

24. Advances in the metathesis of olefins |
1587 |
If the two components of the copolymer are of similar polarity, there is no observable phase separation within the block copolymer and DSC shows a single glass transition temperature Tg. When there is a larger difference in polarity, microphase separation can be detected by a variety of techniques. The simplest test is to look for the two Tg peaks of the separate amorphous phases490. SAXS (small-angle X-ray scattering) can also be used, but most impressive is TEM, especially for metal-containing block copolymers; see Section VIII.C.12. The morphology of Si-containing block copolymers can also be observed by TEM. As the proportion of M2 units in the block copolymer decreases, the morphology changes in the usual way from lamellar to cylindrical to spherical and all three can be observed in the same system611.
The M2 units in the block copolymers from cyclobutene derivatives such as 136 and 139 readily eliminate an aromatic ring compound on heating, giving M20 units, DCHCHDCHCHD, equivalent to two acetylene units. M20 M1M20 triblocks made in this way can be doped with WF6 and their morphology studied by SEM. They can also be surface-etched by metathesis degradation so as to enhance the spherical domains of M1 units613. The reverse type of triblock, M1M20 M1, can also be made, in which the average number of double bonds in the centre block can be closely controlled. Comparison with M1M20 diblocks having the same number of M20 units shows that the triblocks are restricted in the extent to which their double bonds can be brought into conjugation by twisting of the polyene chain, whereas in diblocks the polyene chains are more mobile and more readily brought into conjugation610.
Amphiphilic star-block copolymers can be prepared by adding a polycyclic diene such as 238 to a living diblock copolymer made by sequential ROMP of (i) the monomer in Table 9 with R D COOSiMe3, and (ii) norbornene. The trimethylsilyl ester groups are then converted to carboxylic acids by soaking the cast film of the polymer in water for 2 3 days to give a product with a hydrophobic core of polynorbornene and a hydrophilic outer layer126,502.
C. Block Copolymers by Modification of Homopolymers
If the ROMP of norbornene is initiated with a titanacyclobutane complex, the resulting living polymer chains may be coupled by reaction with half an equivalent of a polymer containing keto end-groups, so as to give an ABA triblock copolymer. This has been done using poly(oxy-2,6-dimethyl-1,4-phenylene) to provide block B, the phenolic hydroxyl end-groups in this polymer being first reacted with 4-fluorobenzophenone in the presence of K2CO3 to give the required keto end-groups. A copolymer formed in this way with three equal blocks shows only a single Tg (70 °C) compared with 36 °C and 90 °C for A and B, respectively; thus there is no phase separation in this triblock material, even though A and B are not miscible. However, with exo-dicyclopentadiene in place of norbornene, the triblock does show microphase separation617. A variation on this principle has been used to make well-defined ABA triblock copolymers where A consists of units of 185 and B is a pre-determined number (9, 10, 11 or 12) of [DCHCHD] units396,618,619.
Block copolymers can also be made by transformation of the propagating species after polymerization of the first monomer. The following are some examples:
(1) If a mixture of anti- and syn-7-methylnorbornene (M1 and M2 respectively) is treated with W[DC(CH2)3CH2](Br)2(OCH2CMe3)2, M1 is selectively polymerized. If an equivalent of GaBr3 is now added, converting [W] Br into [W]C GaBr4 , the metal carbene becomes much more active allowing M2 to add to the living ends. However, propagation is much faster than initiation at this second stage and the product is a mixture of block copolymer and homopolymer of M1322.

1588 |
K. J. Ivin |
(2) When a mixture of norbornene (M1) and 5-acetoxy-1-cyclooctene (M2) is treated with 18 (Table 2) in CH2Cl2, M1 is selectively polymerized in less than 3 h. If 4 equiv of PCy3 are now added, the PPh3 ligands are displaced, giving a more active system and allowing M2 to add to the chains, resulting in an increase in MW. The reaction must be terminated quickly, otherwise secondary metathesis reactions occur, converting the initial block copolymer into a random copolymer597.
(3) A titanacyclobutane carrier for the living ROMP of norbornene can be converted, by reaction with methanol, into an alkyl titanocene methoxide complex which can then be used in conjunction with EtAlCl2 to propagate the ZNP of ethene, so forming a block copolymer of norbornene and ethene620.
(4) If the living ROMP of norbornene is terminated with a 9-fold excess of terephthalaldehyde, the chains formed carry an aldehyde end-group which, when activated by ZnCl2, can be used to initiate the aldol-group-transfer polymerization of tert- butyldimethylsilyl vinyl ether621.
(5) Living anionic polymerization of styrene can be initiated by butyllithium in cyclohexane. If a solution of WCl6 in cyclohexane is added to such a living polymer solution (MW D 61, 000, W/Li D 1/4) and then exposed to gaseous acetylene, the latter reacts to give a soluble product for which the GPC gives two peaks, one corresponding to the original polystyrene and the other, MW > 200, 000, consisting of a block copolymer of styrene and acetylene. It seems likely that the reaction of the living anionic polystyrene with WCl6 converts it partially into a living tungsten carbene complex which propagates the metathesis polymerization of acetylene622. Similar results have been obtained using cyclopentene in place of acetylene623.
(6) The ADMET polymerization of 1,4-divinylbenzene gives rise to linear oligomers containing up to 20 units from which the dimer, trimer and tetramer can be readily isolated and then cross-metathesized with polybutadiene using a tungsten carbene initiator. The UV/vis spectrum of the product shows that the oligomer sequence remains intact in the resulting copolymer624.
D. Comb and Graft Copolymers
1. Comb copolymers
These are homopolymers that have short regular side chains and are akin to graft
copolymers. They can be produced by the ROMP of such monomers as 284501, 285504
and 286470,471.
The polymers of 284 are hydrogels and can take up a moderate amount of water. The ROMP of 285 only proceeds to completion if the polystyrene side chains are kept reasonably short (n D 4, 7 or 9). Polymers of monomers such as 286 exhibit a nematic or smectic mesophase resulting from side-chain crystallization. Isotropization temperatures increase with increasing MW, becoming constant at about 30 50 repeat units. Side-chain crystallization tends to be suppressed as the MW increases.
2. Copolymers with short grafts
These can be made by copolymerizing two monomers, one of which contains a side chain such as C10 or C12, e.g. the copolymerization of norbornene dicarboxylic esters with 5-decylnorborn-2-ene. Alternatively, the homopolymer of the diester can first be hydrolysed to the acid form and then partially reacted with 1-dodecylamine. The viscosity

24. Advances in the metathesis of olefins |
1589 |
|
X |
X |
|
X |
|
|
|
X |
|
X = CO2 (CH2 )11CH3 |
X = CO2 CHMeCH2 [CHPhCH2 ]nCHEtMe |
|
(284) |
(285) n = 4−9 |
|
X
X = CO2 (CH2 )nO |
OMe |
(286) n = 2−8; exo/endo = 1/3
of aqueous solutions of such hydrophobically modified polymers increases sharply with increase in concentration as a result of intermolecular association582.
3. Copolymers with long grafts
Three examples may be cited. First, the macromonomer ω-norbornenylstyrene has been made by reacting a living anionic polystyrene with ethylene oxide to give a hydroxyl endgroup which is then reacted with norborn-5-ene-2-carbonyl chloride. The macromonomer so formed can be copolymerized with norbornene using WCl6/Me4Sn as catalyst. The resulting copolymer contains 3 16 polystyrene grafts per chain of 500 units and gives clear films, showing that the microdomains are smaller than the wavelength of visible light475.
Another macromonomer has been made by reacting the terminal hydroxyl groups of poly(oxy-2,6-dimethyl-1,4-phenylene) (MW D 2000 7000) with norborn-5-ene-2- carbonyl chloride. When this macromonomer is reacted with the 5-COO(CH2CH2O)3CH3 derivative of norbornene, using RuCl3 as catalyst, copolymers of MW up to 250,000 are obtained which contain about 2% of the macromonomer units. If the macromonomer contains only exo-substituted end-groups, it is more reactive than when it contains 55% exo and 45% endo end-groups625.
Thirdly, if living polynorbornene (A) having titanacyclobutane ends is terminated with a short-chain polyether ketone (B) containing about 3 keto groups per chain, a triblock copolymer ABA is formed, with a single graft of A emanating from the B block617.
E. Copolymers by ROMP in Conjunction with Radical Reactions
The ROMP of cyclooctene-5-methacrylate and its copolymerization with cyclooctadiene is catalysed by 19 in the presence of p-methoxyphenol as radical inhibitor. The double
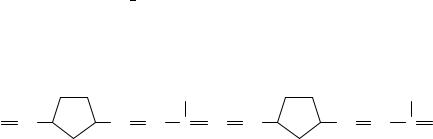
1590 |
K. J. Ivin |
bonds in the methacrylate groups are inert towards metathesis. After chain transfer with ethyl vinyl ether to release the polymer from the ruthenium centre, it can be cross-linked by radical polymerization through the methacrylate side chains626.
The simultaneous ROMP of norbornene or dicyclopentadiene on the one hand, and the radical polymerization of styrene or methyl methacrylate on the other, gives a polymer blend627.
X. POLYMERIZATION OF ACETYLENES BY OLEFIN METATHESIS CATALYSTS
A. Proof of Mechanism
The most direct proof of the metal carbene mechanism of polymerization of acetylenes by olefin metathesis catalysts (equation 3) comes from the use of electrondeficient (<18e) metal carbene complexes as initiators. Thus 7W (R D Me) initiates the polymerization of acetylene itself, and triblock copolymers can be made by successive addition of norbornene, acetylene and norbornene to the initiator. Provided that the centre block contains not more than 10 units of acetylene, such triblock copolymers have narrow MWD (Mw/Mn < 1.1), characteristic of a living system628. Again, Ta(DCHCMe3)(DIPP)3(THF), where DIPP D 2,6-diisopropylphenoxide, reacts with 1 equiv of MeC CMe to give a THF-free metallacyclobutene complex, which on addition of pyridine yields a vinylalkylidene complex by opening of the
metallacyclobutene |
ring. This |
can then |
be used to |
initiate |
the polymerization of |
|
up to 200 equiv of MeC CMe to give |
a living polymer which, after |
termination |
||||
629 |
||||||
with benzaldehyde, |
is found |
to have a |
very narrow |
MWD |
Mw/Mn |
< 1.05 . |
Likewise Mo(DCHCMe2Ph)(DN-adamantyl)[OCH(CF3)2]2(2,4-lutidine) initiates the living polymerization of HC CC6H4(SiMe3)-2 to give a polymer with MW proportional to the amount of monomer consumed and with Mw/Mn D 1.05630.
Further evidence comes from the structure of statistical copolymers of cycloalkenes (M1) with acetylenes (M2) in which the M1M2 junctions are present in sufficient proportion to be detectable by NMR. For example, a copolymer of cyclooctene and 2,4-dichlorophenylacetylene, containing 15% of M1 units, has been prepared using WCl4[OCH(CH2Cl)2]2/Et2AlCl as catalyst. The reactivity ratios are such that these units are nearly all flanked by M2 units, and the olefinic protons of the M1 units give a signal at υ 5.62, somewhat shifted from that for the olefinic protons in the homopolymer of M1 υ 5.3 631. This is direct proof that acetylenes can add to propagating metal carbene complexes. Another example is the copolymerization of norbornene (M1) with phenylacetylene (M2) using WCl6 as catalyst in toluene at 30 °C. The reactivity ratios, r1 D 0.20, r2 D 7.0, show that both types of propagating metal carbene complex P1 and P2 (in which the previous units added were M1 and M2 respectively) prefer to add M2 rather than M1. In the 1H 1H COSY NMR spectrum of the copolymer there are two correlations for the M1M2 olefinic protons, one at the intersection of υ 5.2 and 6.1, the other at the intersection of υ 5.5 and 6.6. These are assigned to the protons in the cis and trans M1M2 dyads 287 and 288. The presence of these peaks proves that the product is a statistical copolymer and not a mixture of homopolymers632,633.
|
|
|
Ph |
|
|
|
Ph |
[ CH • |
• |
|
c |
[ CH • |
• |
|
t |
CH |
CH C ] |
CH |
CH C ] |
||||
|
δ |
5.2 |
6.1 |
|
δ |
5.5 |
6.6 |
|
(287) |
|
|
(288) |
|

24. Advances in the metathesis of olefins |
1591 |
A further confirmation of the metal carbene mechanism is provided by enyne intramolecular metathesis reactions such as that depicted in equation 61. The C C bond in the substrate becomes the single bond attaching the alkenyl group to the phenanthrene ring system634,635.
C CH
CH CHMe
H
C
C H
W[ =C(OMe)Ph](CO)5
in toluene, 75 °C/18 h |
Me |
(61) |
|
(289) |
(290) |
B. Metathesis Polymerization of Acetylene
Since 1985 a number of typical olefin metathesis catalysts have been found to induce the polymerization of acetylene636 641. In most cases the polyacetylene formed is a black intractable material, insoluble in all solvents, and having a high trans content. However the initiator 7 (R D Me), in the presence of quinuclidine, provides much better control, allowing the preparation of soluble polymers containing up to 9 monomer units. The quinuclidine forms stronger complexes with the propagating species than with the initiating species, thereby slowing down propagation relative to initiation. The living ends may be terminated in a controlled way by cleavage with pivaldehyde. In the HPLC of such polymers, both the all-trans oligomers (n D 3 9) and those containing one or more cis double bonds are resolved396,628.
C. Metathesis Polymerization of Monosubstituted Acetylenes
Monosubstituted acetylenes are polymerized by most metathesis catalysts. The halides NbCl5, TaCl5, MoCl5, WCl6 can be used without a cocatalyst because acetylenes themselves react readily with the halide to generate an initiating metal carbene complex.
The |
product may be linear polymer |
only |
(MW D642103 |
|
106), cyclic oligomers only |
|
|
||||||
or a |
mixture of linear polymer and |
cyclic |
trimers |
. The linear polymers generally |
have an all-HT structure295. Backbiting to give cyclic trimers is markedly reduced or totally eliminated by the presence of bulky groups either on the metal centre or on the monomer. For example, HC CBu/TaCl5 (or NbCl5 643 and HC CCH(SiMe3)R/TaCl5644 give only cyclic trimers, whereas HC CCMe3/TaCl5645 and HC CCMe3/Nb(OC6H3- Me2-2,6)Cl4(THF)/t-BuMgCl646 give high polymer (MW ca 106).
The catalyst system MoOCl4/Bu4Sn/EtOH (1/1/1) is remarkable in that it is not only active in toluene at 30 °C, but it gives a 97% cis living polymer of CH CCMe3, though the catalyst efficiency is only about 2%647.
Much work has been carried out on phenylacetylene and its derivatives631,648 674. Of particular interest are the ortho-substituted phenyl derivatives because, unlike their meta and para isomers, they generally give living systems when initiated by MoOCl4/Bu4Sn/EtOH (1/1/1), allowing the preparation of block copolymers. This is the

1592 |
K. J. Ivin |
case for phenylacetylenes with the following ortho-substituents: Me, CHMe2, GeMe3, Cl, Br, CF3672,675 678, SiMe3678 and also for (2,3,5,6-tetrafluoro-4-butyl-phenyl)acetylene679. As with HC CCMe3647, the steric hindrance offered by the substituents close to the metal centre in the propagating species is evidently a decisive factor in giving a living system with this catalyst. Even more instructive is the behaviour of the o-SiMe3 monomer with Mo(DCHCMe2Ph)(DN-adamantyl)(OR)2(base) initiators, in which the first step is represented by equation 62630.
|
|
|
|
|
|
R′C |
|
CH |
|
R′ |
|
Mo( |
|
CHCMe2 Ph)( |
|
NAd(OR)2 |
(lut) |
|
(NAd)(OR)2 Mo |
α |
Hγ |
||
|
|
|
|||||||||
|
|
|
|
|
|||||||
|
|
|
|
|
|||||||
|
|
−lut |
|
|
|||||||
|
|
|
|
|
|
(R′ = o-Me3 SiC6 H4 ) |
Hβ |
|
CMe2 Ph |
||
(291) |
|
|
|
|
|
|
|||||
|
|
|
|
|
|
|
|
(292) |
(62) |
|
With initiator 291 [OR D OCH(CF3)2, lut D 2,4-lutidine, Ad D adamantyl], the first insertion product is 292, in which the phenyl substituent is attached to the ˛-carbon (head structure), the double bond is trans (Jˇ D 15.6 Hz) and the metal centre is base-free. The monomer is thus able to displace the lutidine ligand in 291 and then react, but the lutidine is unable to coordinate to 292 where less space is available. When further monomer is added the MW of the polymer formed is directly proportional to the amount of monomer consumed, and Mw/Mn D 1.04 when Mn D 13, 900. Initiators with bulkier alkoxy or imido ligands either do not react or fail to show the characteristics of a living system. The key to success with this monomer is therefore to use a metal carbene complex with relatively small ligands. For the shorter-chain polymers (DP < 25) the absorption spectrum initially shows two maxima separated by about 50 nm but on standing gives largely the red-shifted form; this change is attributed to cis ! trans isomerization. The living polymerizations of ethynylferrocene and ethynylruthenocene are also readily initiated680.
The polymers of the more highly substituted acetylenes RC CH can generally be prepared with higher MW than for those of the less highly substituted acetylenes. They are usually colourless or yellow, amorphous, readily soluble and non-conducting, in sharp contrast to polyacetylene itself, which is black, insoluble and semi-conducting, showing metallic conduction when doped. This is because the substituents force the main chains to take up twisted, non-conjugated, conformations.
D. Metathesis Polymerization of Disubstituted Acetylenes
W(CO)6 in hexane is effective as a photochemical initiator of polymerization of nonfunctionalized disubstituted acetylenes, such as MeC CMe, only if 1% of a monosubstituted acetylene is added. Polymerization then begins after an induction period of 30 min during which time a steady concentration of an active metal carbene complex is presumably generated. In contrast, initiation by Mt(CO)6/hv/CCl4 (Mt D Mo, W) is effective for the polymerization of PhC CCl without the use of a cocatalyst, and gives high polymer (Mw ca 106). Reaction continues in the dark after an initial irradiation period during which
time the initiating species [Mt]DCCl2 is thought to be generated681,682. SnCl4/toluene can be used in place of CCl4683.
Terminal olefins such as vinyltrimethylsilane can act as chain transfer agents in the polymerization of MeC CPh catalysed by NbCl5/Bu4Sn or of ClC CC6H13 or ClC CPh

24. Advances in the metathesis of olefins |
1593 |
catalysed by MoCl5/Bu4Sn. The end groups derived from the transfer agent can be detected when the MW is sufficiently low684,685.
With WCl6 or MoCl5 as catalyst it is generally necessary to use a cocatalyst such as Ph4Sn, unlike the situation with monosubstituted acetylenes, where the substrate acts as its own cocatalyst. With NbCl5 and TaCl5 a cocatalyst is not always necessary, though its use may affect the course of the reaction. For example, for the TaCl5-catalysed polymerization of MeC CPh in toluene at 80 °C, the conversion reaches 100% in 6 h, but the polymer then degrades rapidly to oligomer686,687. With TaCl5/Ph4Sn (1/1) under the same conditions the reaction is complete in 1 h and the MW of the polymer remains stable for at least 24 h; one may suppose that bulky ligands attached to the tantalum centre then prevent secondary metathesis reactions of the double bonds in the polymer chain. Similar
observations have been made with PhC CC6H4X-4 as monomer, where X D H688, CMe3 etc.689,690 and SiMe6913 ,692. The structure of the monomer can be a crucial factor with
these catalysts. Thus MeC CBu is polymerized by both MoCl5/Ph4Sn and WCl6/Ph4Sn
in toluene at 30 °C681, but the more sterically hindered MeC CCHMe2 is polymerized only by WCl6/Ph4Sn at 60 °C682.
The catalyst system MoOCl4/Bu4Sn/EtOH (2/2/1) is remarkable in that it gives living polymers of RC CCl (RDBu, Hex) having narrow MWDs (Mw/Mn D 1.1 1.4), just as it does with certain monosubstituted acetylenes (Section X.C). With MoOCl4 or MoOCl4/Bu4Sn as catalyst the MW of the polymer increases with conversion, but the polymers have a broader MWD indicating the occurrence of secondary metathesis reactions686,693. The role of EtOH in the three-component catalyst system is probably to replace one of the chloride ligands by an ethoxy ligand in the propagating metal carbene species thereby making it less prone to undergo termination or secondary metathesis reactions677. The 13C NMR spectra of these polymers are as expected for a regular HT structure694.
These polymers are generally white and have intrinsic viscosities which are sometimes nearly proportional to the MW, for example the polymer of EtC CPh695. This indicates that the polymer molecules have a fairly rigid but twisted backbone with very little conjugation of the double bonds, unlike polyacetylene and polymers of linear monosubstituted
acetylenes682.
Further details may be found in the reviews of Masuda and coworkers696,697 and of Breslow698.
E. Metathesis Polymerization of Diynes; Cyclopolymerization
The monomer HC C C CX (XDSiMe3) is readily polymerized by WCl6/Ph4Sn (1/1) in toluene at 40 °C to give a soluble polymer (Mn ca 104) which, when cast as a film and exposed to a mercury lamp for a few seconds, becomes completely insoluble699. The initial reaction is probably metathesis polymerization through the C CX bond, while the subsequent cross-linking by irradiation occurs through the other triple bond. Other 1,3-diynes (XDalkyl, phenyl, carbazolyl etc.) can be polymerized in this way. The polymerization of PhC C C CCH2OH by NbCl5/Bu4Sn in toluene at 80 °C gives an 80% yield of soluble polymer (Mw D 3200), which on heating under vacuum to 800 °C is converted to a graphite-like structure which has a high conductivity even in the absence of dopant. The first stage is thought to involve preferential metathesis polymerization through the C CCH2OH bond700.
The metathesis polymerization of diynes having four single bonds between the triple bonds (dipropargyl compounds) yields cyclopolymers. The structural units may contain a cyclohexene ring (equation 63) or a cyclopentene ring (equation 64) with the possibility

1594 |
K. J. Ivin |
of both in the same chain (see below).
|
|
[ |
] |
|
|
|
|
(63) |
|
CH |
CH |
|
X |
|
|
|
|
||
C |
C |
metathesis |
|
|
|
|
catalyst |
|
|
H2 C |
CH2 |
|
|
|
|
X |
[ |
] |
|
(see text for X |
||||
|
(64) |
|||
and catalyst) |
|
X
This reaction occurs with a wide variety of diynes in which X may be CPh2701, C(CO2R)2702 705, C<(CO.O)2 > CMe2706, C[CO2CH2(CF2)5CF2H]2707, C[CO2(CH2)6 N-carbazolyl]2708, CHOH709, CPh(OH)710a, C(OPh)2710b, CMe(OSiMe2 CMe3)707, CHY or CY2 where Y is a mesogenic group711,712, CHCO2(CH2)2O2CC6H3-(NO2)2-3,5713,
C[PO(OEt)2]2 and C(CO2Et)[PO(OEt)2]714, NY where Y is a mesogenic group715 718, NC R2Z where R D hexyl, Z D Br, BPh4, tos719,720, O721,722, S, SO or SO2723 725, SiR2 where R D Me, Ph726,727, GeR2 where R D Me, Ph728. When the monomer contains a mesogenic group, both the monomer and the cyclopolymer exhibit liquid-crystalline properties.
1H and 13C NMR spectra confirm the disappearance of triple bonds and the formation of double bonds during these reactions. Up to 1994 it was generally assumed that the rings formed were mainly 6-membered, but for X D C(CO2R)2 there is good evidence that 5-membered rings are also formed705. Thus, on addition of the initiator 8 (Table 2) to the monomer with R D Et, the carbene proton resonance of the initiator (υ 12.14) is replaced by resonances at υ 12.41 and 13.03, assigned to the carbene protons of living 293 and 294, respectively (equations 65 and 66). Furthermore, in the 13C NMR spectrum of the polymer, there are two distinct carbonyl signals (υ 170.8, 172.0) and two groups of quaternary carbon signals (υ 54 55, 57 58), the latter being assigned to 6- and 5- membered rings respectively by comparison with the spectra of model compounds. For the 5-membered rings the υ 57 58 signal shows fine structure that may be attributed to the influence of the ring structure in the adjacent units. On changing the initiator, the solvent, or the ester group in the monomer, there is some variation in the proportions of 5- and 6-membered rings formed (80/20 21/79), but the extremes are not reached.
[Mo] |
|
|
CHP |
||
|
|
||||
|
|
||||
|
|
+ |
|
|
β-addition |
|
|
|
|
|
|
HC |
|
CR′ |
|||
|
|
||||
|
|
||||
|
|
||||
|
|
P = polymer chain
R′ = CH2 XCH2 C CH X = C(CO2 Et)2
H |
[Mo] |
|
|
δ 12.41 |
C |
|
|
|
HC [Mo] |
|
|
|
|
|
|
C |
CHP |
||
C |
H |
C |
||
|
|
|
|
CHP |
|
|
|
|
|
|
X |
|
|
X |
|
|
(293) |
(65)

|
|
|
|
24. Advances in the metathesis of olefins |
1595 |
||||
|
|
|
|
|
H |
|
δ 13.03 |
[Mo] |
|
[Mo] |
|
|
CHP |
|
C |
[Mo] |
|
HC |
|
|
|
α-addition |
|
|
|
CH CHP |
|||
+ |
|
|
|
|
|||||
|
|
C |
C CH CHP |
|
|
|
|||
|
|
|
|
|
|||||
|
|
|
|
|
|||||
R′C |
|
CH |
|
|
X |
|
|
X |
|
|
|
|
|
|
|||||
|
|
|
|
|
|||||
|
|
|
|
|
|||||
|
|
|
|
|
|
|
|
||
|
|
|
|
|
|
|
|
|
(294) |
(66) |
|
Such reactions clearly involve two successive types of propagation step: (i) double-bond exchange between MtDC and the first C C of the monomer, and (ii) rapid intramolecular cyclization by double-bond exchange between MtDC and the second C C of the monomer. If the first step occurs by ˇ-addition (with the substituent ˇ to the metal centre), the subsequent intramolecular reaction is likely to yield a 6-membered ring (equation 65), whereas if it occurs by ˛-addition the subsequent reaction is more likely to yield a 5- membered ring (equation 66). This observation is somewhat surprising because simple monosubstituted acetylenes, containing one C C bond, appear to polymerize only by ˛-addition to give an all-HT structure (Section X.C). It is therefore unexpected that a monosubstituted acetylene in a diyne can add in both directions. Further work is needed to establish whether the result with X D C(CO2R)2 is the exception rather than the rule. Preliminary reports indicate that when X possesses one extremely bulky substituent (X D CHCH2OSiPh2But), the resulting polymer has mostly one type of ring structure713. Sterically demanding substituents (preferably two) on X certainly encourage the second, intramolecular, step and reduce the proportion of residual pendant C C groups in the polymer which, if they subsequently react with each other, can give rise to a component with double the MW of the main product.
The use of molybdenum carbene initiators, and suitably substituted benzaldehydes as terminating agents, allows the preparation of cyclopolymers having electron-donating or electron-attracting groups at one or both ends of the chains. When the chains are sufficiently short, say DP D 5, and the two end-groups are the same, the absorption maximum for the ‘push push’ polymer is at a rather longer wavelength than in the corresponding ‘pull pull’ polymer, but when the DP is 20 or more the chain length exceeds the effective conjugation length and the end groups have comparatively little effect705.
Copolymerization of the diynes having X D C(CO2Et)2 and X D C[CO2(CH2)6 N-carbazolyl]2 gives a conductive copolymer729. The cyclopolymerization of RC CCH2 OCH2C CH (R D Me, SiMe3) gives insoluble polymers; but their copolymers with diethyl dipropargylmalonate are soluble730. Tripropargylammonium bromide gives a conjugated polymeric salt having two cyclic recurring units per monomer unit731. Tetrapropargylammonium bromide gives a cross-linked cyclopolymer732.
Of the first-generation catalysts, those based on MoCl5 are the most effective, either with a cocatalyst such as EtAlCl2 or Bu4Sn, or alone, at 60 90 ° C in the usual solvents. Molybdenum carbene complexes give living systems, allowing the preparation of block copolymers of 1,4-diynes with norbornadiene derivatives705. The polymers are generally soluble and highly coloured, with MW D 103 105, and become conducting when doped with iodine. The diynes HC C(CH2)nC CH (n D 2, 4, 6, 8) have also been polymerized. For n D 2, using NbCl5 as catalyst, the product is mainly trimer, but for n D 6 or 8, a polymer with a highly branched structure appears to be formed733.
F. Copolymerization of Acetylenes
Acetylenes copolymerize with each other and with cycloalkenes under the influence of olefin metathesis catalysts. With non-living systems it is possible to make statistical

1596 |
K. J. Ivin |
copolymers by adding the monomer mixture to the catalyst system. Copolymers of acetylene with phenylacetylene can be made with MoCl5 as catalyst, containing 0 81.7 mol% acetylene units. The UV/vis absorption band moves to longer wavelengths as the proportion of acetylene in the copolymer increases, the absorption maximum at 508 nm corresponding to an average of 9 10 conjugated double bonds734.
The Hammett parameters for these copolymerizations indicate that the chain carrier is electrophilic, i.e. the carbene carbon bears a net positive charge, with the counterbalancing negative charge spread to some extent over the chloride ligands. The product of the two reactivity ratios is sometimes considerably larger than 1.0, e.g. 13 in the case of HC CC6H4CF3-2/norbornene, and 30 for HC CC6H3-(CF3)2-2,5/norbornene735,736. The copolymers in these cases are thus very blocky, which appears to be due to the presence of the 2-CF3 group. Occasionally, as in the copolymerization of phenylacetylene and cyclopentene, the product of the reactivity ratios is so great that the crosspropagation reactions scarcely occur and the product is essentially a mixture of the two homopolymers632.
When the propagating species are long-lived it is possible to make block copolymers of controlled chain length by sequential addition of monomers, for example with ClC CC4H9/ClC CC14H29686,693 and with acetylene/norbornene396,628.
XI. INTRAMOLECULAR METATHESIS REACTIONS OF ENYNES AND
DIENYNES
Enyne intramolecular metathesis reactions, of the type shown in equation 61, can be very useful in organic synthesis. A number of such reactions, catalysed by tungsten or chromium carbene complexes, have been reported634,635,737 740. The ruthenium carbene catalysts 18 20 (Table 2) are likely to be increasingly used for this purpose because of their stability, ease of handling and good yields, as in the synthesis of various 5-, 6- and
7-membered heterocycles, e.g. equation 67741.
|
|
19 (1 mol%) |
TsN |
|
|
|
|
TsN |
OAc |
benzene, reflux 40 min; |
|
yield 86% |
|
||
|
|
Me
O
19 (3 mol%)
benzene, 65˚C, 6 h yield 88%
Me
(67)
OAc
Me
O
Me |
Me |
(68) |
|
|
−MeCH CH2
Me
O
Me Me