie, R Craigie, GM Clore, AM Gronenborn. Nature Struct Biol 4:567–577, 1997.
14.M Cai, R Zheng, M Caffrey, R Craigie, GM Clore, AM Gronenborn. Nature Struct Biol 4:839–840, 1997.
15.PJ Lodi, JA Ernst, J Kuszewski, AM Hickman, A Engelman, R Craigie, GM Clore, AM Gronenborn. Biochemistry 34:9826–9833, 1995.
16.Y Goldgur, R Craigie, GH Cohen, T Fujiwara, T Fujishita, H Sugimoto, T Endo, H Murai, DR Davies. Proc Natl Acad Sci USA 96:13040–13043, 1999.
17.E Asante-Appiah, AM Skalka. J Biol Chem 272:16196–16205, 1997.
18.MR Fesen, KW Kohn, F Leteurtre, Y Pommier. Proc Natl Acad Sci USA 90:2399– 2403, 1993.
19.TRJ Burke, MR Fesen, A Mazumder, J Wang, AM Carothers, D Grunberger, J Driscoll, K Kohn, Y Pommier. J Med Chem 38:4171–4178, 1995.
20.MR Fesen, Y Pommier, F Leteurtre, S Hiroguchi, J Yung, KW Kohn. Biochem Pharm 48:595–608, 1994.
HIV IN Inhibitor Interactions with Active-Site Metal Ions |
203 |
21.A Mazumder, M Gupta, DM Perrin, DS Sigman, M Rabinovitz, Y Pommier. AIDS Res Hum Retroviruses 11:115–125, 1995.
22.H Zhao, N Neamati, A Mazumder, S Sunder, Y Pommier, TRJ Burke. J Med Chem 40:1186–1194, 1997.
23.A Mazumder, A Gazit, A Levitzki, M Nicklaus, J Yung, G Kohlhagen, Y Pommier. Biochemistry 34:15111–15122, 1995.
24.K Mekouar, J-F Mouscadet, D Desmae¨le, F Subra, H Leh, D Savoure´, C Auclair, J d’Angelo. J Med Chem 41:2846–2857, 1998.
25.MC Nicklaus, N Neamati, H Hong, A Mazumder, S Sunder, J Chen, GWA Milne, Y Pommier. J Med Chem 40:920–929, 1997.
26.DJ Hazuda, PJ Felock, JC Hastings, B Pramanik, AL Wolfe. J Virol 71:7005–7011, 1997.
27.N Neamati, H Hong, S Sunder, GWA Milne, Y Pommier. Mol Pharm 52:1041– 1055, 1997.
28.N Neamati, H Hong, JM Owen, S Sunder, HE Winslow, JL Christensen, H Zhao, TRJ Burke, GWA Milne, Y Pommier. J Med Chem 41:3202–3209, 1998.
29.N Neamati, JA Turpin, HE Winslow, JL Christensen, K Williamson, A Orr, WG Rice, Y Pommier, A Garofalo, A Brizzi, G Campiani. J Med Chem 42:3334–3341, 1999.
30.Spartan, Wavefunction, Inc, Irvine, 1993.
31.S Maignan, J-P Guilloteau, Q Zhou-Liu, C Cle´ment-Mella, V Mikol. J Mol Biol 282, 359–368, 1998.
32.MOE, Chemical Computing Group, Montreal, 1998.
33.WJ Stevens, H Basch, M Krauss. J Chem Phys 81:6026–6033, 1984.
34.TR Cundari, WJ Stevens. J Chem Phys 98:5555–5565, 1993.
35.WJ Stevens, H Basch, M Krauss, P Jasien. Can J Chem 70:612–630, 1992.
36.M Krauss, WJ Stevens. Ann Rev Phys Chem 35:357–385, 1985.
37.General Atomic and Molecular Electronic Structure System: GAMESS, Gordon Research Group, Iowa State University, Ames, IA, 1998.
38.G Bujacz, M Jaskolski, J Alexandratos, A Wlodawer, G Merkel, RA Katz, AM Skalka. Structures 4:89–96, 1996.
39.G Bujacz, J Alexandratos, A Wlodawer, G Merkel, M Andrake, RA Katz, AM Skalka. J Biol Chem 272:18161–18168, 1997.
40.TR Cundari, J Deng. J Chem Inf Comput Sci 39:376–381, 1999.
41.J Lubkowski, F Yang, J Alexandratos, A Wlodawer, H Zhao, TRJ Burke, N Neamati, Y Pommier, G Merkel, AM Skalka. Proc Natl Acad Sci USA 95:4831–4836, 1998.
42.AA Bliznyuk, JE Gready. J Comput Chem 20:938–988, 1999.
43.R Wang, L Liu, L Lai, Y Tang. J Mol Model 4:379–394, 1998.

8
Cyclometallation of a Computationally Designed Diene: Synthesis of ( )-Androst-4-ene-3,16-dione
Douglass F. Taber
University of Delaware, Newark, Delaware
James P. Louey
Sacred Heart University, Fairfield, Connecticut
Yanong Wang
American Home Products, Pearl River, New York
Wei Zhang
Bristol-Myers Squibb Pharmaceutical Research Institute, New Brunswick,
New Jersey
1. INTRODUCTION
We recently reported (1) a new approach to the stereoselective construction of polycyclic systems, illustrated by the cyclozirconation/carbonylation (2) of a computationally designed diene 1 (Scheme 1) to give the tetracyclic ketone 2. Ketone 2 was converted over several steps to ( )-androst-4-ene-3,16-dione (3).
(3) We describe here the history of this work and the development of the computational approach that led to the design of diene 1. We also lay out further lines of exploration that will be interesting to pursue.
2. INTRAMOLECULAR DIENE CYCLOZIRCONATION
When we began this work in 1988 (4), substantial work on the intramolecular cyclometallation of eneynes and diynes had been reported (5). It was, therefore, surprising
205
206 |
Taber et al. |
Scheme 1
Scheme 2
A Computationally Designed Diene |
207 |
that a general procedure for the intramolecular cyclometallation of dienes (Scheme 2) had not yet been developed (6). We were delighted to find that reduction of (Cp)2ZrCl2 with BuLi (7) in the presence of a diene 4 gave the zirconacycle 5 and that the metallacycle would react with a variety of interesting electrophiles.
3.THERMAL REVERSIBILITY OF DIENE CYCLOZIRCONATION
We discovered the thermal reversibility of the diene cyclometallation when we attempted cyclization of the diene 9 (Scheme 3) (8,9). The reaction proceeded to completion after two hours at room temperature, but rather than the expected tricyclic alcohol 13, the product was the dimer 11, from the reaction of two monosubstituted alkenes. We repeated the cyclozirconation, but let it proceed for a longer time. After 18 hours at room temperature, a new product had appeared (TLC analysis). After 1.5 hours at 75°C, the reaction was complete. The tricyclic alcohol 13 was isolated in 63% yield from 9.
Scheme 3