

|
|
24. Advances in the metathesis of olefins |
1527 |
||
|
O |
|
O |
|
O |
|
|
|
|
||
|
|
Me |
|
N |
|
|
|
|
|
N |
|
|
N |
|
N |
Me |
|
|
|
Ph |
Ph |
Ph |
Ph |
|
[3] |
|
[2,3] |
[2] |
[3] |
|
|
|
O |
|
|
Me |
N |
R |
N |
|
|
|
|
Ph |
Ph |
|
|
|
[2] |
|
R = H, Me [2,3] |
|
|
Refs. [1]2 6 1, [2]2 2 7f, [3]2 2 7b, [4]2 2 7g, [5]78
The bis(N-allyl) dipeptide 52 gives a 51% yield of the cyclic dipeptide 53 under the conditions shown in equation 36, whereas an analogous N,O-bis(allyl) ester fails to undergo RCM238. This illustrates that the success or failure of RCM of such acyclic dienes depends critically upon the preferred conformations about the seven intervening bonds and upon the potential strain energy in the 8-membered ring to be formed. A number of factors may favour a rotamer which is conformationally and energetically disposed towards RCM, leading to the formation of 8-membered or larger rings. Such factors are (i) peptide linkages, which have a significant rotational barrier, as in 52; (ii) an attached ring which prevents rotation about one of the bonds, as in 54, equation 37; (iii) hydrogen bonds, particularly in polypeptides; see below. First it may be noted that 55 can be converted to 56, which is the core fragment of the anti-cancer agent FR-900482238,239.
|
Boc |
|
Boc |
|
N |
Me |
N |
Me |
|
|
|
|||
|
|
0.005 M, 10 mol% 19 |
|
(36) |
|
|
60 °C, 24 h, yield 51% |
|
|
N |
O |
N |
O |
|
|
|
|
||
|
CO2 Me |
|
CO2 Me |
|
(52) |
|
|
(53) |
|
The preparation of fused nitrogen heterocycles such as pyrrolizidines, indolizidines, quinolizidines, pyrrolidinoazocines and piperidinoazocines by the RCM of appropriate dienes (equation 38), is another case where presence of a ring assists the RCM reaction. However, when n D 7 (with x D 1), the CDC bonds, separated by 11 single bonds, are too far apart for RCM to occur. Applications of this general strategy are in prospect for the formation of fused nitrogen heterocyclic systems in problems of alkaloid synthesis240.

1528 |
|
|
|
K. J. Ivin |
|
|
|
|
|
|
|
OSiMe2 But |
|
|
|
|
|
|
OSiMe2 But |
|
|
|
0.0025 M, 10 mol% 19 |
|
|
|
|
|
|
|
|
|
60 °C, 24 h, yield 59% |
|
|
|
|
|
|
|
N |
|
|
|
|
|
|
N |
(37) |
|
Boc |
|
|
|
|
|
|
Boc |
|
|
|
|
OH |
|
|
|
|
||
|
(54) |
|
|
|
|
|
(55) |
|
|
|
|
|
|
|
|
|
|
||
|
|
|
|
O |
NH |
|
|
|
|
|
|
|
N |
|
|
|
|
|
|
O |
|
|
(56) |
|
|
|
O |
|
|
|
|
|
|
|
|
|
|
||
( )x |
|
|
0.015 M, 10 mol% 8 |
( |
) |
|
|
|
|
|
|
|
|
|
|
|
|
||
N |
( |
)n |
C6 H6 , 20-50 °C |
|
x |
N |
|
(38) |
|
|
|
|
|||||||
|
x = 1,2 |
yield = 65-90% |
|
|
|
( ) |
|
||
|
|
|
|
|
|
|
|||
|
n = 1,2,3 |
|
|
|
|
|
n |
|
|
|
|
|
|
|
|
|
|
||
|
|
O |
Me |
|
|
|
|
|
|
|
|
|
|
Me |
|
|
|
|
|
|
|
N |
|
O |
|
|
|
|
|
|
N |
H |
|
|
|
|
|
|
|
H |
|
H |
N |
O |
|
|
|
|
|
|
a |
|
|
|
|
|
|
||
N |
O |
b |
|
|
|
|
|
|
|
|
|
|
|
|
|
|
|
|
|
Boc |
|
|
|
|
Ph |
|
|
|
|
|
|
|
N |
|
|
|
|
|
|
|
|
|
|
|
|
|
|
|
|
|
|
|
|
H |
|
|
|
|
|
|
(57) (mixture of 4 diastereomers) |
|
|
O |
Me |
(39) |
|||
|
|
|
|
||||||
|
|
0.002 M, |
|
|
|
|
|
Me |
|
|
|
|
|
|
N |
|
|
||
|
20 mol% 19 |
|
|
|
|
|
O |
||
|
|
|
N |
|
H |
|
|||
|
CH2 Cl2 , 40 °C |
|
H |
|
|
|
|||
|
|
|
|
|
|
H |
N |
O |
|
|
|
|
|
|
|
|
|
O
N
Ph
Boc
N
H
(S, S, S)-(58) formed from one diastereomer
A remarkable case of RCM assisted by conformational restraint is that shown in equation 39. This involves a substrate in which the two double bonds are separated by 13 single bonds only one of which forms part of a ring, but in which an additional constraint is imposed by the hydrogen bond. When a mixture of the four stereoisomers denoted by 57 is treated with catalyst 19 under the conditions indicated, only one of the isomers undergoes RCM to give (S,S,S)-58, showing that in this case the configuration

24. Advances in the metathesis of olefins |
1529 |
with respect to the chiral centres a and b is also crucial. The yield is 60% with respect to the (S,S,S)-reactant241.
An even more remarkable case of RCM in a hydrogen-bonded system is shown in equation 40. The eight-residue cyclic polypeptide cyclo[-L-Phe-D-MeNAla-L-Hag-D- MeNAla)2-], containing two L-homoallylglycine (Hag) residues, self-assembles to form two interconverting hydrogen-bonded dimers, one of which is represented by 59 and contains two pairs of double bonds in sufficiently close proximity that each pair undergoes RCM in the presence of 19 to give 60 with a conversion of 65% (mixture of cc, ct and tt isomers). Such a strategy may be useful in stabilizing kinetically labile ˛-helical and ˇ-sheet peptide secondary structures242.
(59)
0.005 M, |
CHCl3 , |
(40) |
20 mol% 19 |
20 °C, 48 h |
|
|
|
|
(60)
Another case of long-range RCM is shown in equation 41. In 61 the CDC bonds are separated by 12 single bonds, four of which are restrained by ring systems. The product, 62, is an intermediate in the synthesis of manzamine A (a novel anti-tumour alkaloid)243; also see Martin244.
|
CH2 OSiPh2 But |
|
CH2 OSiPh2 But |
|
H |
|
|
|
|
|
H |
N |
O |
19 |
(41) |
|
N |
||
|
|
O |
|
O |
N |
|
|
|
|
||
|
O |
O |
N |
|
|
|
O |
(61) |
(62) |

1530 |
K. J. Ivin |
The synthesis of compound 64 from 63 by reaction 42 is notable in that there are 13 single bonds between the reacting double bonds, and only a single peptide link to provide a measure of conformational restraint. A 60% yield of a single stereoisomer (>98% Z) is achieved when carried out in 0.01 M solution in THF, using 25 mol% 8 as catalyst. After hydrogenation and removal of the silyl protecting group one obtains a direct relative of the anti-fungal agent Sch 38516 (fluvirucin B1)245.
|
|
|
|
Me |
|
|
Me |
|
|
|
|
|
OSiMe2 But |
8 |
|
|
OSiMe2 But |
|
|
|
|
|
|
|
O |
|
|
O |
|
Me HN |
Me |
Me |
HN |
|
Me |
|
(63) |
|
|
(64) |
(42) |
|
|
|
|
|
Finally, one should again note that with catalyst 19 one can carry out RCM on compounds containing unprotected peptidic structures, as illustrated by equation 43241.
Boc |
|
|
|
|
Boc |
|
|
N |
|
|
|
|
N |
|
|
H |
|
|
|
|
H |
|
|
|
|
|
0.2 M, 5 mol% 19 |
|
|
|
|
|
|
|
|
|
|
|
|
O |
N |
|
CHCl3 , 25 °C, |
O |
N |
|
|
|
|
|
yield 81% |
|
|
|
|
O |
|
|
|
|
O |
|
|
O |
|
|
|
|
O |
|
|
N |
|
|
|
|
|
N |
|
(PhCH2 )2 N |
H |
|
(PhCH2 )2 N |
|
H |
(43) |
|
|
|
|
|
|
|
|
|
5. Rings containing P |
|
|
|
|
|
|
|
Diallylphenyl phosphane (65) |
undergoes RCM when heated at 80 °C with 5 mol% |
of 12 in chlorobenzene for 24 h, giving a 95% yield of the corresponding phospholene derivative (66); see equation 44. The reaction is considerably faster than the intermolecular metathesis of allyldiphenyl phosphane with the same catalyst181,246.
PPh |
12 |
|
− C2 H4 |
||
|
PPh |
(44) |
|
(65) |
(66) |

24. Advances in the metathesis of olefins |
1531 |
6. Rings containing Si, Ge and Sn
The metathesis of dimethyldiallylsilane (67) is a case where there is a delicate balance between the intramolecular and intermolecular processes. The balance may be swung either way by adjusting the substrate concentration. The yield of cyclic ‘monomer’ (68), equation 45, varies from 28% to 90% according to the catalyst and conditions209,247,248. When the reaction is carried out in solution with 8 as catalyst, the three cyclic dimers of 68 (cc, ct and tt) are also formed (78% cc), at an overall concentration which
exceeds that of 68 itself247. Reactions analogous to equation 45 have been reported for (CH2DCHCH2)2GeMe2249 and (CH2DCHCH2)2SnBu2248, but with the formation of other products.
SiMe2 |
8 |
SiMe2 |
|
|
− C2 H4 |
(45) |
|||
|
|
|||
(67) |
|
(68) |
|
Good yields of product are obtained for reactions 46 (100%)234,250 and 47 (71%)251.
SiMe2 |
|
|
|
SiMe2 |
|
|
|
8 |
|
O |
(46) |
||
O |
−C2 H4 |
|||||
|
||||||
SiMe2 |
|
|
|
SiMe2 |
|
|
O |
|
|
|
O |
Me |
|
Me |
8 |
|
|
|||
Si |
|
−C2 H4 |
Si |
(47) |
||
Ph |
|
|
|
O |
Ph |
|
O |
|
|
|
|
B. ADMET Polymerization
The ability of hexa-1,5-diene to undergo multiple condensation reactions in the presence of a WCl6-based catalyst, with the elimination of ethene and the formation of a series of linear oligomers, was first reported by the group of Dall’Asta252 in 1973. The preparation of high polymers by this route was pioneered by Wagener and coworkers254 using first 8W and more recently 8 itself. These carbene catalysts have the advantage over the earlier catalysts of being Lewis-acid-free and of giving much longer-lived propagating systems. The molecular weight of the polymer increases as the reaction proceeds, its final value being determined by the initial ratio of substrate to initiator and by the initiation efficiency. Table 5 lists most of the dienes which have been shown to undergo ADMET polymerization over the last 6 years.
For the diallyl compounds the final product is an equilibrium mixture of the cyclic species formed by RCM and the linear polymer, as illustrated in Figure 2 for diallyl ether as reactant. In this case the double bonds in the polymer are nearly all trans. More usually the double bonds formed in ADMET polymers are 65 85% trans; an example is shown in Figure 3.
Isopropenyl groups are relatively unreactive towards metathesis. 2,5-dimethylhexa-1,5- diene, with an isopropenyl group at both ends, does not react at all in the presence of either 8 or 8W. However, while 2-methylhexa-1,5-diene reacts only at the vinyl end in the

1532 |
|
|
|
|
|
|
|
|
|
K. J. Ivin |
|
|
|
|
|
|
|
|
|||
TABLE 5. Substrates susceptible to ADMET polymerization |
|
|
|
|
|
|
|
||||||||||||||
|
|
|
|
|
|
|
|
|
|
|
|
|
|
|
|
|
|
|
|
||
|
|
|
|
Substrate |
Catalyst |
Polymer DP |
Reference |
||||||||||||||
|
|
|
|
|
|
|
|
|
|
|
|
|
|
|
|
|
|
|
|
|
|
(CH2DCHCH2)2 |
a |
>500 |
|
254 |
|||||||||||||||||
8W b |
|
||||||||||||||||||||
|
|
|
|
|
|
|
|
|
|
|
|
|
W-1 |
|
|
|
|
c |
|
255 |
|
CH2DC(Me)(CH2)2CHDCH2 |
8 |
|
|
|
|
|
162 |
||||||||||||||
d |
|
|
|
|
|
|
|||||||||||||||
p-CH2DCHC6H4CHDCH2 |
W-2 |
|
2 |
|
720 |
|
256 |
||||||||||||||
|
|
|
|||||||||||||||||||
[CH2DCH(CH2)3]2 |
8W |
|
ca 1000 |
254 |
|||||||||||||||||
|
|
|
|
|
|
|
|
|
|
|
|
|
8 |
|
130 |
|
257 |
||||
CH2 |
|
CH(CH ) C(Me) |
|
|
|
|
|
|
|
|
|
||||||||||
|
|
|
|
|
|
|
|
|
|
||||||||||||
|
|
|
|
|
|
|
|
|
|
||||||||||||
|
2 2 |
|
|
|
|
|
|
|
|
8 |
|
ca 110 |
|
258 |
|||||||
CH2 |
|
|
CH(CH2 )2 CH |
8 |
|
|
|
|
|
|
|
|
|||||||||
|
|
|
|
|
|
|
|
|
|
||||||||||||
|
|
|
|
|
|
|
|
|
|
||||||||||||
[CH2DCH(CH2)3 CMe2]2CO |
|
|
|
|
|
|
|
234 |
|||||||||||||
(CH2DCHCH2)2 SiMe2 (neat) |
8W, 8 |
100 |
|
247 |
|||||||||||||||||
(CH2DCHCH2)2SiPh2 (neat) |
8 |
|
20 |
e |
247 |
||||||||||||||||
(CH2DCHCH2)2SiMeCl (neat) |
8 |
|
oligomer |
|
259 |
||||||||||||||||
(CH2DCHCH2SiMe2CH2)2 |
8W |
|
240 |
|
260 |
||||||||||||||||
p-C6H4(SiMe2CH2CHDCH2)2 |
8W |
|
6 |
|
260 |
||||||||||||||||
[CH |
2DCH(CH2)2]2NPh |
8 |
|
45 |
|
261 |
|||||||||||||||
|
8 |
|
|
|
|
f |
|
262 |
|||||||||||||
(CH2DCHCH2)2O (neat) |
|
10 |
|
|
|||||||||||||||||
[CH2DCH(CH2)3]2O |
8 |
|
140 |
|
263 |
||||||||||||||||
[CH2DCH(CH2)4]2O |
8 |
|
100 |
|
263 |
||||||||||||||||
CH2DCH(CH2)2COO(CH2)4CHDCH2 |
8 |
|
101 |
|
264 |
||||||||||||||||
p-C6H4[COO(CH2)x CHDCH2]2 (x D 2 |
|
4) |
8 |
|
22 |
|
45 |
|
264 |
||||||||||||
|
|
|
|
||||||||||||||||||
[CH2DCH(CH2)8COOCH2]2 |
8W |
|
15 |
|
265 |
||||||||||||||||
[CH2DCH(CH2)x O]2CO (x D 2 |
|
4) |
8 |
|
51 |
|
58 |
|
266 |
||||||||||||
|
|
|
|
||||||||||||||||||
p-[CH2DCH(CH2)2OCOOC6H4]2CMe2 |
8 |
|
40 |
|
266 |
||||||||||||||||
[CH2DCH(CH2)3SiMe2]2O |
8 |
|
128 |
|
250 |
||||||||||||||||
(CH2DCHCH2SiMe2O)2SiMe2 |
8 |
|
55 |
|
250 |
aThe tungsten analogue of 8.
bWCI6/Me4Sn/PrOAc with 5-acetoxypent-1-ene as chain transfer agent. c 1,4-Polyisoprene with Z/E D 35/65.
dW(DCHC6H4-OMe-2)(DNC6H3-Me2-2,6)[OCMe(CF3)2]2 (thf). e55% linear oligomer C38% cyclic ‘monomer’.
f37% polymer C63% cyclic ‘monomer’; see Figure 2.
presence of 8W, to give the ‘dimer’, the molybdenum complex 8 is much more active and the end product is perfectly head-tail 1,4-polyisoprene (Z/E D 35/65). This is presumably formed by alternate reactions of the types represented by equations 48 and 49162.
|
|
|
|
|
|
C |
|
|
|
|
|
|
|
|
|
|
|
|
|
|
|
|
|
|
|
|
|
|
|
|
|
|
|
C |
|
|
|
|
|
|
|
|
|
|
|
|
|
|
|
|
|
||
|
[Mo] |
|
|
|
|
|
|
|
|
|
|
|
|
|
|
|
|
|
|
|
|
|
|
|
|
|
|
|
|
|
|
|
|
|
|
|
|
|
|
|
|
|
|
|
|
|
|
|
|
|
(48) |
||
|
|
|
C |
|
|
C |
|
|
C |
|
|
C |
|
|
C |
|
|
|
|
[Mo] |
|
|
|
C |
|
|
|
C |
|
|
|
C |
|
|
|
|
C |
|
|
C |
|||||||||||||
|
|
|
|
|
|
|
|
|
|
|
|
|
|
|
|
|
|
|
|
|
|
|
|
|
|
|
|
|
|
||||||||||||||||||||||||
|
+ |
|
|
|
|
|
|
|
|
|
|
|
|
|
|
+ |
|
|
|
|
|
|
|
|
|
|
|
|
|
|
|||||||||||||||||||||||
|
|
|
|
|
|
|
|
|
|
|
|
|
|
|
|
|
|
|
|
|
|
|
|
|
|
|
|
|
|
|
|
|
|||||||||||||||||||||
|
|
|
|
|
|
|
|
|
|
|
|
|
|
|
|
|
|
|
|
|
|
|
|
|
|
|
|
|
|
|
C |
|
|
C |
|
|
|
|
|
|
|
|
|
|
|
|
|
|
|
|
|
||
C |
|
|
C |
|
|
|
|
|
|
|
|
|
|
|
|
|
|
|
|
|
|
|
|
|
|
|
|
|
|
|
|
|
|
|
|
|
|
|
|
|
|||||||||||||
|
|
|
|
|
|
|
|
|
|
|
|
|
|
|
|
|
|
|
|
|
|
|
|
|
|
|
|
|
|
|
|
|
|
|
|
|
|
|
|
|
|
|
|
|
|
|
|
||||||
|
|
|
|
|
C |
|
|
|
|
|
|
|
|
|
|
|
|
|
|
|
|
|
|
|
|
|
|
|
|
|
C |
|
|
|
|
|
|
|
|
|
|
|
|
|
|
|
|
|
|||||
|
|
|
|
|
|
|
|
|
|
|
|
|
|
|
|
|
|
|
|
|
|
|
|
|
|
[Mo] |
|
|
|
|
|
|
|
|
|
|
|
|
|
|
|
|
|
|
|
|
|
||||||
|
|
|
|
|
|
|
|
|
|
|
|
|
|
|
|
|
|
|
|
|
|
|
|
|
|
|
|
|
|
|
|
|
|
|
|
|
|
|
|
|
|
|
|
|
|
|
|
|
|
||||
|
|
|
|
|
|
|
|
|
|
|
|
|
|
|
|
|
|
|
|
|
|
|
|
|
|
|
|
|
C |
|
|
C |
|
|
C |
|
|
C |
|
|
C |
|
|||||||||||
[Mo] |
|
|
C |
|
|
C |
|
|
C |
|
|
C |
|
|
C |
|
|
|
|
|
|
|
|
|
|
|
|||||||||||||||||||||||||||
|
|
|
|
|
|
|
|
|
|
|
|
|
|
|
|
|
|
|
|
|
|
|
|||||||||||||||||||||||||||||||
|
|
|
|
|
|
|
|
|
|
|
|
|
|
|
|
|
|
|
|
|
|
|
|
||||||||||||||||||||||||||||||
|
|
|
|
+ |
|
|
|
|
|
|
|
|
|
|
|
|
|
|
|
|
|
|
|
|
|
|
|
|
|
+ |
|
|
|
|
|
|
|
|
|
|
|
|
|
|
|
|
|
|
|
|
(49) |
||
|
|
|
|
|
|
|
|
|
|
|
|
|
|
|
|
|
|
|
|
|
|
|
|
|
|
|
|
|
|
|
|
|
|
|
|
|
|
|
|
|
|
|
|
|
|
|
|
|
|
||||
|
|
C |
C |
|
|
C |
|
|
|
C |
|
|
|
C |
|
|
|
C |
|
|
C |
C |
|
|
|
C |
|
|
C |
|
|
|
C |
|
|
|
C |
|
|||||||||||||||
|
|
|
|
|
|
|
|
|
|
|
|
|
|
|
|
|
|
|
|
|
|
|
|
|
|
|
|||||||||||||||||||||||||||
|
|
|
|
|
|
|
|
|
|
|
|
|
|
|
|
|
|
|
|
|
|
|
|||||||||||||||||||||||||||||||
|
|
|
|
|
|
|
|
|
|
|
|
|
|
|
|
|
|
|
|
|
|
|
|
|
|
|
|||||||||||||||||||||||||||
|
|
|
|
|
|
|
|
|
|
|
|
|
|
|
|
|
|
|
|
|
|
|
|
|
|
|
|
||||||||||||||||||||||||||
|
|
|
|
|
|
|
|
|
|
|
|
|
|
|
|
|
|
|
|
|
|
|
|
|
|
|
|
|
|
|
|
|
|
|
|
|
|
||||||||||||||||
|
|
|
|
|
|
|
|
|
|
|
|
|
|
|
|
|
|
|
|
|
|
|
|
|
|
|
|
|
|
|
|
|
|
|
|
|
|
|
|
|
|
|
|
|
|
|
|
|
|
|
|
|
|
C C
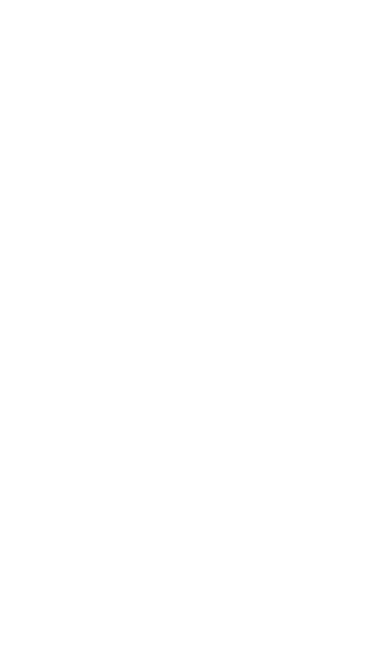
24. Advances in the metathesis of olefins |
1533 |
FIGURE 2. 50 MHz 13C NMR spectrum of the equilibrium products of metathesis reactions of diallyl ether catalysed by 8 (R D Me). Peaks 1 and 2: 2,5-dihydrofuran. Peaks 3 and 4: linear polymer (ca 100% trans). Peaks a, b and c: end-group carbons, a CH2CHDCH2, b CH2CHDCH2 and c CH2CHDCH2. Solvent CDCl3. Reproduced by permission of Huthig¨ & Wepf Publishers, Zug, Switzerland, from Ref. 262
FIGURE 3. 50 MHz 13C NMR spectrum of the 81% trans polymer obtained by the ADMET polymerization of 4,4,7,7-tetramethyl-4,7-disiladeca-1,9-diene. Reprinted with permission from Ref. 260. Copyright (1991) American Chemical Society
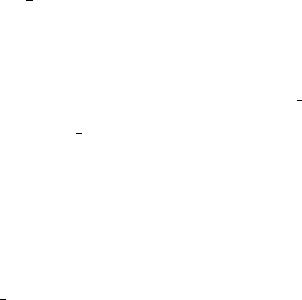
1534 K. J. Ivin
C. ADMET Copolymerization
Hexa-1,5-diene and deca-1,9-diene react together in the presence of 8W to form a copolymer containing [DCH(CH2)2CHD] and [DCH(CH2)6CHD] units, M1 and M2 respectively. In the 13C NMR spectrum of the copolymer, the olefinic carbons in the M1M2 junctions are clearly resolved from those in the M1M1 and M2M2 junctions and the intensities show that M1 and M2 units are distributed at random along the chains253. Copolymers have likewise been obtained in the reactions of deca-1,9-diene with ˛,ω- dienes containing keto234, ether263, ester264, thio267 and ferrocene268 groups. Copolymers can also be formed by placing a homopolymer derived from one diene in contact with the second diene in the presence of a metathesis catalyst. The double bonds in the polymer chain readily undergo secondary metathesis reactions with the second diene, leading to
the formation of a copolymer. |
|
Sometimes it is possible to make a copolymer |
from deca-1,9-diene and a |
second diene that will not itself undergo ADMET |
polymerization, for example |
with divinyldimethylsilane260 or with p-dipropenylbenzene269. In other cases the second diene refuses to copolymerize but instead undergoes RCM; this is so with (CH2DCHCH2CMe2)2CO as the second diene234.
VIII. RING-OPENING METATHESIS POLYMERIZATION (ROMP) OF
CYCLOALKENES
A. General Aspects
1. Ring chain equilibria
It is well known that in many cases of ROMP a series of cyclic oligomers is produced as well as linear polymer. If the catalyst is long-lived an equilibrium is eventually reached between the various cyclic species and the linear polymer. This may take some time to achieve. Thus, with cyclooctadiene as substrate the most abundant cyclic oligomeric species initially formed is (C4H6)4, but this eventually gives way to (C4H6)3 as a result of secondary metathesis reactions270. Sometimes the cyclic dimers are strongly favoured, as in the case of 68 (see Section VII.A.6) and cycloheptene271 273. The concentrations of the higher cyclic oligomers decrease with increasing ring size and provide a good test of the theory of ring chain equilibria developed by Jacobson and Stockmayer274. Although the original theory gives the correct form of dependence of equilibrium constant Kx on ring size x, the calculated values of Kx are too high; but when the theory is refined by the use of the rotational-isomeric-states model (with no adjustable parameters) the calculated values are in satisfactory agreement with experiment for rings containing more than about 30 atoms275. The discrepancy between theory and experiment for smaller rings is due to the failure of the assumption that the rings are unstrained. Their strain energy has been estimated by means of a Monte Carlo configurational search using molecular mechanics (MM3), leading to much better agreement between the calculated and experimental values of Kx for rings of all sizes276.
Strictly speaking, the value of Kx depends not only on x but also on the cis/trans ratio in the polymer and in the cyclic oligomer. However, the theory predicts that, in the range 20 90% trans, the effect will be very small275.
2. Use of transfer agents; telechelic polymers
Alk-1-enes are the preferred transfer agents when it is desired to reduce the molecular weight (MW) of polymers produced by ROMP in non-living systems. The transfer

24. Advances in the metathesis of olefins |
1535 |
constants are rather small and it is usually necessary to employ a fairly high concentration of transfer agent if a substantial reduction of MW is required, say to make the polymer easier to dissolve or to improve the resolution of its NMR spectrum. End groups derived from the transfer agent can be detected and identified277 279. Polymers with functional end-groups at both ends (telechelic polymers) can be prepared by the use of appropriate unsaturated transfer agents, preferably the more reactive cis isomers; for example diesters280, bis(silyl) ethers281, borane derivatives282 or protected diols185.
With living systems the MW of the polymer can be reduced by increasing [I]0, but this requires the use of large amounts of initiator. The same result can be achieved at low [I]0 by inclusion of a good transfer agent283.
The determination of transfer constants (ktr/kp) in living systems is not as straightfor-
ward as in non-living systems. A detailed consideration of the problem has been given129. The following serves to illustrate some of the complications that can be encountered. First suppose that ktr − kp ³ ki. If the concentration of the transfer agent is not very high the ROMP will proceed initially as though the transfer agent were not present and a living polymer will be formed with a number average degree of polymerization DPn equal to [M]0/[I]0. In time the transfer agent will react with the living polymer to give dead polymer of the same DP, and a new carbene species. Addition of a fresh batch of monomer would allow the process to be repeated and, provided that the initiation efficiency for the new carbene species was high, the second batch of polymer would again have a narrow molecular weight distribution (MWD). Such conditions are realized in practice with 7 (R D Me) as initiator, styrene (30 equiv) as transfer agent and norbornene added in eight successive batches of 80 equiv at 40 min intervals. The GPC of the total product shows a main peak (95% of the whole) with Mw/Mn D 1.07. When the monomer is 2,3-bis(trifluoromethyl)norbornadiene the product consists only of low-molecular-weight oligomers. This is because kp is much smaller and the transfer constant therefore much larger than for norbornene, so that the chains have no chance to grow to their full length before suffering reaction with the transfer agent283.
A different situation exists when the transfer constant is high and the amount of transfer agent used is such that it is all consumed before polymerization is complete. The remaining monomer will then polymerize in the absence of transfer agent and give rise to a fraction with a much higher MW than that of the initially formed polymer. The product will thus have a bimodal MWD. There may also be some cyclic oligomers formed by the backbiting reaction. The most reliable method of measuring the transfer rate constant ktr in such a system is to observe directly by NMR the rate of removal of chain transfer agent (CTA) by reaction with the propagating species. In this way the reaction of CH2DCHCMe3 with the propagating species in the ROMP of norbornene initiated by 7 (R D Ph) is found to
have ktr D 3 ð 10 5 M 1 s 1 at 22 °C compared with kp D 17 M 1 S 1. Once the rate constants are known the relative amounts of reagents in the reaction can be optimized to
secure the required MW and MWD. It will be evident that the slopes of (Mayo) plots of 1/(DPn) against [CTA]/[M]0 do not provide a reliable method of determining ktr/kp in
living systems unless care is taken to obviate the aforementioned pitfalls129.
3. Cis/trans blockiness
The distribution of the cis and trans double bonds in a given polymer chain may be expressed in terms of the ratios rt D tt / tc and rc D cc / ct . If the probability of formation of a cis double bond is independent of the configuration of the previous double bond, the distribution will be random (Bernoullian) and characterized by a single parameter rt D 1/rc. This is the case for polymers of norbornene with less than 35% cis content, but for polymers with more than 50% cis content the distribution is generally somewhat

1536 |
K. J. Ivin |
blocky, with rtrc reaching values of 8 or more in some cases. This pattern of behaviour is found not only for the ROMP of norbornene284 286 but also for its derivatives, and for cyclopentene287,288. A very significant observation is that rt seldom falls below unity even when the cis content is high. This means that at high cis content the trans double bonds tend to occur in pairs and are not to be found singly in the chain. To explain both the blockiness and the tt pair phenomenon it is necessary to postulate the presence of three kinetically distinct propagating species when the cis content is high. Detailed analysis of the proportions of double-bond triads in polynorbornene samples shows that the propagating species which have just formed a cis double bond (Pc) all have the same selectivity, but those which have just formed a trans double bond have different selectivity according to whether the last but one double bond formed was cis (Ptc) or trans (Ptt)285. It has been argued that Ptc and Ptt differ essentially in their ligand geometry as a result of the disrotatory modes of cleavage of the metallacyclobutane precursors. Ptt and Pc may differ more in the extent of crowding of the metal site by the polymer chain itself, which may or may not involve coordination of double bonds to the metal centre289. The cis content tends to fall and the blockiness to disappear as the monomer concentration is reduced290 or as the temperature is raised. This would suggest that, given time, the two species Ptc and Ptt become kinetically identical through relaxation to a species with common ligand geometry represented by Pt. Polymers of cyclooctene do not exhibit cis/trans blockiness, showing that lengthening of the repeat unit tends to eliminate the kinetic distinction between Pt and Pc291 293.
4. Head tail bias
The extent of HT bias in the polymers of substituted cycloalkenes is very dependent on (i) the location and nature of the substituent(s), and (ii) the catalyst. Monomers with a substituent at the double bond generally give strongly biased polymers with most catalysts. For example, 1-methylcyclobutene with W(DCPh2)(CO)5 gives an 85% cis polymer with HH : HT : TT D 1 : 8 : 1294. The presence of one or two substituents at the ˛-position results in fully biased polymers with some but not all catalysts. When the substituent(s) are further removed from the double bond there is usually very little HT bias in the polymer.
Some examples of monomers giving polymers with a completely regular HT structure are shown in Table 6. In most cases the polymers are either high-cis or high-trans and then have exceptionally simple 13C NMR spectra; (Figure 4). A few, however, are of intermediate cis content.
With the polymers of 1-methylnorbornene there is a remarkable variation of HT bias with catalyst. Thus RuCl3 at 60 °C gives an unbiased all-trans polymer (HH : HT : TT D 1 : 2 : 1), whereas IrCl3 at 75 ° gives a strongly biased high-trans polymer, the extent of bias increasing with dilution of the monomer. OsCl3 at 60 °C gives a polymer containing 16% cis double bonds which occur only in HT dyads, and 84% trans double bonds which occur mainly in HT dyads (HH : HT : TT D 1 : 11.3 : 1). Catalysts such as WCl6/R4Sn (R D Me, Bu) at 20 °C give polymers with 40 70% cis double bonds, but containing absolutely no cis HH structures. Figure 5 shows the olefinic region of the 13C NMR spectrum for a polymer of this kind, having a modest overall bias. Note thattrans HH D trans TT C cis TT , and that (cis HH) is not present. Models indicate that the formation of a cis HH structure would be extremely difficult303.
The magnitude of the HT bias is clearly determined by both polar and steric effects and may also be governed to some extent by relaxation processes between propagation steps. In living systems the head species PH (69) is generally present in higher concentration than the tail species PT (70)307. It is likely that in most, but not all, cases of total HT