
Frantisek Svec - Capillary Electrochromatography
.pdf
184 |
A. Podgornik · T.B. Tennikova |
5
Chromatographic Reactors
5.1
Short Description
The idea of a chromatographic reactor was presented at the beginning of the 1960s almost simultaneously by several researchers [127–129]. They recognized the benefits of a simultaneous removal of products during a reaction, especially in the cases of reversible reactions where the conversion can be pushed beyond the thermodynamic equilibrium under these circumstances. In particular, a discontinuous chromatographic reactor was defined by Langer and Patton [130] as “a chromatographic column in which a solute or several solutes are intentionally converted,either partially or totally,to products during their resistance in the column. The solute reactant or reactant mixture is injected into chromatographic reactor as a pulse. Both conversion to products and separation take place in the course of passage through the column; the device is truly both a reactor and a chromatograph”.A schematic presentation of such a batch chromatographic reactor is shown in Fig. 3.
In such an apparatus, a chemical reaction takes place with a conversion of compound A into the products B and C. Typically, a sharp pulse of component A is fed into the column. During the passage through the column, compound A is converted into the products B and C and the amount of component A decreases. Because of their different retention times,the products B and C are concomitantly separated from each other and component A. Due to the removal of the products from the reaction zone, chemical equilibrium is never reached and the reaction will ideally proceed until the total conversion of the compound A. The reaction may take place in the stationary and/or the mobile phase. Heterogeneous reactions may be either catalyzed by the packed adsorbent or by an additional catalyst, which is mixed with the adsorbent.
The products leave the chromatographic reactor already separated and thus, no further purification steps are required. Therefore, two operations, namely reaction and separation, are combined in a single unit, which significantly reduces the costs of the whole process [131].
Fig. 3. Schematic presentation of the operating principle of a batch chromatographic reactor. A pulse of compound A is injected into the reactor. As the substance travels through the reactor it is converted into compounds B and C,which are continuously separated. (Reprinted with permission from [134])
Chromatographic Reactors Based on Biological Activity |
185 |
5.2
Reaction Types
The full advantage of the chromatographic reactor (simultaneous reaction and separation) is fully realized only for selected types of reactions, which are briefly summarized below [132].
5.2.1
Reversible Reaction A ´ B + C
In this case the application of a chromatographic reactor leads to significantly higher conversion when B and C are eluted on either side of A. It means that the capacity factors should be as follows: Kb >Ka >Kc or alternatively Kc >Ka >Kb . In the case of B and C eluting on the same side of A, even if they are separated, the improvement to be expected is much smaller [133].
5.2.2
Reversible Reaction: A + B ´ C + D
To obtain the best performance in this particular case, the products C and D should be separated in order to slow down the reverse reaction while good contact between the reactants A and B should be preserved. Three main possibilities can be distinguished:
–The retention times of A and B are different: a benefit of the separation can be obtained with a suitable feeding mode in a way that the pulse of the faster reactant travels through that of the slower reactant during their passage through the reactor.
–A and B have the same retention time: in this case the problem is similar to the case of a reaction of type A´B+C with the difference that the forward reaction is of second order and very sensitive to the injected concentrations.
–B is used as a carrier fluid: since B is in large excess, the problem is reduced to a reaction of type A´C+D.
5.2.3
Consecutive Competing Irreversible Reactions: A + B Æ R, R + B Æ S
Let R be the desired product. In this case,we have a competition between the rate of the second reaction and the rate of separation of R from B. Under these circumstances, the feeding mode determines the efficiency of the conversion. However, so far there are no published experimental results that would demonstrate the possibility of increasing yield of R by using a chromatographic reactor with simultaneous separation.

186 |
A. Podgornik · T.B. Tennikova |
5.2.4
Removal of Inhibitors
There are several reactions, especially in the case of enzymes, where either the substrate(s) or the product(s) inhibit partially or even totally the reaction. In such a case the removal of the inhibitor from the reaction zone results in a higher yield [133, 134].
5.3
Reactor Types
Increased conversion and product purity are not the only benefits of simultaneous separation during the reaction. The chromatographic reactor was also found to be a very suitable tool for studying kinetics and mechanisms of chemical and biochemical reactions. Some recent publications describe the results on investigation of autocatalytic reactions [135], first-order reversible reactions [136], and estimation of enantioselectivity [137, 138]. It is beyond the scope of this chapter to discuss the details, but the interested reader is referred to an overview published by Jeng and Langer [139].
Most publications dealing with chromatographic reactors focus on theoretical issues of this very complex system. Models of different complexity were derived and used to predict the behavior of chromatographic reactors. Such models typically take into consideration different types of mass transfer, adsorption isotherms,flow profiles,and reactions.A general scheme of these models,not including the reaction, is presented in Fig. 4. There are also several review papers
Fig. 4. Different types of models used for describing chromatographic processes. (Reprinted with permission from [131])
Chromatographic Reactors Based on Biological Activity |
187 |
which describe the results of mathematical simulations based on these models assuming different reaction rates. On the other hand, there is a smaller but significant number of publications with experimental data on the performance of chromatographic reactors. In most cases, gas-solid systems were studied although some experiments with liquid-solid systems were reported as well. An overview can be found in the book of Ganestos and Barker [140].
A chromatographic reactor can be realized with different configurations from a single fixed-bed reactor to multireactor arrangement enabling continuous operation. Here, a short description of the basic types together with some recent results are presented.
5.3.1
Batch Chromatographic Reactors
5.3.1.1
Fixed-Bed Chromatographic Reactor
Chromatographic fixed-bed reactors consists of a single chromatographic column containing a solid phase on which adsorption and reaction take place. Normally a pulse of reactant is injected into the reactor and, while traveling through the reactor, simultaneous conversion and separation take place (Fig. 3). Since an extensive overview of the models and applications of this type of reactor was presented by Sardin et al. [132],only a few recent results will be discussed here. Most of the practical applications have been based on gas-liquid systems,which are not applicable for the enzyme reactions,but a few reactions were also reported in the liquid phase. One of these studies, performed by Mazzotti and co-workers [141], analyzed the esterification of acetic acid into ethyl acetate according to the reaction:
ethanol + acetic acid ´ ethyl acetate + water.
The reaction is reversible and therefore the products should be removed from the reaction zone to improve conversion. The process was catalyzed by a commercially available poly(styrene-divinyl benzene) support,which played the dual role of catalyst and selective sorbent. The affinity of this resin was the highest for water, followed by ethanol, acetic acid, and finally ethyl acetate. The mathematical analysis was based on an equilibrium dispersive model where mass transfer resistances were neglected.Although many experiments were performed at different fed compositions, we will focus here on the one exhibiting the most complex behavior; see Fig. 5.
Prior to injection, the reactor was saturated with ethanol.At time 0, feeding of a mixture of ethanol and acetic acid in the ratio of 70:30 into the reactor was started. The concentrations of the different compounds were measured at the reactor outlet.
As the reactants entered the column, they were adsorbed and the reaction started.Water was strongly retained by the resin while the ethyl acetate was readily desorbed and carried by the mobile phase. Since the product was removed from the reaction zone,esterification proceeded until the full consumption of the
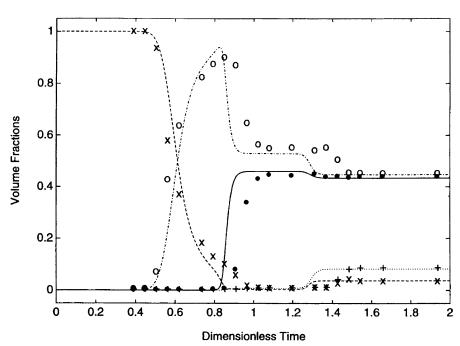
188 |
A. Podgornik · T.B. Tennikova |
Fig. 5. Complex behavior of a batch chromatographic reactor system.After an inlet step, three steady states were detected at the reactor outlet. Experimental data for acetic acid (filled circle), ethanol (¥), water (+) and ethyl acetate (open circle) were successfully fitted by a mathematical model (solid and dashed lines). (Reprinted with permission from [159])
limiting reactant. The process continued until the entire resin was saturated with water. The concentration profiles at the outlet of the reactor exhibited interesting dynamics.After approximately 0.5 dimensionless units of time, a sudden decrease of ethanol and a simultaneous increase of ethyl acetate was observed. This was the consequence of the weak retention of ethyl acetate. After approximately 0.8 units of time, a steady state characterized by a high ethyl acetate concentration was achieved. This increase was the result of the selective role of the resin. Water was adsorbed and the reaction was allowed to proceed to completion, i.e., far beyond the thermodynamic equilibrium. The second steady state was observed between 0.9 and 1.3 time units. This plateau was the result of the acetic acid excess in the feed.As the reaction was not complete, an equilibrium mixture enriched in ethyl acetate and acetic acid together with small amounts of water and ethanol was formed. A final steady state occurred after 1.2 units of time. At that point the resin became saturated with water, and thus no further retention of any component took place. As a result all concentrations corresponded to the thermodynamic equilibrium.A detailed analysis with a description of all the phenomena supported by the pertinent simulations was presented in the work. However, even this short summary should serve to indicate the very complex behavior of a chromatographic reactor, which depends on the composition of the feed, as well as on the adsorption characteristics of the matrix.
Chromatographic Reactors Based on Biological Activity |
189 |
A similar reaction, namely the hydrolysis of methyl acetate,
methyl acetate + water ´ acetic acid + methanol
was recently studied by Sircar and Rao [142]. In this case, two different supports were used, namely an ion exchanger as a catalysts and activated carbon as selective adsorbent for the acetic acid. The authors found that at a temperature of 35 °C a conversion of 31.4% could be achieved in the chromatographic reactor in comparison with 21.0%, which could be obtained with a fixed-bed reactor containing only the catalysts. Similar to the previous case, after saturation of the resin, a regeneration step was needed and a process scheme including this procedure to perform continuous process is presented in the paper.
As already discussed, the enhanced conversion is due to the separation of the products from the reaction zone. This can be realized via different distribution coefficients of the compounds (and consequently, a separation of the components) or via (selective) adsorption on a support. Since in the first case the compound travels through the reactor with different speeds,a continuous feed would cause repeated mixing of the separated compounds. Therefore, no improvement can be expected. In the second case,a regeneration of the adsorbent is needed after a certain operative period. This is an inherent drawback of the discontinuous operation of the fixed-bed chromatographic reactor.
For optimal performance, the feeding strategy for the chromatographic reactor should be carefully designed. In addition,the following criteria should be fulfilled [132]:
–The reaction rates should be as high as possible (under these circumstances reversible reactions are close to equilibrium).
–At least two chromatographically separable products must be formed.
–The reactants are introduced sequentially according to their retention properties.
–The adsorbent (and possibly, the mobile phase if a liquid) is chosen such as to obtain elution as specified by the stoichiometric ratios.
Recently,Falk and Seidel-Morgenstern [143] performed a detailed comparison between fixed-bed reactors and fixed-bed chromatographic reactors. The reaction studied was an equilibrium limited hydrolysis of methyl formate into formic acid and methanol using an ion-exchange resin as both the catalyst and the adsorbent. The analysis was based on a mathematical model, which was experimentally verified. The comparison was based on the following four assumptions:
–The same amount of feed was introduced into both reactors; i.e., pulses of higher concentration were injected into chromatographic reactor but due to the periods without feeding, the average concentration was equal to the concentration continuously feed into the fixed-bed reactor.
–The volumetric flows were the same.
–The properties of the solid phase were the same.
–The reactor dimensions and operating temperatures were the same.
The criterion used for this comparison was the achievable conversion. It was demonstrated that, taking into account the periodic nature of the batch chro-
190 |
A. Podgornik · T.B. Tennikova |
matographic reactor, a conventional fixed-bed reactor might lead to a higher overall conversion. Although this conclusion was drawn for a particular chemical reaction, the acknowledged drawback of a discontinuous operation may only be overcome by an alternative design of the chromatographic reactor.
5.3.2
Continuous Chromatographic Reactors
Three types of continuous chromatographic reactors can be generally distinguished: (1) the continuous annular chromatographic reactor, (2) the countercurrent moving bed chromatographic reactor, and (3) the simulated moving bed chromatographic reactor [144]. These types differ significantly in the design as well as in their performance. Each type will be described separately.
5.3.2.1
Continuous Annular Chromatographic Reactor
In the continuous annular chromatographic reactor the stationary phase is realized in the shape of an annulus, which is slowly rotating around its axis, while a continuous feed stream enters from a stationary inlet.A carrier liquid is distributed uniformly from above the annulus. The chemical reaction occurs in the bed and the reactant(s) and products are separated along the column’s axis by the carrier. Due to the rotation of the stationary phase, the components elute at different outlet angles according to the strength of their interaction with the stationary phase. The system is schematically presented in Fig. 6. To obtain optimum performance, the reaction rate should be fast enough for the reaction to occur primarily on top of the bed, while the bottom serves largely as a separator [144].
Reactions in which a single reactant gives more than one product are the most suitable for this type of chromatographic reactor. Therefore the reaction should be of type A´B+C and the adsorption of A, B, and C should differ significantly. As in the case of the discontinuous reactors discussed above, the most satisfactory operation is obtained if the distribution coefficient of A is between the values for B and C [144]. Few examples of real applications of this type of continuous chromatographic reactor exist in the literature. Cho et al. [145, 146] studied a liquid-phase hydrolysis of methyl formate. A gas-solid phase catalytic dehydrogenation of cyclohexane into benzene using a Pt catalyst was studied by the same group [147]. In addition, there are two reports on the application of this type of reactor to perform biochemical reaction using enzymes,both by Sarmidi and Barker [148, 149], which will be described in detail later on.
Recently,a theoretical analysis of the productivity of a heterogeneous catalytic reaction of the type
A+eluent ´ C+D
performed in a continuous annular chromatographic reactor was presented [150]. The annular chromatographic reactor was connected in two ways. In the first case the system consisted of a fixed-bed reactor followed by an annular chromatographic reactor and in the second of a fixed-bed reactor followed by a sim-

Chromatographic Reactors Based on Biological Activity |
191 |
Fig. 6. Schematic presentation of a continuous annular chromatographic reactor. The sample (big arrow) and the mobile phase (small arrows) are continuously introduced from the top of the column, which rotates with the constant angular velocity w. Passing through the column, compound A is converted into the compounds B and C. Due to their different retention they split in three streams and exit at different positions from the column. (Reprinted with permission from [144])
ple annular chromatographic separator. The efficiency of the particular systems depended on the differences in distribution coefficients of C and D, the reaction equilibrium constant, and whether the reaction is instantaneous or not. In addition, the productivity of the annular chromatographic reactor was shown to be highly dependent on the rotation rate. We can probably expect more experimental studies of this attractive type of chromatographic reactor in the future.
5.3.2.2
Countercurrent Moving Bed Chromatographic Reactor
Another approach to continuous reaction chromatography is the countercurrent moving-bed chromatographic reactor (CMCR). In this type of reactor the stationary (solid) phase travels in the opposite direction to the liquid phase. In practice this is performed by introducing the stationary phase from the top of the reactor. The stationary phase flows downwards under the influence of gravity while the liquid phase is pumped upwards from the bottom.A schematic presentation of such a system is shown in Fig. 7. Depending on the adsorption characteristics of the different components, they can travel in the direction of the liquid or the solid phase resulting in their separation.
The results of many, albeit mainly theoretical studies of the behavior of this type of reactor based on different reaction types and adsorption isotherms have
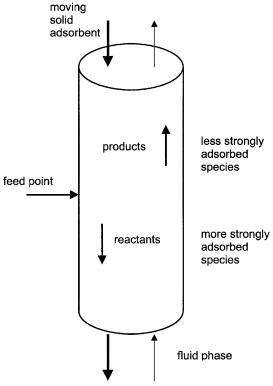
192 |
A. Podgornik · T.B. Tennikova |
Fig. 7. Schematic presentation of a true countercurrent moving bed chromatographic reactor. (Reprinted with permission from [151])
been published recently [144, 151]. However, there are very few examples of experimental results obtained with this type of chromatographic reactor. Takeuchi and Uraguchi [152] studied the oxidation of CO using aluminum oxide as a catalyst. Another experimental study was the catalytic hydrogenation of 1,3,5- trimethylbenzene into 1,3,5-trimethylcyclohexane [153]. In this case, a catalyst (Pt on aluminum particles) was continuously introduced into the reactor from the top. A higher purity of the product and a conversion much higher than the equilibrium one were obtained. The results were supported by theory [154]. According to the authors’ best knowledge no application of this type of reactors in the area of enzyme (or other biological) reactions has been published so far.
Although the countercurrent moving bed chromatographic reactor represents an interesting model for theoretical studies, the very few experimental examples already indicate the difficulties in realizing such a process in practice. One of the main difficulties is handling the solid phase. Its movement inevitable causes back mixing thereby reducing the efficiency of the process. Abrasion of the particles is another problem [131, 151]. To avoid the above-mentioned problems the simulated moving bed reactors discussed in the next section were developed.

Chromatographic Reactors Based on Biological Activity |
193 |
5.3.2.3
Simulated Moving Bed Reactors (SMBR)
In a simulated moving bed reactor, as its name already indicates, the movement of the solid phase is simulated. This is achieved by using a set of fixed-bed reactors (columns) connected in series and periodically switching the feed and withdrawal points from one column to the other. A schematic presentation of a simulated moving bed chromatographic reactor is shown in Fig. 8.
The process can be divided into four different sections. Section I is located between the desorbent and extraction node. The flow rate is higher than in all the other sections, which is necessary to remove the more strongly adsorbed product (here component B) from the adsorbent. Section II is located between the extract and the feed node. In this section the components B and C are formed. The less strongly adsorbed product (here component C) is desorbed and transported upstream together with the solvent, whereas B is still held on the adsorbent and transported to the extract port. The extract stream therefore contains the more strongly adsorbed product B. In Section III the conversion of component A takes place. Component B is retained and, thus, component C can be collected at the raffinate port. In Section IV component C is adsorbed and transported back to Section III together with the adsorbent, while the fluid phase is cleaned and recycled.
There are a number of papers published recently dealing with the modeling of the SMBR. Several researchers explored the effects of different parameters on
Fig. 8. Schematic presentation of a simulated moving bed chromatographic reactor together with profiles inside the columns. (Reprinted with permission from [131])