

1. Molecular mechanics calculations |
31 |
|||
TABLE 16. (continued) |
|
|
|
|
|
|
|
|
|
|
MM2 |
MM3 |
Experiment |
|
|
|
|
|
|
nitroethylene |
127.1 |
126.6 |
126.0 |
|
nitrobenzene |
126.2 |
126.1 |
123.4 |
|
range |
3.7 |
1.4 |
7.8 |
|
Dihedral Angles (deg) |
|
|
|
|
C C N O |
|
|
|
|
2-nitropropane |
61.6 |
60.7 |
42.1 |
|
|
60.9 |
60.7 |
77.9 |
|
2-methyl-2-nitropropane |
120.4 |
120.2 |
|
|
|
0.3 |
0.0 |
16.5 |
|
|
119.9 |
120.2 |
|
|
nitroethylene |
0.0 |
0.0 |
0.0 |
|
nitrobenzene |
0.0 |
0.0 |
0.0 |
|
Dipole Moments (D) |
|
|
|
|
nitromethane |
3.61 |
3.49 |
3.46 |
|
2-nitropropane |
3.63 |
3.60 |
3.75 |
|
2-methyl-2-nitropropane |
3.67 |
3.63 |
3.74 |
|
nitrocyclopropane |
3.68 |
3.94 |
3.95 |
|
nitroethylene |
3.95 |
3.78 |
3.70 |
|
nitrobenzene |
3.99 |
3.81 |
4.01 |
|
range |
0.38 |
0.45 |
0.55 |
|
aFor each structural parameter, the difference between the two extreme values is provided at the end of the list. (The ‘range’ columns were added by the present authors).
range than either force field results. Thus, for example, while the O N O bond angle is always calculated to be in the range of 125.3° 129.0° and 126.0° 127.4° for MM2 and MM3, respectively, the corresponding experimental numbers are 122.2° 130.0°. Similar trends are observed for all other structural parameters as well as for the dipole moments, and suggests that the force fields are perhaps not sensitive enough to the different chemical environments of the nitro groups in these systems.
b. Rotational barriers. A comparison of the rotational barriers of the nitro group in several aliphatic and aromatic nitro compounds between experimental data and MM2 and MM3 calculations is provided in Table 17. The data are clearly divided into two groups.
TABLE 17. Nitro group rotational barriers (cm 1) for the set of nitro compounds used in the parameterization of MM2 and MM3 as obtained by the two force fields and from experiment43. Reproduced by permission of Elsevier Science from Ref. 43
|
MM2 |
MM3 |
Experiment |
|
|
|
|
CH3 NO2 |
18 |
2 |
6 |
CH3CH2 NO2 |
130 |
19 |
<14 |
(CH3)2CH NO2 |
267 |
150 |
0 |
(CH3)3 NO2 |
3 |
28 |
202 |
cyclo-C3H5 NO2 |
2945 |
3020 |
3300a |
CH2CH NO2 |
3419 |
3800 |
6510 |
C6H5 NO2 |
2974 |
3243 |
3260 |
aEstimated from IR data and not from microwave data.

32 |
Pinchas Aped and Hanoch Senderowitz |
While aliphatic nitro compounds are essentially free rotors around the C N bond with experimental and calculated barriers to rotation in the order of 0 0.3 kcal mol 1, the conjugated systems, namely nitrobenzene, nitroethylene and nitrocyclopropane (where the conjugation is between the p orbital of the nitro group and the system of the cyclopropane ring), have much higher barriers of several kcal mol 1. Both experimental and the molecular mechanics results suggest that while the geometry of nitrobenzene and nitroethylene is planar, the nitro group in nitrocyclopropane bisects the plane of the cyclopropyl ring. The overall agreement between experiment and calculation is very good.
c. Vibrational spectra. A complete list of the vibrational frequencies for the 8 molecules used in this parameterization work is given in Reference 43. The overall agreement between experiment and calculation is reasonable with a root-mean-square error over 8 compounds of 57 cm 1. The planar systems show more coupling between different vibrational modes and are therefore more dependent on cross-terms in the vibrational calculation. Since only few cross-terms are included in the MM3 version employed here (MM3-90), the vibrational frequencies of the planar systems are reproduced to a smaller degree of accuracy than the nonplanar ones. The fit between experiment and calculation for C H frequencies in nitro compounds is greatly improved upon inclusion of electronegativity corrections for bond lengths and force constant as implemented in MM3-94. The RMS error for a set of 5 nitroalkanes (nitromethane, dinitromethane, trinitromethane, nitroethane, 2-nitropropane) is reduced from 69.7 cm 1 to 33.0 cm 1, close to that obtained for the hydrocarbon part of the force field31. The nitro group is strongly electron-withdrawing and consequently exerts a strong shortening effect on C H bonds, hence the dramatic improvement.
d. Heats of formation. Heats of formation for nitro compounds were calculated with the bond-energy scheme1. Values for five parameters (C N and N O bond energies and structural terms for primary, iso and tertiary groups connected to the nitrogen of the nitro group) were obtained through a least-squares fit to the experimental heats of formation of 7 nitroalkanes. Because of the paucity of the experimental data, the accuracy of the numbers is claimed to be somewhat limited43. A comparison between the observed and calculated heats of formation for the molecules in the data set is provided in Table 18 and reveals good fit between theory and experiment. The standard deviation over 7 comparisons is 0.28 and 0.26 kcal mol 1 for MM2 and MM3, respectively.
TABLE 18. Heats of formation and standard deviations (SD) (kcal mol 1) for nitro compounds as calculated by the MM2 and MM3 force fields and observed by experiment43. Reproduced by permission of Elsevier Science Ltd from Ref. 43
|
|
MM2 |
|
MM3 |
Experiment |
|||
|
|
|
|
|
|
|||
Nitromethane |
17.76 |
|
17.76 |
|
17.76 |
|||
Nitroethane |
24.47 |
|
24.45 |
|
24.45 |
|||
1-Nitropropane |
29.86 |
|
29.59 |
|
29.59 |
|||
2-Nitropropane |
33.66 |
|
33.22 |
|
33.22 |
|||
2-Methyl-2-nitropropane |
42.33 |
|
42.33 |
|
42.33 |
|||
1-Nitrobutane |
34.09 |
|
34.39 |
|
34.39 |
|||
Nitrobutane |
|
38.66 |
|
|
39.10 |
|
|
39.10 |
2- a |
0.28 |
(7) |
0.26 |
(7) |
|
|||
SD |
|
|
|
|
aNumber of comparisons are given in parentheses.

1. Molecular mechanics calculations |
33 |
4. MM2 and MM3 parameterization of enamines and aniline derivatives44
The parameterization of MM2 and MM3 for enamines and aniline derivatives was based on the experimental and ab initio results of vinylamine, N-methylvinylamine, aniline, N- methylaniline and N,N-dimethylaniline. A complete list of parameters for both force fields is provided in Reference 44.
a. Structure. Stretching parameters for the C N bond and bending parameters for the C C N, C N C and C N H bond angles were derived from ab initio calculations of vinylamine, N-methylvinylamine and aniline. The bending parameters for H C N were set equal to those of H C O. For MM3 they were further modified to best reproduce the experimental data. The amino groups of all the compounds studied in this work were calculated to be pyramidal, as also supported by other theoretical and experimental works44. Selected structural parameters (see Reference 44 for a complete comparison) for the R2CDC NR2 moiety of vinylamine, N-methylvinylamine, 1-aminopropene, aniline, N-methylaniline and N,N-dimethylaniline are gathered in Table 19. The general agreement
TABLE 19. Selected structural parameters of the R2CDC NR2 moiety (bond lengths in A,˚ bond and torsional angles in degrees) for the set of enamines and aniline derivatives used in the parameterization of MM2 and MM3 as obtained by the two force fields, from ab initio calculations and from experiment44 . Reproduced by permission of John Wiley & Sons, Inc. from Ref. 44
|
|
|
MM2 |
MM3 |
ab initio |
Experiment |
|
|
|
|
|
|
|
|
˚ |
|
|
|
|
|
Bond lengths (A) |
|
|
|
|
|
|
CDC |
a |
|
1.340 |
1.341 |
1.343 |
1.335 |
vinylamine |
|
|
||||
N-methylvinylamineb |
1.342 |
1.341 |
1.324 |
|
||
1-aminopropene-transc |
1.341 |
1.342 |
1.341 |
|
||
1-aminopropene-cis |
|
1.342 |
1.344 |
1.344 |
|
|
anilined |
|
|
1.399 |
1.403 |
1.401 |
1.397 |
N-methylanilinee |
|
1.402/1.402 |
1.406/1.405 |
1.397 |
|
|
N,N-dimethylanilinef |
1.408 |
1.409 |
|
1.405 |
||
(CD)C N |
a |
|
1.405 |
1.397 |
1.395 |
1.397 |
vinylamine |
|
|
||||
N-methylvinylamineb |
1.402 |
1.393 |
1.389 |
|
||
1-aminopropene-transc |
1.405 |
1.397 |
1.405 |
|
||
1-aminopropene-cis |
|
1.405 |
1.397 |
1.402 |
|
|
anilined |
|
|
1.406 |
1.398 |
1.415 |
1.402 |
N-methylanilinee |
|
1.450 |
1.396 |
1.44 |
|
|
N,N-dimethylanilinef |
1.406 |
1.399 |
|
1.43 |
||
(CDC )N C |
b |
1.457 |
1.462 |
1.449 |
|
|
N-methylvinylamine |
|
|
||||
N-methylanilinee |
|
1.457 |
1.462 |
1.48 |
|
|
N,N-dimethylanilinef |
1.454 |
1.463 |
|
1.46 |
||
N H |
a |
|
1.020/1.020 |
1.016/1.015 |
1.003/1.006 |
1.010 |
vinylamine |
|
|
||||
N-methylvinylamineb |
1.020 |
1.017 |
0.997 |
|
||
1-aminopropene-transc |
1.020/1.020 |
1.016/1.015 |
1.015/1.016 |
|
||
1-aminopropene-cis |
|
1.020/1.019 |
1.016/1.015 |
1.014/1.015 |
|
|
anilined |
|
|
1.020 |
1.015 |
1.007 |
1.001 |
N-methylanilinee |
|
1.020 |
1.016 |
1.03 |
|
(continued overleaf )

34 |
|
|
Pinchas Aped and Hanoch Senderowitz |
|
||
TABLE 19. |
(continued) |
|
|
|
|
|
|
|
|
|
|
|
|
|
|
|
MM2 |
MM3 |
ab initio |
Experiment |
|
|
|
|
|
|
|
Bond Angles (deg) |
|
|
|
|
|
|
C C N |
a |
|
124.0 |
125.8 |
126.1 |
125.2 |
vinylamine |
|
|
||||
N-methylvinylamineb |
127.7 |
128.1 |
127.7 |
|
||
1-aminopropene-transc |
123.3 |
125.8 |
126.1 |
|
||
1-aminopropene-cis |
|
125.0 |
127.5 |
126.3 |
|
|
anilined |
|
|
120.7 |
120.7 |
120.6 |
|
N-methylanilinee |
|
118.0 |
119.0 |
|
|
|
N,N-dimethylanilinef |
122.8/122.7 |
121.5/121.4 |
|
|
||
C N C |
|
b |
116.6 |
116.9 |
119.5 |
|
N-methylvinylamine |
|
|
||||
N-methylanilinee |
|
117.3 |
119.4 |
|
|
|
N,N-dimethylanilinef |
113.0/115.8 |
111.6/118.0 |
|
116 |
||
Torsional Angles (deg) |
|
|
|
|
||
C C N H a |
|
154.8/23.3 |
154.2/26.5 |
148.9/22.5 |
|
|
vinylamine |
|
|
|
|||
N-methylvinylamineb |
155.4 |
161.8 |
151.0 |
|
||
1-aminopropene-transc |
154.5/ 23.2 |
154.2/ 26.6 |
141.9/ 17.0 |
|
||
1-aminopropene-cis |
|
158.3/27.1 |
157.6/30.8 |
145.0/18.1 |
|
|
anilined |
|
|
155.7/24.0 |
154.3/27.5 |
|
|
N-methylanilinee |
|
23.4 |
21.8 |
|
|
|
C C N C |
|
b |
20.8 |
24.4 |
14.2 |
|
N-methylvinylamine |
|
|
||||
N-methylanilinee |
|
158.5 |
162.5 |
|
|
|
N,N-dimethylanilinef |
21.2/156.5 |
20.0/158.8 |
|
|
aExperiment: MW; ab initio calculations: 5-31GŁ . bAb initio calculations: 4-31G(NŁ ).
cAb initio calculations: MP2/6-31GŁ .
dExperiment: MW; ab initio calculations: 4-31G(NŁ ). eAb initio calculations: STO-3G.
fExperiment: ED.
between molecular mechanics calculations and experimental/ab initio results is good, although a comprehensive comparison is hindered by the paucity of the experimental data and the different levels of ab initio calculations. The dipole moments of vinylamine and N- methylvinylamine were determined from ab initio calculations to be 1.548 D (6-31GŁ)45 and 1.51 D (4-31G(NŁ))46, respectively. Both MM3 and, in particular, MM2 do a good job in reproducing the dipole moment for vinylamine (1.524 D and 1.546 D for MM2 and MM3, respectively) but underestimate that of N-methylvinylamine yielding values of 1.281 (MM2) and 1.258 (MM3) Debye. The experimental dipole moment of aniline (1.53 D)47 is well reproduced by both force fields (MM2: 1.544 D; MM3: 1.542 D).
b. Conformational energies and rotational barriers. The energy difference between the cis and trans conformers of N-methylvinylamine was calculated ab initio (4-31G(NŁ )) to be 1.26 kcal mol 1 in favor of the former46. This number is well reproduced by both MM2 (1.18 kcal mol 1) and MM3 (1.19 kcal mol 1). MM2 also does a good job in reproducing the ab initio (MP2/6-31GŁ) cis trans energy difference in 1-aminopropene of 0.79 kcal mol 1 in favor of the trans conformer48, but the MM3 value is slightly too high (0.76 and 0.97 kcal mol 1 for MM2 and MM3, respectively). The rotational barriers
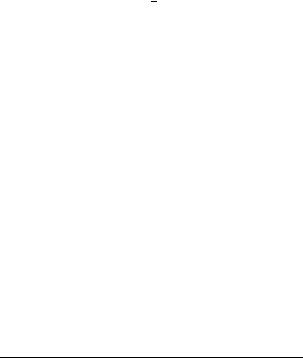
1. Molecular mechanics calculations |
35 |
around the C(sp2) N bond in aniline, N-methylaniline and vinylamine were found to be 3.54 (IR)49, 3.47 (IR)50 and 4.95 (6-31GŁ)45 kcal mol 1, respectively. The corresponding MM2 estimates are 5.27, 5.38 and 4.95 kcal mol 1 and those of MM3, 3.58, 3.91 and 5.01 kcal mol 1, in excellent agreement with the experimental/ab initio data. The torsional barrier around the N CH3 bond in N-methylvinylamine was found to be 3.09 kcal mol 1 (IR)50 and calculated as 3.09 and 3.32 kcal mol 1 by MM2 and MM3, respectively. The experimental (IR) barrier to N-inversion in vinylamine of 1.08 kcal mol 151 is also well reproduced by MM3 (1.03 kcal mol 1). The overall reproduction of the energetic data in these systems by both force fields is good, the MM3 values being in a slightly better agreement with the experimental/ab initio ones.
c. Heats of formation. A comparison of experimental and calculated heats of formation for aniline, N-ethylaniline, N-naphthylamine and 2-naphthylamine is provided in Table 20. Due to the large errors in the experimental values, the accuracy is less than usual with a standard deviation over 4 comparisons of 0.7 kcal mol 1 for both MM2 and MM3.
d. Vibrational spectra. A comparison of experimental and calculated vibrational spectra for vinylamine, N-methylvinylamine and aniline is provided in Reference 44. The RMS error in the calculated frequencies is about 57 cm 1, close to that obtained for unsaturated hydrocarbons. The two main sources of error are N H stretching frequencies which, due to the unaccounted electronegativity effect of the sp2 carbon connected to the nitrogen, are systematically calculated too low by an average of 75 cm 1, and C H bond frequencies which suffer from the lack of 2-center bend bend cross-terms. Both problems could not be solved without major changes to the force field but, fortunately, do not lead to any discernible structural errors44.
5. Other force fields
In this section we briefly outline the explicit form of several general purpose force fields and present the results of calculations on amino and nitro compounds performed as part of their validation.
a. AMBER2. The molecular energy within the AMBER force field framework is given by:
Etotal D Estretch C Ebend C Etorsion C Enonbonded C Ehydrogen bond |
31 |
||||||
TABLE 20. |
Heats of |
formation and standard |
deviation (SD) |
|
|||
(kcal mol 1) |
for enamines and aniline derivatives |
as calculated by |
|
||||
the MM2 and MM3 force fields and observed by experiment44. |
|
||||||
Reproduced by permission of John Wiley & Sons, Inc. from Ref. 44 |
|
||||||
|
|
|
|
|
|
|
|
|
|
|
MM2/MM3 |
Experiment |
|
||
|
|
|
|
|
|
|
|
Aniline |
|
20.50 |
|
20.81 |
|
|
|
N-Ethylaniline |
14.71 |
|
13.5 |
|
|
||
1-Naphthylamine |
37.79 |
|
37.7 |
|
|
||
2-Naphthylamine |
36.11 |
|
35.5 |
|
|
||
SDa |
|
0.70 |
(4) |
|
|
|
aNumber of comparisons is given in parentheses.
36 |
Pinchas Aped and Hanoch Senderowitz |
In the following equations, all parameters have their usual meanings unless otherwise noted.
Estretch i,j D K rij rij0 2 |
(32) |
Ebend i,j,k D K ijk ijk0 2 |
(33) |
Etorsion i,j,k,l D 0.5Vn[1 C cos nωijkl ] |
(34) |
where, in equation 34, is a phase correction; |
|
Enonbonded i,j D Aij/rij12 Bij/rij6 qiqj/εrij |
(35) |
Ehydrogen bond D Cij/rij12 Dij/rij10 |
(36) |
where Aij, Bij, Cij and Dij are adjustable parameters.
No specific parameterization of AMBER for amino, nitro or nitroso compounds has been published. However, several calculations with this force field are found in the literature and are discussed in Section II.D and in Section III of this chapter.
b. Tripos 5.252. The Tripos 5.2 force field52 is implemented in the Sybyl molecular modeling package53. The molecular mechanics energy within the framework of this force field is given by:
Etotal D Estretch C Ebend C Eout-of-plane C Etorsion C Enonbonded |
37 |
In the following equations, all parameters have their usual meanings unless otherwise noted:
Estretch i,j |
D K rij rij0 2 |
(38) |
Ebend i,j,k |
D K ijk ijk0 2 |
(39) |
Eout-of-plane i,j,k,l D Kd2 |
(40) |
|
where d is the distance from atom i to the plane defined by atoms j, k and l; |
|
|
Etorsion i,j,k,l D K[1 C s/jsj cos jsjωijkl ] |
41 |
|
where s is the rotation degeneracy; |
|
|
Enonbonded i,j D K 1.0/aij12 2.0/aij6 |
42 |
where aij is the distance between atoms i and j divided by the sum of their VdW radii, and K is the geometric mean of the VdW constants associated with the two atoms. An electrostatic term is also included in the force field, but was omitted from the calculations described below due to ambiguities in the calculation of atomic charges, the dielectric functions and the cutoffs, and will not be discussed here.
Several calculations on amino and nitro compounds were performed as part of the validation of Tripos 5.252. Thus, the rotational barriers in methylamine and dimethylamine were calculated to be 2.8 and 4.9 kcal mol 1, respectively, in fair agreement with experiment (2.054 and 3.654 kcal mol 1, respectively), and the A-value of the nitro group was calculated to be 0.6 kcal mol 1, only about half of the experimental value

1. Molecular mechanics calculations |
37 |
(1.1 kcal mol 155 ). In addition, several amino and nitro compounds |
were retrieved |
from the Cambridge Structural Database (CSD)56, all having R factors lower than 0.05, and analyzed for their heavy-atom RMS movement upon energy minimization. The results, an average RMS movement of 0.326 A˚ for 19 structures (CSD notation: ABBUMO10, ABZTCX, ACADOS, ACBUOL, ACHTAR10, ACIMDC, ACKYNU, ACMBPN, ACONTN10, ACNPEC, ACRAMS, ACXMPR, ADELOX10, ADENOS10, ADMINA, ADMOPM, ADRTAR, AFCYDP, AFMSCY; see Reference 52), suggest that the X-ray structures are indeed close to minima on the potential energy functions of the isolated molecules as described by this force field. However, the ability of the force field to identify the global minimum or a set of low-energy minima from among the multitude of conformations available to such molecular systems cannot be assessed in this manner. Additional results obtained with the Tripos 5.2 force field are discussed below in Section II.D.
c. DREIDING57. The DREIDING force field is an attempt to address the problem of the arithmetic explosion of parameters required in force field calculations by developing a set of combination rules which would allow for parameters to be developed from a small set of basic atomic properties. Within this framework, the molecular energy is given by:
Etotal D Estretch C Ebend C Etorsion C Einversion C EVdW C Eelectrostatic C Ehydrogen bond 43
In the following equations, all parameters have their usual meanings, unless otherwise noted:
Estretch i,j D 0.5K rij rij0 2 |
(harmonic function) |
44 |
|
or |
|
|
|
Estretch i,j D Defexp[ ˛nrij rij0 ] 1g2 |
(Morse function) |
45 |
|
where, in equation 45, n is the bond order, |
De is |
the dissociation energy |
(n ð |
70 kcal mol 1), K is the force constant (0.5n ð 700 kcal mol 1/A˚ 2) and ˛ is a scaling factor given by (K/2De)0.5;
Ebend i,j,k D [0.5Kijk sin j0 2] cos ijk cos j0 2 (harmonic in cos ) |
46 |
or |
|
Ebend i,j,k D 0.5Kijk ijk j0 2 (harmonic in ) |
47 |
where k D 100 (kcal mol 1)/rad2; |
|
Etorsion i,j,k,l D 0.5Vjk f1 cos[njk ωijkl ωjk0 ]g |
48 |
where njk is the periodicity and ωjk0 is the ‘natural’ torsional angle.
The inversion potential function is applied to all atoms i connected to exactly three other atoms, j, k and l. For nonplanar systems it is given by:
Einversion i,j,k,l D 0.5Ci cos i cos i0 2
where Ci D Ki/ sin i0 2, i is the angle between the i l bond and the j-i-k Ki is the force constant. For planar systems the inversion energy is given by:
Einversion i,j,k,l D Ki 1 cos i
where Ki and i are defined as above.
49
plane and
50

38 |
Pinchas Aped and Hanoch Senderowitz |
The nonbonded interactions are given by a Lennard-Jones 12-6 type expression (LJ) or an exponential-6 form (X6):
E |
VdW LJ |
i,j |
D |
Ar 12 |
|
Br 6 |
|
|
(51) |
||||
|
|
|
ij |
|
ij |
|
|
|
|||||
E |
VdW X6 |
i,j |
D |
A exp |
|
Cr |
ij |
|
|
Br 6 |
(52) |
||
|
|
|
|
|
|
ij |
|
where rij is the distance between the two atoms and A, B and C are obtained from atomic parameters by suitable combination rules.
Electrostatic and hydrogen bond energies are given by:
Eelectrostatic i,j D 322.0637 qiqj/εrij |
(53) |
Ehydrogen bond D, H, A D DHB[5 rHB/rDA 12 6 rHB/rDA 10] cos4 DHA |
(54) |
where, in equation 54, DHA is the (donor) (hydrogen) (acceptor) angle, and rDA is the distance between the donor and acceptor atoms. DHB and rHB were assigned values of 9.5 kcal mol 1 and 2.75 A,˚ respectively, based on results for the water dimer.
In accord with the DREIDING underlying philosophy, no specific parameterization was performed for amino, nitro or nitroso compounds. Rather, a set of atomic parameters were assigned for sp1, sp2 and sp3 nitrogen as well as for nitrogen involved in resonance, and a set of combination rules was developed which allows for the determination of parameters for all combinations of atoms. Several calculations of amino and nitro compounds were performed as part of the validation of DREIDING57. The barrier to inversion of ammonia was calculated to be 7.4 and 8.1 kcal mol 1 when using the harmonic cos and harmonicbending functions, respectively, both in reasonable agreement with the experimental
value (5.8 kcal mol 138 ) but less satisfactory than the MM3 estimate of 5.5 kcal mol 16 . The barriers to rotation in methylamine, dimethylamine and trimethylamine were calculated to be 2.09, 2.92 and 3.53 kcal mol 1, respectively. The first value is in excellent
agreement with experiment (1.98 kcal mol 154 ) but the two latter ones underestimate the corresponding barriers by 0.7 and 0.9 kcal mol 1. The A-value of the nitro group was calculated to be 1.58 kcal mol 1, in reasonable agreement with the experimental value
(1.1 kcal mol 155 ).
As in the case of Tripos 5.252, several amino and nitro compounds were retrieved from the CSD and analyzed for their (all) atom movements upon energy minimization. Although the set of 19 amino and nitro compounds calculated with DREIDING is identical to that examined by Tripos 5.2, a direct comparison between the performance of the two force fields is not possible since the former reported RMS movements of all atoms while the latter, of heavy atoms only. The all atom average RMS movement reported by DREIDING for the set of 19 compounds is 0.298 A˚ and the conclusions we derived for the Tripos force field apply also here. Additional results obtained with the DREIDING force field are discussed in Section II.D.
d. Universal Force Field (UFF)58,59. The Universal Force Field (UFF) may be regarded as a conceptual extension of the DREIDING force field to cover the entire periodic table. Parameters are developed from a small set of atomic properties based only on the element, its hybridization and connectivity while appropriate combination rules are applied to allow for parameter derivation for any combination of atoms. Furthermore, the ‘classic’ bending potential function has been modified to allow for calculation of inorganic molecules and organo-metallic complexes. Within the UFF force field the molecular energy is given by:
Etotal D Estretch C Ebend C Etorsion C Einversion C EVdW C Eelectrostatic |
55 |

1. Molecular mechanics calculations |
39 |
In the following equations, all parameters have their usual meanings, unless otherwise noted:
Estretch i,j D 0.5Kij rij rij0 2 (harmonic function) |
56 |
|
or |
|
|
Estretch i,j D Dijfexp[ ˛ rij rij0 ] 1g2 |
(Morse function) |
57 |
where, in equation 57, Dij is the dissociation energy, |
˛ is a scaling factor |
given by |
Kij/2Dij 0.5 and all other parameters have their usual meaning; |
|
|
Ebend i,j,k D Kijk Cn cos n ijk |
58 |
where the number of terms in this Fourier expansion depends on the molecular geometry;
Etorsion i,j,k,l D Kijkl Cn cos nωijkl |
59 |
where the force constant and the expansion coefficients are determined by the rotational barrier, the periodicity of the potential and the ‘natural’ angle;
Einversion i,j,k,l D Kijkl C0 C C1 cos ωijkl C C2 cos 2ωijkl |
60 |
This expression is used in all cases where atom i is connected to exactly three other
atoms, j, k and l. Here, Kijkl is the force constant, ωijkl is the angle between the i l axis and the i j k plane and the expansion coefficients depend on the central atom type;
EVdW i,j D Dij[ 2 Xij/rij 6 C Xij/rij 12] |
61 |
where Dij is the well depth, Xij is the VdW bond length and rij is the distance between the two atoms;
Eelectrostatic i,j D 332.0637 qiqj/εrij |
62 |
where qi and qj are partial atomic charges, ε is the dielectric constant and rij is the distance between atoms i and j.
As in the case of DREIDING, the underlying philosophy of UFF does not allow for parameters to be developed for specific molecular systems (e.g. amino, nitro or nitroso). Rather, these are extracted from atomic parameters using a set of combination rules. Several calculations on amino and nitro compounds were performed as part of the validation of UFF58,59. In the following we refer to the structural results and save the discussion of the energetic ones to Section II.D.
A comparison of structural data as calculated by UFF with experimental and MM2 results for dimethylamine, trimethylamine, 1,5,9,13-tetraaza-cyclohexadecane (7), azetidine (3) and nitromethane is provided in Table 2159. Given the known inaccuracies inherent to any comparison of experimental and theoretical structural data, UFF appears to predict the general structure of unstrained saturated amines reasonably well (bond lengths and bond angles to within 0.026 A˚ and 2.5° of the experimental values) although it does not reproduce the experimentally observed shortening of C N bonds when going from secondary to tertiary amines. The performance of UFF, however, is considerably reduced when going to the strained azetidine (3) or unsaturated nitromethane where differences of up to 0.076 A˚ in bond lengths (N O bond in nitromethane) and 7.4° in bond angles (C C N bond in azetidine) are observed. In addition, UFF predicts azetidine to be planar, in contrast with the puckered geometry obtained from both experiment and MM2 and MM3 calculations. Clearly, special parameters are needed for an accurate description

40 |
Pinchas Aped and Hanoch Senderowitz |
TABLE 21. Selected structural parameters for dimethylamine, trimethylamine, 1,3,5,9-tetraazacyclohexadecane (7), azetidine (3) and nitromethane as calculated by UFF and MM2 and obtained from experiment58,59
|
UFF |
MM2a |
Experimentb |
Dimethylamine |
|
|
|
C N |
1.464 |
1.461 |
1.464 |
C H |
1.110 |
1.113 |
1.090 |
N H |
1.047 |
1.020 |
1.022 |
C N C |
110.2 |
112.3 |
112.0 |
Trimethylamine |
|
|
|
C N |
1.471 |
1.455 |
1.451 |
C H |
1.112 |
1.114 |
1.109 |
C N C |
110.0 |
110.9 |
110.9 |
1,5,9,13-Tetraazacyclohexadecane |
(7) |
|
|
C N (av.) |
1.470 |
1.462 |
1.457 |
C C (av.) |
1.537 |
1.540 |
1.511 |
N1. . .N5 |
3.24 |
2.96 |
2.92 |
N1. . .N9 |
4.50 |
4.18 |
4.14 |
C C C (av.) |
113.5 |
115.5 |
116.0 |
C C N (av.) |
111.8 |
113.2 |
112.4 |
Azetidine (3) |
|
|
|
C N |
1.463 |
1.471 |
1.482 |
C C |
1.514 |
1.549 |
1.553 |
C H |
1.112 |
1.116 |
1.107 |
N H |
1.048 |
1.014 |
1.022 |
C C C |
84.9 |
86.6 |
86.9 |
C C N |
93.2 |
86.4 |
85.8 |
|
0.1 |
36.2 |
33.1 š 2.4 |
Nitromethane |
|
|
|
C N |
1.455 |
1.503 |
1.489 |
N O |
1.300 |
1.222 |
1.224 |
N C H (av.) |
110.0 |
110.2 |
107.2 |
C N O |
120.2 |
116.5 |
117.3 |
O N O |
119.7 |
127.0 |
125.3 |
aMM2 calculations for 1,3,5,9-tetraazacyclohexadecane (7) and azetidine (3) were taken from Reference 59, which refers to the original Allinger parameterization of amines5. However, to the best of our knowledge this force field did not include the primary electronegativity corrections. Those for dimethylamine and trimethylamine were calculated for this work with MM2-91 and those for nitromethane were taken from Reference 43.
bSee References 58 and 59 for the sources of the experimental data.
of strained and unsaturated systems, as demonstrated by the success of MM2 and MM3 in this field.
D. Energetic Comparison Between MM2, MM3, AMBER, Tripos 5.2, DREIDING and UFF
In this section we provide a short comparison of conformational energies for a set of nitrogen-containing molecules as obtained by several commonly used molecular mechanics force fields and by experiment. The data, collected in Table 22, were mostly taken from Reference 60 but several were calculated for this work.
Any attempt to evaluate the performance of empirical force fields by comparing calculated and experimental conformational energies is influenced by choices of the molecules to be included in the data set and of the source of the experimental data. Clearly, there