
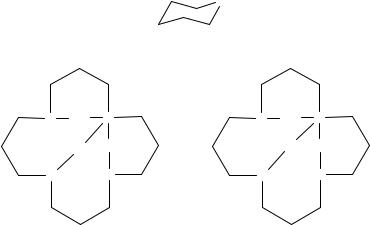
|
|
|
1. Molecular mechanics calculations |
|
11 |
||
|
|
|
|
|
NMe |
|
|
|
|
115.7 |
|
(6) |
|
|
|
|
1.515 |
1.515 |
|
|
112.5 |
|
|
|
1.450 |
112.9 |
112.5 |
|
115.5 |
|
|
|
1.451 |
|
|
1.461 |
|
||
112.6 |
114.4 N |
2.92 |
N 114.8 |
|
N 2.96 |
N 1.462 |
113.2 |
1.510 |
1.460 |
|
112.7 |
1.501 |
|
112.0 |
1.540 |
115.8 |
4.16 |
2.92 116.6 |
4.18 |
2.96 115.5 |
|
||
1.508 |
112.0N |
|
N 1.451 |
1.517 |
|
N 112.6 |
1.540 |
|
112.2 |
N |
113.1 |
||||
|
1.468 |
|
114.0 |
|
1.461 |
|
|
|
1.452 |
116.1 |
1.461 |
|
115.5 |
1.462 |
|
|
111.6 |
112.8 |
|
112.1 |
|
||
|
|
1.503 |
|
|
1.540 |
|
|
|
1.519 |
|
|
|
|||
|
|
|
|
|
|
||
|
|
X-ray |
|
(7) |
MM2 |
|
|
calculated and matched against the experimental heats of formation of 20 aliphatic amines in a least-squares manner to derive appropriate values for the aforementioned parameters. These were incorporated in the force field and used in all subsequent heat of formation calculations. A comparison between calculated and experimental results is provided in Table 4. As expected from a least-squares procedure, the agreement between experiment and calculation is generally very good with a standard deviation over 18 comparisons of 0.46 kcal mol 1 (two molecules, cyclobutylamine and 3-azabicyclo[3.2.2]nonane, were excluded from the above comparison since their experimental heats of formation are suspected to be erroneous5). However, the true test of these parameters, and indeed of all force field parameters developed in this and similar studies, should involve systems which were not included in the data set for the parameterization.
d. Dipole moments. Calculation of dipole moments for amines required the assignment of bond moments to the N lp, N H and N C bonds. This was done by fitting the calculated dipole moments of several aliphatic amines to the calculated ones via a leastsquares procedure. The results are presented in Table 5 and show good agreement between MM2 and experimental values. The only notable exception is quinuclidine, where the approximations inherent to the dipole moment calculation scheme employed in MM2 (neglect of induced dipole moments) have the largest effect.
3. MM2 0 parameterization of nitro compounds and MM2 parameterization of nitrosamines, nitramines, nitrates and oximes
Molecular mechanics calculations of the title compounds are much less common than those of amines, probably due to the lack of high quality parameters. In particular, none of these systems (save the nitro group, see Section II.C.3) has been parameterized during the original development of, and later additions to, the MM2 force fields by the Allinger group. Consequently, any serious attempt at modeling such systems must begin with the development of suitable parameters for their unique functionalities. In the following we list several examples where MM2 was parameterized for, and subsequently used in, the structural and energetic study of nitro compounds, nitrosamines, nitramines, nitrates and oximes.

12 |
Pinchas Aped and Hanoch Senderowitz |
TABLE 4. Heats of formation and standard deviations (SD) (kcal mol 1) for amino com-
pounds as calculated by the MM2 and MM3 force fields and observed by experimenta5,6 . Reprinted with permission from Refs. 5 and 6. Copyright (1985, 1990) American Chemical Society
Compound |
MM2 |
MM3 |
Experiment |
||
|
|
|
|
|
|
Methylamine |
5.10 |
5.04 |
|
5.50 |
|
Dimethylamine |
4.06 |
4.04 |
|
4.43 |
|
Trimethylamine |
6.15 |
6.09 |
|
5.76 |
|
Ethylamine |
11.82 |
11.92 |
|
11.35 |
|
Diethylamine |
17.60 |
17.41 |
|
17.33 |
|
Triethylamine |
21.66 |
21.49 |
|
22.17 |
|
n-propylamine |
16.89 |
16.95 |
|
16.77 |
|
Isopropylamine |
20.38 |
20.31 |
|
20.02 |
|
n-Butylamine |
21.95 |
21.85 |
|
21.98 |
|
sec-Butylamine |
24.79 |
24.31 |
|
25.06 |
|
Isobutylamine |
23.77 |
23.51 |
|
23.57 |
|
t-Butylamine |
28.90 |
28.90 |
|
28.90 |
|
Piperidine |
11.73 |
11.83 |
|
11.76 |
|
2-Methylpiperidine |
20.39 |
20.30 |
|
20.19 |
|
Cyclobutylamine |
11.10 |
9.90 |
|
|
9.90 |
Cyclopentylamine |
13.68 |
13.70 |
|
13.13 |
|
Quinuclidine |
0.98 |
1.29 |
|
1.03 |
|
Diisopropylamine |
|
31.67 |
|
34.41 |
|
Cyclohexylamine |
0.43 |
24.86 |
|
2.06 |
|
Pyrrolidine |
0.94 |
|
0.80 |
||
Azetidine |
7.21 |
24.62 |
|
|
24.62 |
3-Azabicyclo[3.2.2]nonane |
|
|
10.44 |
||
2,2,6,6-Tetramethyl-4-piperidone |
65.64 |
|
|
|
65.43 |
SDb |
0.46 (18) |
0.35 |
(20) |
|
aWhen a discrepancy occurred between the experimental data reported in References 5 and 6, the later value was used.
bNumber of comparisons are given in parentheses.
TABLE 5. Calculated (MM2) and observed dipole moments of amines (Debye)5 . Reprinted with permission from Ref. 5. Copyright (1985) American Chemical Society
Compound |
MM2 |
Experiment |
||||
|
|
|
|
|
|
|
Ammonia |
1.43 |
1.47 |
|
|
|
|
Methylamine |
1.33 |
1.30 |
|
|
|
|
Dimethylamine |
1.10 |
1.03 |
|
|
|
|
Trimethylamine |
0.64 |
0.63, 0.79 |
|
0.91 |
||
|
||||||
n-Propylamine |
1.33 |
1.17, 1.25 |
|
|
||
Isopropylamine |
1.33 |
1.20, 1.45 |
|
|
||
Ethylamine |
1.33 |
1.23 |
|
|
|
|
Diethylamine |
1.10 |
1.04 |
|
1.27 |
|
|
|
|
|
||||
Triethylamine |
0.64 |
0.67 |
|
1.02 |
|
|
|
|
|
||||
n-Butylamine |
1.33 |
1.33 |
|
1.45 |
|
|
|
|
|
||||
Pyrrolidine |
1.11 |
1.34, 1.44 |
|
|
||
N-Methylpiperidine |
0.64 |
0.80 |
|
1.34 |
|
|
|
|
|
||||
Piperidine |
1.10 |
1.05 |
|
1.35 |
|
|
|
|
|
||||
N-Methylpiperidine |
0.64 |
0.65 |
|
0.95 |
|
|
|
|
|
||||
2-Methylpiperidine |
1.10 |
1.171a |
||||
Quinuclidine |
0.64 |
1.17, 1.22, 1.57 |
a6-31G ab initio calculations.

1. Molecular mechanics calculations |
13 |
Parameters for the nitro group have been developed within the framework of the MM20 force field15. The nitro group was considered to be composed of a five-valent nitrogen connected to two oxygen atoms by two double bonds. Structural and energetic parameterization was based on the experimental structures of nitromethane, nitroethylene and nitrobenzene and on experimental heats of formation of nitromethane, nitroethane, nitropropane, nitrobutane, dinitromethane, trinitromethane and nitrobenzene, respectively. Parameters were determined by a least-squares fit procedure. The resulting force field faithfully reproduced the experimental data for all the molecules used in the parameterization data set with an average absolute error between experiment and calculation of 0.009 A˚ (8 comparisons) for bond lengths, 0.7° (8 comparisons) for bond angles, 0.015 D (2 comparisons) for dipole moments and 1.0 kcal mol 1 (7 comparisons) for heats of formation. A complete list of parameters and force field results is given in Reference 15.
Delpeyroux and coworkers16 have developed a set of molecular mechanics parameters for nitramines (R N NO2) for the EMO program and used it in conjunction with MM2-85 parameters to calculate the structures of 1,4-dinitro-glycoluryl (8), 1,3-dinitro- 4,6-diacetylglycoluryl (9) and 2,5,7,9-tetranitro-tetraazabicyclo(4.3.0)nonanone (10). The complete parameter list for the nitramine functionality is provided in Reference 16 but the parameterization procedure is not discussed.
H |
|
NO2 |
Ac |
NO2 |
N |
|
N |
N |
N |
O |
|
O O |
|
O |
N |
|
N |
N |
N |
NO2 |
|
H |
Ac |
NO2 |
|
(8) |
|
|
(9) |
|
NO2 |
NO2 |
|
|
|
|
|
|
|
|
N |
N |
|
|
|
|
|
|
|
|
|
O |
|
NO |
|
|
|
|
|
|
N |
N |
|
N |
|
|
|
|
|
|
NO2 |
NO2 |
|
|
|
|
|
|
|
|
|
(10) |
|
(11) |
A set of MM2 (QCPE 395, 1980) parameters for nitrosamines (R N NO) was developed by Polonski and coworkers17 in the course of their study on the conformational dependence of Circular Dichroism of N-nitrosopyrolidines (11). Parameters for the N N torsion were obtained by fitting the barrier to rotation of the nitroso group as determined from NMR measurements for similar systems (ca 23 kcal mol 1). Other parameters involving the Nsp2 were taken from a set used for azoalkanes18. N-Nitrosodimethylamine ((CH3)2N NO) was chosen as a model compound and its gas-phase electron diffraction structure was used to determine ‘natural’ bond lengths and angles. The remaining stretching and bending parameters were determined according to Pearlstein and Hopfinger19 where the corresponding potential functions for N-nitrosodimethylamine were calculated

14 Pinchas Aped and Hanoch Senderowitz
with MNDO. Bond dipole moments were estimated from partial atomic charges obtained by a Mulliken population analysis of an ab initio wavefunction. The complete nitrosamines parameters set is provided in Reference 17.
MM2-85 and MM2-87 parameters for a five-membered heterocyclic aromatic ring incorporating the N O unit and for conjugated oximes (R D N O H) were developed by Kooijman and colleagues as part of their work on muscarinic agonists20. Parameters were derived based on Cambridge Structural Database statistics and semiempirical calculations, but the derivation procedure is not discussed by these authors. The new parameters are claimed to reproduce bond lengths and angles in a set of appropriate test structures retrieved from the Cambridge Structural Database to within 0.02 A˚ and 3° of the experimental data and to reproduce the observed dipole moments for a set of five-membered heterocyclic rings to within 0.4 D, but no detailed comparison is provided in the paper. A complete list of the new parameter is available in Reference 20.
Parameters for nitrates (R O NO2) have been developed for the MM2-85 force field by Wang and coworkers21. Force constants and ‘natural’ values for bond lengths and angles involving the nitrate group were obtained from the microwave structure of methyl nitrate and ethyl nitrate. The force constant, ‘natural’ bond length and dipole moment for the C O bond were modified from the MM2 original ones to account for the electron-withdrawing properties of the NO2 group. Torsional parameters for rotation around the HC- - -ONO2 and CC- - -ONO2 bonds were obtained by fitting the experimental barrier to rotation of methyl nitrate and ethyl nitrate, respectively. Those for rotation around the HCO- - -NO2 and CDC- - -CONO2 bonds were obtained by fitting the corresponding torsional profiles of methyl nitrate and propenyl nitrate as calculated by MINDO/3. Finally, heat of formation parameters for N O and NDO were obtained by fitting the experimental values for methyl nitrate, ethyl nitrate and 1,2,3-propanetriol trinitrate. A comparison between force field results and experimental data reveals moderately reasonable reproduction of the latter for all systems used in the data set for the parameterization. In particular, bond lengths and angles (heavy atoms only) for methyl nitrate and ethyl nitrate are reproduced to within 0.01 A˚ and 7° from their respective microwave values, those for 1,2,3-propanetriol trinitrate to within 0.02 A˚ and 5° from their X-ray values and dipole moments and heats of formation for methyl nitrate, ethyl nitrate and 1,2,3-propanetriol trinitrate are reproduced to within 0.3 D and 0.4 kcal mol 1. The trans gauche energy difference of ethyl nitrate is also in very good agreement with the experimental value (0.5 kcal mol 1 from both experiment and calculations). However, the performance of these parameters for other nitrates could not be evaluated since the only other compounds calculated in this work, isopropyl nitrate, propenyl nitrate and benzyl nitrate, have not been experimentally reported at the time of its publication. A complete list of the new force field parameters is provided in Reference 21.
4. MM2 parameterization of nitro compounds, enamines and aniline derivatives
Parameterization of MM2 for nitro compounds, enamines and aniline derivatives has been performed in conjunction with the parameterization of MM3 and will be discussed in Sections II.C.3 and II.C.4.
5. MM2 parameterization of the N C N anomeric moiety
Although the treatment of stereoelectronic effects is somewhat beyond the traditional capabilities of molecular mechanics, force fields can be suitably parameterized to reproduce their energetic and structural manifestations. In the following, we discuss the parameterization of MM2-80 and MM2-87 for the anomeric effect characteristic of the N C N moiety22.

1. Molecular mechanics calculations |
15 |
The anomeric effects23 was first observed in carbohydrates and defined as the preference of an electronegative substituent at the anomeric center of a pyranose ring for the axial (12) rather than the equatorial (13) position, in contrast to what is expected from steric considerations. Extensive theoretical and experimental work23,24 has subsequently revealed the generality of the phenomenon and the effect was redefined as the tendency of an R X C Y moiety (X D O, N, S; Y D OR, NR2, halogen) to adopt gauche rather than anti conformation around the X C and when present, C Y bonds (the latter referred to as the exo-anomeric effect). The currently most accepted explanation of the anomeric effect is given in Molecular Orbitals terms25 and invokes delocalization of a lone pair situated on X into the adjacent Ł C Y orbital (14). This is a two-electron two-orbital stabilizing interaction whose magnitude depends on the relative orientation of the participating orbitals and on the energy gap between them. Thus, attention is shifted from an R X C Y gauche orientation to an X C Y lp anti one. The manifestations of the anomeric effect, relevant to molecular mechanics calculations, are twofold: (1) energetic preference of conformers having a lp antiperiplanar to a Ł orbital; (2) changes in bond lengths and angles as a results of such lp Ł interaction.
O |
O |
|
X |
|
|
+ |
|
|
X |
|
|
X |
|
|
|
R |
C |
R |
C |
|
|
|
|
||||
X |
|
|
|
Y |
|
Y− |
|
|
|
|
|
R′ |
R′ |
|
|
|
|
|
|
|
(12) |
(13) |
|
|
|
(14) |
|
Several studies have been devoted to the parameterization of molecular mechanics force fields for the anomeric effect22,26. Here we concentrate on the works of the Tel Aviv group relevant to amines, namely the modification and parameterization of MM280 and, later, MM2-87 to allow the treatment of stereoelectronic effects characteristic of the R N C N R (R D H, alkyl) moiety22. These include: (1) energetic preference of conformers with a nitrogen lp antiperiplanar to an adjacent C N bond; (2) energetic preference of conformers with an intramolecular hydrogen bond; (3) structural changes in the R N C N R moiety where an N2 C3 N4 lp antiperiplanar arrangement results in shortening of the C3 N4 bond, elongation of the N2 C3 bond and opening of the C1 N2 C3 and N2 C3 N4 bond angles; (4) changes in C N bond lengths to tertiary amines incorporated in anomeric moieties. It has long been recognized22 25 that the anomeric effect in the N C N moiety is weaker than that in O C O due to the poorer - acceptor characteristics of the C N bond and the consequent weaker lpN Ł C N overlap. As a result its energetic and structural manifestations are expected to be less pronounced than in the case of the oxygen analog.
Most of the data for these parameterization studies came from ab initio calculations although other sources were also used, in particular, to validate the resulting force field. Thus a set of small model molecules with different conformations of the R N C N R moiety was calculated at various levels of theory and the results used to derive torsional parameters, hydrogen bond parameters and conformationally dependent correction terms for ‘natural’ bond lengths and angles, as described below:
Torsional parameters. These (in conjunction with hydrogen bonding parameters, see below) were chosen to reproduce the ab initio conformational energies of the model compounds.
Hydrogen bonds. When an N C N moiety has hydrogen atoms on either nitrogens, several of its conformations may be stabilized by intramolecular hydrogen bonds. Since MM2 does not have a special potential function for hydrogen bonding, such interactions

16 |
Pinchas Aped and Hanoch Senderowitz |
were originally treated, in a nondirectional manner, by assigning special VdW parameters to the H. . .N atom pair. This treatment has been replaced by directional hydrogen bonding where the VdW ε parameter (equation 7) is correlated with the geometry of the hydrogen bond according to:
ε D ε0 G exp DR |
10 |
where ε is a new VdW energy parameter, DR D abs r r0 and G is a geometrical fit term given by:
For the N lp. . .H N interaction:
G D cos ω |
0 < ω < 80 |
(11) |
46.26240356 exp 4ω |
80 < ω < 180 |
|
where ω is the lp N. . .N H torsion. |
|
|
For the N lp. . .H(C N) interaction: |
|
|
G D 1 cos ˛ 1 cos ˇ |
|
(12) |
In equation 12, ˛ is the N lp . . . H angle and ˇ is the lp . . . H C angle.
Structural parameters. Variations of bond lengths and bond angles in the C1 N2 C3 N4 C5 moiety as a result of the aforementioned lpN CŁ N overlap were
introduced into MM2 by deriving conformationally dependent correction terms for r0 and 0. This treatment circumvented the electronegativity correction to bond lengths implemented in MM2-87 (Sections II.B.1 and II.C.1).
Inner C N bonds. r0 was made a function of the geometry of the anomeric moiety according to:
r00 D r0 υr |
13 |
where, for the N2 C3 bond, for example, υr is given by:
υr D 0.5K1[1 C cos 2ω23 ] 0.5K2[1 C cos 2ω34 ] C d |
14 |
where ω23 and ω34 are the lp N2 C3 N4 and N2 C3 N4 lp torsions. The first term in equation 14 causes r0 shortening while the second causes its elongation; d is used to correct for conformationally independent bond length variations such as bond shortening, known to appear when several heteroatoms are connected to the same carbon8. The values of K1, K2 and d were determined by fitting MM2 results to the ab initio values.
Outer C N bonds. Outer C N bonds in the R N C N R moiety are known22,26 to inversely depend on the adjacent inner bond lengths probably due to a hybridization effect. ‘Natural’ bond lengths for those bonds were determined according to:
r00 D r0 C D |
(15) |
D D aυr C b |
(16) |
where υr is the change in the adjacent inner bond while a and b were determined as before, by fitting ab initio results.
C N bond lengths in tertiary amines. Experimental and ab initio calculations have demonstrated the gradual decrease in C N bond lengths when going from primary (CH3NH2) to secondary ((CH3)2NH) to tertiary ((CH3)3N) amines5. However, when a tertiary amine is incorporated in an anomeric unit, the cumulative effect of anomeric interactions, steric interactions and conformationally independent C X bond shortening8 add up to level off this trend. Moreover, a statistical analysis of C N bond lengths in

1. Molecular mechanics calculations |
17 |
primary, secondary and tertiary amines retrieved from the Cambridge Structural Database revealed only negligible differences22b. Correction terms for tertiary C N ‘natural’ bond lengths were derived for the N C N moiety based on a comparison with the results of ab initio calculations of suitable model systems.
N C N bond angles. The ‘natural’ value ( 0) for the N C N bond angle was determined from ab initio calculations of suitable model compounds. Since this angle depends on the conformation of the anomeric moiety, conformationally dependent correction terms for 0 were derived according to:
00 D 0 C υ |
(17) |
υ D 0.5K1[cos 2ω23 1] C 0.5K2[cos 2ω34 1] |
(18) |
where ω23 and ω34 are defined as in equation 14 and K1 and K2 determined by fitting ab initio results.
C N C bond angles. Theory predicts22 25 an opening of these angles as a result of an anomeric interaction. Thus conformationally dependent correction terms for 0 were derived according to:
00 D 0 C υ |
(19) |
υ D 0.5K1[1 C cos 2ω23 ] C 0.5K2[1 C cos 2ω34 ] |
(20) |
where ω23 and ω34 are defined as in equation 14 and K1 and K2 determined by fitting ab initio results.
Torsional parameters and VdW parameters for internal hydrogen bonds in the N C N moiety were obtained by fitting the ab initio rotational profiles of methylenediamine (MDA, 15) and N-methylmethylenediamine (NMMDA, 16). A comparison of relative conformational energies between ab initio and MM2 results for 15 and 16 is provided in Table 6. Bond length correction terms for inner and outer C N bonds (K1, K2 and
TABLE 6. Relative energies (kcal mol 1) of all possible conformers of methylenediamine (MDA, 15) and N- methylmethylenediamine (NMMDA, 16) as calculated ab initio (HF/3-21G//HF/3-21G) and with the reparameterized force field (MM2-87 version)a . Reproduced from Ref. 22b by permission of Elsevier Science Ltd
|
ab initio |
MM2 |
|
|
|
MDA (15) |
|
|
aa |
0.00 |
0.00 |
ag |
1.62 |
1.21 |
gC gC |
2.70 |
3.39 |
gC g |
8.23 |
5.56 |
NMMDA (16) |
|
|
aa |
0.00 |
0.00 |
ag |
1.23 |
1.46 |
agC |
1.69 |
1.95 |
gC a |
1.50 |
1.36 |
g g |
2.27 |
4.10 |
gC gC |
2.48 |
3.55 |
aConformers of the lp N2 C3 N4 lp moiety are |
defined |
|
via two torsional angles: D1 |
D lp N2 C3 N4; |
D2 D |
N2 C3 N4 lp, a D anti, g D gauche22b. |
|

TABLE 7. Selected structural parameters (bond lengths in A,˚ bond angles and torsional angles in degrees) of methylenediamine (MDA, 15), N-methylmethylene- diamine (NMMDA, 16), N,N-dimethylmethylenediamine (NNDMMDA, 17) and tetramethylmethylenediamine (TMMDA, 18) as calculated ab initio (ai, HF/3- 21G//HF/3-21G) and with the reparameterized force field (MM2-87 version)a . Reproduced from Ref. 22b by permission of Elsevier Science Ltd
MDA (15) |
aa |
|
|
ag |
gC gC |
|
gC g |
|
|
|
|
|
N1 C2 |
ai |
MM2 |
ai |
MM2 |
ai |
MM2 |
ai |
MM2 |
|
|
|
|
1.466 |
1.468 |
1.459 |
1.458 |
1.461 |
1.460 |
1.464 |
1.461 |
|
|
|
|
|
C2 N3 |
1.466 |
1.468 |
1.470 |
1.470 |
1.461 |
1.460 |
1.464 |
1.461 |
|
|
|
|
N1 C2 N3 |
118.3 |
117.8 |
113.4 |
113.6 |
106.9 |
107.9 |
110.4 |
108.7 |
|
|
|
|
NMMDA (16) |
aa |
|
|
aa |
agC |
|
gC a |
|
|
g g |
|
gC gC |
N1 C2 |
ai |
MM2 |
ai |
MM2 |
ai |
MM2 |
ai |
MM2 |
ai |
MM2 |
ai |
MM2 |
1.464 |
1.469 |
1.457 |
1.458 |
1.455 |
1.458 |
1.468 |
1.472 |
1.461 |
1.464 |
1.460 |
1.461 |
|
C2 N3 |
1.464 |
1.466 |
1.467 |
1.469 |
1.471 |
1.469 |
1.457 |
1.456 |
1.458 |
1.459 |
1.458 |
1.459 |
N3 C4 |
1.467 |
1.467 |
1.465 |
1.468 |
1.467 |
1.468 |
1.470 |
1.469 |
1.467 |
1.469 |
1.464 |
1.468 |
N1 C2 N3 |
118.2 |
118.4 |
113.8 |
114.3 |
113.3 |
113.1 |
114.4 |
114.4 |
108.5 |
109.8 |
107.5 |
108.7 |
C2 N3 C4 |
115.9 |
112.3 |
115.7 |
112.6 |
114.9 |
112.4 |
114.4 |
114.0 |
115.0 |
114.2 |
115.6 |
112.1 |
N1 C2 N3 C4 |
61.9 |
61.2 |
70.9 |
66.9 |
172.3 |
175.8 |
57.8 |
66.1 |
66.2 |
67.1 |
179.0 |
179.9 |
NNDMMDA (17) |
aa |
|
|
ag |
gC gC |
|
|
|
|
|
|
|
N1 C2 |
ai |
MM2 |
ai |
MM2 |
ai |
MM2 |
|
|
|
|
|
|
1.461 |
1.470 |
1.452 |
1.460 |
1.459 |
1.466 |
|
|
|
|
|
|
|
C2 N3 |
1.465 |
1.462 |
1.472 |
1.466 |
1.460 |
1.457 |
|
|
|
|
|
|
N3 C4 |
1.464 |
1.461 |
1.465 |
1.463 |
1.463 |
1.465 |
|
|
|
|
|
|
N3 C5 |
1.464 |
1.461 |
1.464 |
1.462 |
1.465 |
1.465 |
|
|
|
|
|
|
N1 C2 N3 |
118.0 |
119.2 |
114.5 |
114.3 |
109.2 |
110.1 |
|
|
|
|
|
|
C2 N3 C4 |
114.4 |
111.0 |
112.9 |
110.7 |
113.6 |
109.5 |
|
|
|
|
|
|
C2 N3 C5 |
114.4 |
111.0 |
113.8 |
111.2 |
113.5 |
112.8 |
|
|
|
|
|
|
N1 C2 N3 C4 |
66.8 |
61.9 |
166.3 |
172.5 |
167.1 |
171.7 |
|
|
|
|
|
|
N1 C2 N3 C5 |
66.0 |
62.1 |
63.4 |
64.4 |
61.7 |
65.8 |
|
|
|
|
|
|
TMMDA (18) |
C Cb |
|
|
|
|
|
|
|
|
|
|
|
g g |
|
|
|
|
|
|
|
|
|
|
|
|
N1 C2 |
ai |
MM2 |
|
|
|
|
|
|
|
|
|
|
1.458 |
1.466 |
|
|
|
|
|
|
|
|
|
|
|
N1 C4 |
1.463 |
1.466 |
|
|
|
|
|
|
|
|
|
|
N1 C5 |
1.465 |
1.466 |
|
|
|
|
|
|
|
|
|
|
N1 C2 N3 |
110.4 |
114.0 |
|
|
|
|
|
|
|
|
|
|
C4 N1 C2 |
113.9 |
109.2 |
|
|
|
|
|
|
|
|
|
|
C5 N1 C2 |
113.1 |
113.6 |
|
|
|
|
|
|
|
|
|
|
C4 N1 C2 N3 |
165.9 |
177.2 |
|
|
|
|
|
|
|
|
|
|
C5 N1 C2 N3 |
63.5 |
60.4 |
|
|
|
|
|
|
|
|
|
|
aConformers of the lp N2 C3 N4 lp moiety are defined via two torsional angles: D1 D lp N2 C3 N4; D2 D N2 C3 N4 lp, a D anti, g D gauche22b. bThe only conformer of this molecule observed experimentally.
18

1. Molecular mechanics calculations |
19 |
d of equation 14 and a and b of equation 16) were derived through fitting MM2 results to ab initio geometries of 15, 16, N,N-dimethylmethylenediamine (NNDMMDA, 17) and tertramethylmethylenediamine (TMMDA, 18). The latter two molecules were also used to derive correction terms for fitting tertiary C N bonds. A ‘natural’ value for the N C N angle and correction terms for the N C N and C N C angles were derived in a similar manner. A comparison of selected structural parameters between ab initio and MM2 results for 15 18 is provided in Table 7 and a complete list of the parameters is given in References 22a and 22b.
NH2 |
NH2 |
NH2 |
NHMe NH2 |
N(Me)2 (Me)2 N |
N(Me)2 |
(15) |
|
(16) |
(17) |
|
(18) |
The performance of the modified force field was evaluated by comparing calculated and experimental relative stability of a series of 1,3-diaza cyclic compounds (19 24).
R |
NR′ |
|
|
|
|
|
|
|
|
|
|
|
|
|
|
|
N |
|
a |
b |
c |
d |
e |
f |
g |
|
|
|
|
|
|||
|
|
|
|
|
|
|
|
|
||||||||
|
|
|
|
|
|
|
|
|
|
|
|
|
|
|||
|
|
R |
= |
H |
Me |
t-Bu |
Me Et i-Pr |
t-Bu |
|
|
|
|||||
(19) |
|
R′ |
= |
H |
H |
H |
Me |
Et |
i-Pr Me |
|
|
|
|
|
||
Me |
|
|
|
|
|
|
|
|
|
|
|
|
Me |
|
|
|
|
|
|
|
|
Me |
|
|
|
|
|
|
|
|
|||
N |
R′ |
|
|
|
N |
R′ |
|
|
Me |
|
|
N |
|
Me |
||
N |
Me |
|
|
|
N |
|
|
|
|
|
|
|
N |
|||
|
|
|
|
|
|
|
|
|
|
|
|
|||||
R′ = Me |
|
|
|
|
|
Me |
|
|
|
Me |
|
|
|
|
|
|
(20) |
|
|
|
|
|
|
|
|
(21) |
|
|
|
||||
Me |
|
|
|
|
|
|
|
|
|
|
|
|
|
|
||
N |
|
|
|
|
|
R |
|
|
|
|
|
|
|
|
|
|
N |
|
|
|
|
|
|
|
|
|
|
|
|
|
|||
|
|
|
N |
|
|
|
a |
b |
c |
d |
|
|
||||
|
|
|
|
|
|
R′ |
|
|
|
|
|
|
|
|
|
|
|
|
|
|
|
|
N |
|
R = H Me H |
Me |
|||||||
|
|
|
|
|
|
|
|
|||||||||
|
|
|
|
|
|
|
|
|
R′ = H H |
Me |
Me |
(22)(23)
|
|
15 |
16 |
|
Me |
|
|
N |
2 |
14 |
|
|
||
|
|
1 |
N |
|
N |
|
|
3 |
|
8 |
|
N |
||
7 |
|
9 |
|
|
|
N |
|
N 4 |
N |
6 |
N |
|
|
|
N |
5 |
10 |
N |
|
|
||||
12 |
|
|
||
|
|
11 |
|
|
Me |
13 |
|
|
|
|
|
|
||
(24) |
(25) |
|
|
(26) |

20 |
Pinchas Aped and Hanoch Senderowitz |
TABLE 8. Conformational energies (kcal mol 1) of 1,3-diazacyclic compounds (19 24) as calculated by the modified MM2 force field (MM2-80 version) and observed experimentally (eq. equatorial; ax. axial)22a. Reproduced by permission of John Wiley & Sons, Inc.
System |
eq.eq. |
eq.ax. |
ax.eq. |
ax.ax. |
|
|
|
|
|
19a-Calc. |
4.9 |
1.1 |
|
0.0 |
19b-Calc. |
3.4 |
0.0 |
1.4 |
0.1 |
19b-Exp. |
|
N-Hax predominant |
|
|
19c-Calc. |
3.5 |
0.0 |
5.5 |
4.7 |
19c-Exp. |
|
N-Hax ¾ 66% |
|
|
19d-Calc. |
1.76 |
0.0 |
|
4.03 |
19d-Exp. |
favored |
|
|
|
19e-Calc. |
1.9 |
0.0 |
|
4.0 |
19e-Exp. |
favored |
|
|
|
19f-Calc. |
2.5 |
0.0 |
|
5.8 |
19f-Exp. |
favored |
|
|
|
19g-Calc. |
1.9 |
0.0 |
|
|
19g-Exp. |
favored |
|
|
|
20(R0 D eq)-Calc. |
3.7 |
0.0 |
|
3.8 |
20(R0 D eq)-Exp. |
|
favored |
|
|
20(R0 D ax)-Calc. |
4.2 |
1.0 |
|
4.0 |
21-Calc. |
0.0 |
1.8 |
|
|
21-Exp. |
100% |
|
|
|
22-Calc. |
0.0 |
|
|
14.3 |
22-Exp. |
100% |
|
|
|
23a-Calc. |
4.9 |
1.1 |
1.2 |
0.0 |
23a-Exp. |
|
|
|
favored |
23b-Calc. |
3.6 |
0.1 |
1.2 |
0.0 |
23b-Exp. |
60% |
|
|
|
23c-Calc. |
3.4 |
1.5 |
0.0 |
0.2 |
23c-Exp. |
25% |
|
|
|
23d-Calc. |
2.11 |
0.41 |
0.0 |
3.92 |
23d-Exp. |
40% |
|
|
|
24-Calc. |
4.3 |
0.0 |
|
1.4 |
24-Exp. |
|
100% |
|
|
|
|
|
|
|
The results, presented in Table 8, show that in most cases the conformer with the lowest steric energy indeed corresponds to the experimentally most favored one. In addition, several molecules containing the N C N moiety were retrieved from the Cambridge Structural Database and calculated with the new parameter set. A comparison between MM2 and X-ray geometries (selected structural parameters only) for two conformers of 1,4,5,8-tetraazadecalin (25, 26) is provided in Table 9 and shows good fit between the experimental and calculated data.
Other nitrogen-containing anomeric moieties, similarly treated within the MM2 framework, are N C O27,28 and N C F29. These works, however, exceed the scope of this chapter and will not be discussed here.
C. MM3
The MM3 force field4 was developed in order to correct for some of the basic limitations and flows in MM2 by providing a better description of the molecular potential surface in terms of the potential functions and the parameters. One major outcome of the improved force field is the omission of lone pairs on nitrogen and oxygen since the reason for their inclusion in MM2 was no longer pertinent. This allows for a realistic