
Multiple Bonds Between Metal Atoms / 05-Tungsten Compounds
.pdf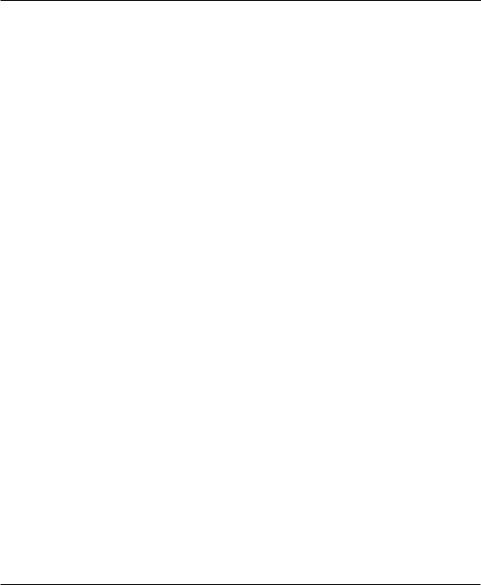
5
Tungsten Compounds
Judith L. Eglin,
Los Alamos National Laboratory
5.1Multiple Bonds in Ditungsten Compounds
In contrast to the ease of preparation of the other group 6 elements Cr and Mo, the syntheses of critical ditungsten starting materials have been notably difficult. Specifically, W24+ tetracarboxylates W2(O2CR)4 and salts of the [W2Cl8]4- anion have not shown the synthetic utility of the Mo24+ analogs. Therefore, progress in the synthesis, structural characterization, and reactivity studies of W24+ compounds has relied on new developments in synthetic methodologies and new ligand types.
The more than fifty structurally characterized quadruply bonded W24+ compounds fall into three primary categories: classic paddlewheel complexes with four bridging anionic ligands both with and without axially coordinated neutral ligands,1-22 compounds coordinated by only anionic ligands,23-26 and compounds coordinated by four anionic ligands and neutral ligands.1,27-42 In Section 5.2, the first attempts to synthesize the tetracarboxylates of the type W2(O2CR)4, culminating in their successful isolation and characterization in the early 1980’s, are described. Subsequent sections focus on the comparatively small but growing number of other W24+ and MoW4+ quadruply bonded complexes and paddlewheel compounds with either W25+ or W26+ cores. The W–W distances of the structurally characterized ditungsten complexes are provided in Table 5.1. A list of other synthesized but not structurally characterized W24+ complexes is provided in Table 5.2.
5.2The W24+ Tetracarboxylates
Following the successful preparation of Mo2(O2CCH3)4 from Mo(CO)6 by Wilkinson and co-workers,43 three reports44-46 appeared between 1969 and 1973 that described the analogous reaction between acetic acid and W(CO)6. In two of these studies,44,45 the thermal reaction between W(CO)6 and acetic acid-acetic anhydride was investigated. The third report46 described attempts to prepare W2(O2CCH3)4 by photolysis of a 1:2 stoichiometric mixture of W(CO)6 and acetic acid in benzene. None of these early investigations yielded the yellow-brown solids of the acetate derivative.17,18,21 The use of other carboxylic acids, either alone or mixed with the corresponding anhydrides, in place of acetic acid produces brown complexes of approximate formula [W(O2CR)2]x, where R = Ph, p-CH3C6H4, C6F5, C3H7 or C3F7.45 Oxidation state titrations on several of the products gave oxidation numbers for tungsten close to +2.0.
183

184Multiple Bonds Between Metal Atoms Chapter 5
Table 5.1. Structurally characterized compounds with a quadruply bonded W24+ core listed by increasing W–W bond length
Compound |
W–W (Å) |
ref. |
|
W2(dmhp)4·0.5(diglyme) |
2.155(2) |
2 |
|
W2(hpp)4·2NaHBEt3 |
2.1608(5) |
3 |
|
W2(mhp)4·CH2Cl2 |
2.161(1) |
4 |
|
W2(hpp)4 |
2.1617(4) |
68 |
|
W2(dmhp)4·(diglyme) |
2.163(1) |
2 |
|
W2(map)4·2THF |
2.164(1) |
5 |
|
W2(ap)4·2/3THF |
2.168(2) |
6 |
|
2.164(2) |
|||
|
|
||
W2(dmhp)2[(PhN)2N]2·2THF |
2.169(1) |
7 |
|
W2(dmhp)2[µ-(PhN)2CCH3]2·2THF |
2.174(1) |
8 |
|
W2[O2CC6H2-2,4,6-(CH3)3]4·2CH3C6H5 |
2.176(1) |
9 |
|
W2(chp)4 |
2.177(1) |
10 |
|
W2(fhp)4·THF |
2.185(2) |
11 |
|
W2(DTolF)4·C7H8 |
2.187(1) |
12 |
|
W2(O2CEt)4 |
2.189(1) |
13 |
|
W2(DCl2PhF)4 |
2.1920(3) |
14 |
|
W2(Dp-ClPhF)4 |
2.1924(2) |
22 |
|
W2(O2C(CH2)2CH3)4 |
2.194(3) |
15 |
|
W2(Dm-MePhF)4 |
2.1957(6) |
22 |
|
W2(O2CC6H5)4·2THF |
2.196(1) |
9,16 |
|
W2[O2CC6H4(4-OCH3)]4·2THF |
2.203(1) |
9 |
|
W2(O2CCF3)4·2/3(diglyme) |
2.211(2) |
17 |
|
2.207(2) |
|||
|
|
||
W2(O2CBut)4·2PPh3 |
2.218(1) |
18 |
|
W2(µ-O2CCF3)2(δ1-O2CCF3)2(PBun3)2 |
2.224(1) |
19 |
|
W2(azin)4·2THF |
2.227(2) |
20 |
|
W2(µ-O2CBut)3(δ1-O2CBut)(PMePh2)2 |
2.2345(9) |
1 |
|
W2(O2CCF3)4·2PPh3 |
2.240(1) |
21 |
|
2.243(1) |
|||
|
|
||
W2Cl4(NH2Cy)4 |
2.2455(5) |
27 |
|
W2(µ-O2CCF3)(O2CCF3)3(PMe3)3 |
2.246(1) |
19 |
|
Na4(TMEDA)4[W2Cl8] |
2.259(1) |
26 |
|
2.254(1) |
|||
|
|
||
W2Cl4(4-But-py)4·4-But-py |
2.259(1) |
28 |
|
W2Cl4(4-But-py)4·C7H8 |
2.2602(8) |
28 |
|
W2Cl4(4-But-py)4·(CH3)2CO |
2.2605(6) |
28 |
|
W2Cl4(PMe3)4 |
2.262(1) |
29,30 |
|
Li4W2(CH3)xCl8-x·4THF |
2.263(2) |
25 |
|
W2Cl4(4-But-py)4 |
2.2631(6) |
28 |
|
`-W2Br4(dppm)2 |
2.2632(1) |
31 |
|
Li4W2(CH3)8·4Et2O |
2.264(1) |
25 |
|
W2Cl4(PBun3)4·C7H8 |
2.267(1) |
32 |
|
W2(CCMe)2Cl2(PMe3)4 |
2.268(1) |
33 |
|
`-W2Cl4(dppm)2 |
2.269(1) |
34 |
|
W2Cl4(PMePh2)4·C6H6 |
2.2728(7) |
35 |

Tungsten Compounds 185
|
|
Eglin |
Compound |
W–W (Å) |
ref. |
|
|
|
_-W2Cl4(dppp)2 |
2.274(2) |
36 |
W2(CCMe)4(PMe3)4 |
2.2742(9) |
37 |
_-W2Cl4(dppe)2·0.5H2O |
2.281(1) |
30,38 |
_-W2Cl4(dmpe)2·(toluene) |
2.287(1) |
29,30 |
[W2{p-But-calix[4](O)4}2(µ-Na)4] |
2.292(1) |
24 |
W2(µ-O2CC6H5)2I2(µ-dppm)2 |
2.2925(6) |
39 |
_-W2Cl4(depe)2 |
2.2950(7) |
40 |
`-W2Cl4(Pri2PCH2CH2CH2PPri2)2 |
2.297(1) |
41 |
W2(µ-O2CC6H5)2Br2(µ-dppa)2·2THF |
2.299(1) |
42 |
`-W2Cl4(dppe)2 |
2.314(1) |
30,38 |
W2(TPP)2 |
2.352(1) |
90 |
W2(C8H8)3 |
2.375(1) |
23 |
Table 5.2. Other compounds with a W24+ core
Compound |
ref. |
Compound |
ref. |
[W(OEP)]2 |
91 |
[W2(O2CBut)3]2(2,5-TH(CO2)2) |
66 |
[W(TOEP)]2 |
92,122 |
W2(O2CBut)2Cl2(PMe3)2 |
1 |
W2(µ-mhp)2(µ-TFA)2 |
17 |
W2(O2CC6H5)2Cl2(µ-dppa)2·2THF |
42 |
W2(TFA)4 |
21 |
W2(O2CC6H5)2I2(µ-dppa)2·2THF |
42 |
W2(TFA)4·2PMe3 |
19 |
W2(O2CC6H5)2Cl2(µ-dppm)2 |
39 |
W2(TFA)4·2PEt3 |
19 |
W2(O2CC6H5)2Br2(µ-dppm)2 |
39 |
W2(O2CC6H5)4 |
45 |
W2Cl4(3-Bun-py)4 |
28 |
W2(O2CC6H4CH3)4 |
45 |
W2Cl4(NH2Prn)4 |
27 |
W2(O2CC6F5)4 |
45 |
W2Cl4(NH2But)4 |
27 |
W2(O2CC3F7)4 |
45 |
Na4(THF)x[W2Cl8] |
87 |
W2(O2CCH3)4 |
13,18 |
Na4(DME)4[W2Cl8] |
87 |
W2(O2CBut)4 |
1,13, |
W2Cl4(PMePh2)4 |
86 |
|
18,87 |
W2Cl4(PMe2Ph)4 |
31 |
W2(O2CBut)4·2PMe2Ph |
1 |
W2Cl4(PBun3)4 |
86 |
W2(O2C(CH2)6CH3)4 |
15 |
W2Cl4(PEt3)4 |
97 |
W2(O2C(CF2)6CF3)4 |
15 |
W2Cl4(PPrn3)4 |
150 |
W2[O2CC6H4(4-CN)]4·2THF |
9 |
W2Cl4(PEt3)3(PMe3) |
97 |
W2(µ-O2CCCo3(CO)3)3(µ-O2CCF3)·2THF |
148 |
W2Cl4(PEt3)2(PMe3)2 |
97 |
W2(µ-O2CCCo3(CO)3)4 |
149 |
W2Cl4(PEt3)3(PMe2Ph) |
97 |
W2(O2CBut)4]2·2ButCONMe2 |
13 |
W2Cl4(PEt3)2(PMe2Ph)2 |
97 |
W2(O2CMe)4·2MeCONMe2 |
13 |
W2Cl4(PEt3)3(PMePh2) |
97 |
W2(O2CEt)4·2EtCONMe2 |
13 |
W2Cl4(PBun3)3(PMe3) |
97 |
W2(2-THCO2)4 |
66 |
W2Cl4(PBun3)3(PMe2Ph) |
97 |
W2(3-THCO2)4 |
66 |
W2Cl4(PBun3)2(PMe2Ph)2 |
97 |
W2(µ-O2CBut)(O2CBut)3(PMe3)2 |
1 |
W2Cl4(PBun3)3(PMePh2) |
97 |
W2(µ-O2CBut)(O2CBut)3(PMe2Ph)2 |
1 |
W2Br4(PMe2Ph)4 |
31 |
W2(µ-O2CBut)2(O2CBut)2(PMe3)2 |
1 |
W2Br4(PMePh2)4 |
31 |
[W2(O2CBut)3]2(O2CCO2) |
65 |
W2(CCBut)4(PMe3)4 |
99 |
[W2(O2CBut)3]2(O2C-1,4-C6F4-CO2) |
65 |
`-W2Cl4(dppa)2·2THF |
42 |
[W2(O2CBut)3]2(O2C-1,8-C14H8-CO2) |
65 |
_-W2Cl4(dmpe)2 |
86 |
[W2(O2CBut)3]2(O2C-1,4-C14H10-CO2) |
65 |
_-W2Cl4(dppe)2 |
86 |
[W2(O2CBut)3]2(O2C(C5H4)Fe(C5H4)CO2) |
65 |
|
|

186Multiple Bonds Between Metal Atoms Chapter 5
Unfortunately, none of these products afforded single crystals suitable for a crystal structure determination, and spectroscopic characterizations failed to confirm their identity as W2(O2CR)4, so their relationship to now characterized acetate products remains unclear. In many of these reactions, a large proportion of the tungsten species remained in solution. Workup of the reaction filtrates showed that the main products were trinuclear clusters having a [W3O2(O2CR)6]n+ core.47,48
Following these early unsuccessful attempts to prepare an authentic tetracarboxylate with a W24+ core, the first structural characterization was reported for W2(O2CCF3)4 ·2/3(diglyme).17 This compound was synthesized by Sattelberger et. al. by reduction at -20 °C of W2Cl6(THF)4 (later reformulated as NaW2Cl7(THF)5)49 with 2 equiv of sodium amalgam followed by subsequent addition of Na(O2CCF3).17 While this method failed to provide a direct route to W2(O2CCH3)4,18 this compound can be prepared by the metathesis of W2(O2CCF3)4 with (Bu4N)O2CCH3 in toluene.18 With the synthesis of W2(O2CCF3)4, a route to ditungsten tetracarboxylates was established and provided the experimental foundation for the synthesis of a variety of W2(O2CCR3)4 compounds.17,18,21,50
An alternative synthetic procedure involves reduction of a mixture of WCl4 and sodium trifluoroacetate with Na/Hg in THF at 0 °C.21 The pivalate analog is prepared by this method,18 and the reaction also provides a convenient route to the corresponding tetraarylcarboxylate derivatives W2(O2CAr)4 (Ar = Ph, C6H4-p-OMe, and C6H2-2,4,6-Me3).9,16 Based on the synthesis of other W24+ materials such as W2Cl4(PMePh2)4,31 NaBEt3H has also been used as reducing agent as in the synthesis of W2(O2CPh)4 from WCl4 and NaO2CPh.39 Another useful method for the synthesis of alkyl carboxylate analogs involves room temperature reaction of hydrocarbon solutions of 1,2-W2Et2(NMe2)4 with acid anhydrides (RCO)2O, where R = CH3, C2H5, or CMe3 with product yields in the range of 40 to 65%, after recrystallization. The general reaction is shown in the following equation:13
1,2-W2Et2(NMe2)4 + 4(RCO2)2O Α W2(O2CR)4 + 4RCONMe2 + C2H4 + C2H6
The crystal structures of several tetraalkyl and tetraaryl carboxylate derivatives have been determined, both with and without axially coordinated ether molecules such as THF or diglyme. In W2(O2CC2H5)4, weak intermolecular W–O axial interactions (2.665(4) Å) link the dinuclear units into infinite chains.13 The bis-toluene solvate of W2(O2CC6H2-2,4,6-Me3)4 is the only W24+ tetracarboxylate known to lack axial interactions.9 A summary of the W–W bond lengths for the structurally characterized derivatives is provided in Table 5.1 and the structure of W2(O2CC6H5)4.2THF is shown in Fig. 5.1.9 Note that the shorter W–W bond length in W2(O2CC6H2-2,4,6-Me3)4 can possibly be ascribed to the absence of axial ligands, a structural effect that is seen in other tetracarboxylate M24+ complexes of the group 6 elements.
The air-sensitive alkyl tetracarboxylate complexes can be sublimed,18,21 and give an intense parent ion multiplet in the mass spectra which provides conclusive evidence that these dinuclear complexes can survive intact in the vapor phase. This has allowed measurement of the UV photoelectron spectra of W2(O2CCF3)451 and W2(O2CCH3)452 in the gas phase. The spectrum for gaseous W2(O2CCH3)4 is very similar to that in a thin film.52 The β ionizations of Mo2(O2CCF3)4 and W2(O2CCF3)4 appear at 8.76 and 7.39 eV, respectively,51 a difference that correlates with the much greater susceptibility of W24+ complexes to oxidation and oxidative-addition reactions. This trend is also reflected in the electrochemical properties of W2(O2CR)4 (R = CH3 or CMe3).18 The E1/2(ox) values measured for acetonitrile solutions of these complexes (-0.37 V and -0.40 V, respectively, versus SCE) are c. 0.8 V more negative than for the Mo24+ analogs.18 The pivalate complex is easily oxidized to the paramagnetic EPR-active salt [W2(O2CCMe3)4]I upon treatment with I2 in benzene,18 a reaction that is similar to the oxidations of Mo2(O2CCMe3)4
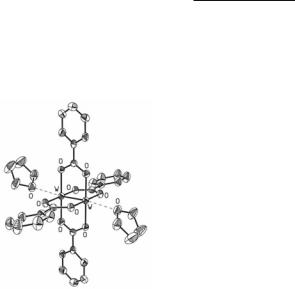
Tungsten Compounds 187
Eglin
and MoW(O2CCMe3)4 (Section 5.5). Other important spectroscopic characterizations carried out on these W24+ tetracarboxylates include the 183W NMR spectra of the trifluoroacetate21 and pivalate,18 and the assignment of the βΑβ* transition for several of the alkyl18 and aryl9 tetracarboxylate derivatives.
Fig. 5.1. The structure of W2(O2CC6H5)4·2THF. The W–O(THF) separations are c. 2.6 Å.
The ability of W2(O2CR)4 compounds to form axial adducts has already been discussed with reference to the ether ligands THF and diglyme (Table 5.1). In addition, triphenylphosphine reacts with W2(O2CR)4 (R = CF3 or CMe3)18,21 to form W2(O2CR)4(PPh3)2. Both complexes have been structurally characterized and have axially bound triphenylphosphine molecules.18,21 The weakening of the W–W bond of W2(O2CR)4 by axial W–L interactions is reflected by changes in the Raman active ι(W–W) modes. This is illustrated by the shift in ι(W–W) from 310 cm-1 in W2(O2CCF3)4 to 280 cm-1 in W2(O2CCF3)4(PPh3)2,21 and from 313 cm-1 in W2(O2CCMe3)4 to 287 cm-1 in W2(O2CCMe3)4(PPh3)2.18
In contrast to reactions of PPh3 with W2(O2CCF3)4 and W2(O2CCMe3)4 leading to 1:2 adducts that contain axially bound phosphine ligands,18,21 the behavior of W2(O2CCF3)4 with other phosphine ligands (PMe3, PEt3, and PBun3) is more complex.19 Toluene solutions of W2(O2CCF3)4 react with these three trialkylphosphine ligands to yield red to red-orange, airsensitive 1:2 adducts. The 19F and 31P{1H} NMR spectra (+25 to -50 °C) support the presence of a single isomer with the phosphine ligands bound equatorially and two bridging bidentate and two monodenate trifluoroacetate ligands.19 A single crystal X-ray structure determination for W2(µ-O2CCF3)2(δ1-O2CCF3)2(PBun3)2 confirms19 the presence of a single isomer in the solid state. Similar to the dimolybdenum analog,53-55 W2(O2CCF3)4 can react with phosphine ligands to give axial (D4h symmetry) or equatorial (C2h symmetry) adducts. An unusual isomer is W2(O2CBut)3(δ1-O2CBut)(PMePh2)2 with one axially and one equatorially coordinated phosphine ligand and an equatorially coordinated carboxylate ligand.1
Reactions of W2(O2CCF3)4 or W2(µ-O2CCF3)2(δ1-O2CCF3)2(PMe3)2 with an excess of PMe3 yields the corresponding dark-green 1:3 adduct.19 W2(µ-O2CCF3)(δ1-O2CCF3)3(PMe3)3 is stable in solution at room temperature but loses a molecule of PMe3 to form W2(µ-O2CCF3)2(δ1- O2CCF3)2(PMe3)2 when heated in benzene.19 The 19F and 31P{1H} NMR spectra indicate that W2(µ-O2CCF3)2(δ1-O2CCF3)2(PMe3)2 has the same structure in solution (with equatorially bound phosphine ligands) as that found in the solid state by X-ray crystallography. A comparison of the spectroscopic properties of W2(µ-O2CCF3)(δ1-O2CCF3)3(PMe3)3 and the previously reported molybdenum analog Mo2(µ-O2CCF3)(δ1-O2CCF3)3(PMe3)356 suggests that these complexes are isostructural. However, the differences that exist in solution between

188Multiple Bonds Between Metal Atoms Chapter 5
Mo2(O2CCF3)4(PR3)2 and W2(O2CCF3)4(PR3)2, and between Mo2(µ-O2CCF3)(δ1-O2CCF3)3(PMe3)3 and W2(µ-O2CCF3)(δ1-O2CCF3)3(PMe3)3, have been linked to differences in the M–P bond
strengths (W–P > Mo–P).19
Unlike the Mo24+ tetracarboxylates that have served as key starting materials in the development of multiply bonded dimolybdenum chemistry, the related W24+ compounds have more limited use as synthetic precursors. The ease of oxidation of W2(O2CR)4 is a hindrance in designing synthetic procedures for preparation and subsequent reactivity studies of the tetracarboxylates.57 However, W2(O2CPh)4 is a synthetic precursor that is easily prepared by reduction of WCl4 with NaBEt3H followed by addition of NaO2CPh.39 Unlike the reaction of Mo2(O2CCF3)4 with Me3SiX where X is Cl, Br, or I and bidentate phosphine ligands such as dppm to produce Mo2X4(µ-dppm)2 compounds, only a maximum of three of the benzoate ligands can be replaced upon oxidative addition of HBr to the W24+ core.58-61 For the reaction of W2(O2CPh)4 with dppm and Me3SiBr, the presence of acid from the halide source in the reaction mixture promotes the loss of a third carboxylate ligand and the formation of the W26+ complex with a bridging hydride W2(µ-H)(µ-O2CPh)Br4(µ-dppm)2·2THF.39 By using halide sources such as Me3SiI or the zinc salts ZnCl2, ZnBr2 or ZnI2 to eliminate acid, the compounds W2(µ-O2CPh)2X2(µ-dppm)2 were made (X is Cl, Br, or I) and these still retained two of the benzoate ligands.39 The structure of W2(µ-O2CPh)2X2(µ-dppm)2 is shown in Fig. 5.2.
Fig. 5.2. The structure of W2(O2CPh)2(dppm)2I2.
The presence of an acid may be required to protonate the third and fourth carboxylate groups from the ditungsten core as in the dimolybdenum analogs where acids drive the reactions to completion.29,62,63 The formation of oxidative addition products does not occur in Mo42+ chemistry as demonstrated by the preparation of K4Mo2Cl8 in a highly acidic reaction medium.64
Dinuclear compounds are of interest in the synthesis of oligomers, and the tetracarboxylate W2(O2CBut)4 has been used as a precursor in the synthesis of two W24+ cores linked by dicarboxylates with either a perpendicular or parallel alignment of the W–W bonds.65 Using a simple substitution reaction, five new precursors to oligomeric materials have been synthesized,
namely [W2(O2CBut)3]2(O2CCO2), [W2(O2CBut)3]2(O2C-1,4-C6F4-CO2), [W2(O2CBut)3]2(O2C- 1,8-C14H8-CO2), [W2(O2CBut)3]2(O2C-1,4-C14H10-CO2), and [W2(O2CBut)3]2(O2C(C5H4)Fe- (C5H4)CO2).65 The work has been expanded to include the thienylcarboxylates in order to further
understand the electronic properties of the parent paddlewheel compounds W2(2-THCO2)466 and W2(3-THCO2)466 in addition to the tetranuclear species [W2(O2CBut)3]2(2,5-TH(CO2)2)66.

Tungsten Compounds 189
Eglin
5.3W24+ Complexes Containing Anionic Bridging Ligands Other Than Carboxylate
An organometallic compound with a W–W quadruple bond is the cyclo-octatetraene derivative W2(COT)3 prepared by reaction of WCl4 and K2C8H8 in tetrahydrofuran.67 One of the COT ligands is a bridging dianion while the others are monoanions, with one COT- bound to each of the W atoms. W2(COT)3 is isostructural with the molybdenum analog.23,67
The nitrogen-containing monoanionic ligands hpp,3,68 mhp,4 chp,10 fhp,11 dmhp,2 map,5 DTolF,12 DCl2PhF,14 Dp-ClPhF,22 Dm-MePhF,22 azin,20 and ap6 form W24+ paddlewheel compounds with W–W bond lengths similar to those of the tetracarboxylates (Table 5.1). The dark-red purple W2(mhp)4 complex was the first one of this series to be reported. It forms upon refluxing W(CO)6 (not WCl4) with 2-hydroxy-6-methylpyridine (Hmhp) in diglyme.4 W2(mhp)4 is isostructural with the Cr and Mo analogs.4 Both W2(mhp)4 and MoW(mhp)4 display readily accessible one-electron oxidations.69 The E1/2 values (from cyclic voltammetry) for these complexes in acetonitrile solutions are -0.35 V and -0.16 V, respectively, versus SCE.69
Some of these anionic ligands allow the syntheses of homologous series of Cr, Mo, and W compounds as in the case of the anions of 2,4-dimethyl-6-hydroxypyrimidine (Hdmhp),2 2-hydroxy-6-chloropyridine (Hchp),10 1,3,4,6,7,8-hexahydro-2H-pyrimido[1,2-a]pyrimidine (Hhpp),3,68,70 2-amino-6-methylpyridine (Hmap),5 and 2-hydroxy-6-fluoropyridine (Hfhp).11 The reactions of the first two of these ligands with W(CO)6 produce W2(dmhp)4 and W2(chp)4.2,10 Interestingly, this synthetic strategy does not always work in the synthesis of W24+ complexes as shown by the reaction of W(CO)6 with N,N'-di-3,5-xylylformamidine. This reaction yields W2(µ-CO)2[µ-HC(NR)2]2[HC(NR)2[(RN)CH(NR)CH2], where R = 3,5-xylyl, a complex that probably contains a W–W double bond (the W–W length is 2.464(3) Å).71,72 In contrast, Cr(CO)6 and Mo(CO)6 react with this formamidine to yield the expected M24+ quadruply-bonded complexes.71 The tungsten complex coordinated by dmhp can be obtained as two diglyme solvates, W2(dmhp)4·1/2diglyme or W2(dmhp)4·diglyme.2 As shown in Table 5.1, the W–W bond lengths of these two forms differ very little.2
The complex derived from 2-hydroxy-6-fluoropyridine is prepared as the 1:1 adduct with THF by Na/Hg reduction of a THF solution containing Na(fhp) and WCl4.11 As the Cr and Mo analogs, W2(fhp)4 has a polar structure with four bridging fhp ligands orientated in the same direction; a THF molecule is axially coordinated to the metal atom bonded to four oxygen atoms.11 A synthetic procedure similar to that used to prepare W2(fhp)4 has been adapted for the synthesis of W2(ap)4 (ap is 2-anilinopyridine), a complex that contains an eclipsed [W2N8] core.6 Cyclic voltammetric measurements on solutions of W2(ap)4 in THF indicate the presence of a very accessible oxidation at E1/2 = -0.067 V versus Ag/AgCl and a one-electron reduction at -0.84 V.6
For hpp,3,68 DTolF,12 DCl2PhF,14 Dp-ClPhF),22 and Dm-MePhF,22 the W24+ paddlewheel compounds are synthesized by low temperature reduction of WCl4 with either Na/Hg or NaBEt3H followed by addition of the appropriate deprotonated ligand. The core structure of W2(Dm-MePhF)4 is shown in Fig. 5.3.22 For W2(hpp)4,3,68 the use of the reducing agent NaBEt3H results in interstitial NaBEt3H, and the structure of W2(hpp)4.2NaBEt3H (2.1608(5) Å)3 was determined. Reaction of W2(hpp)4Cl2 in refluxing THF with potassium metal provides a synthetic pathway to W2(hpp)4 (2.1617(4) Å).68 The room temperature reaction of four equivalents of Hhpp with the triply bonded compounds 1,2-W2Bui2(NMe2)4 or 1,2-W2(p-tolyl)2(NMe2)4 in benzene results in the generation of isobutene and isobutylene, respectively and formation of W2(hpp)4.73 This is a very strong reducing agent.68 Remarkably in the gas-phase, the onset of the ionization of W2(hpp)4 (3.51 eV) is nearly 0.4 eV lower in energy than Cs.74
The compounds W2(azin)420 and W2(map)45 are made by substitution reactions. The former, W2(azin)4,20 results in 75% yield from the reaction of W2(O2CPh)4 with four equivalents
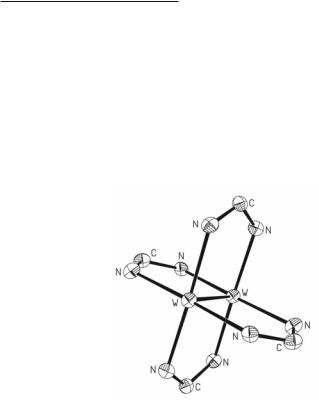
190Multiple Bonds Between Metal Atoms Chapter 5
of Li(azin) in hexanes. The reaction of W2(mhp)4 with the lithium salt of 2-amino-6-meth- ylpyridine (Hmap) leads to displacement of the mhp ligands and formation of W2(map)4.5 The solvates M2(map)4·2THF (M = Cr, Mo, or W) are isomorphous,5 and the W–W bond length is very similar to that in W2(mhp)4 and other complexes of this type. Other examples of ligand displacement reactions are encountered for W2(dmhp)4. Two of the dmhp ligands can be replaced by reacting W2(dmhp)4 with the lithium salts of N,N'-diphenylacetamidine and 1,3-diphenyltriazine, Li[(PhN)2CCH3] and Li[(PhN)2N] respectively, in THF.7,8 The thermally stable but air-sensitive complexes W2(dmhp)2[(PhN)2CCH3]2·2THF and W2(µ-dmhp)2[(PhN)2N]2.2THF contain a transoid arrangement of bridging ligands.
Fig. 5.3. The core of W2(Dm-MePhF)4.
Similar to the tetracarboxylates, W24+ complexes with nitrogen-containing, anionic bridging ligands are easily oxidized. Reactions of W2(mhp)4 with gaseous HCl or HBr in methanol yield [W2X9]3-, rather than [W2X8]4-.2,75 A similar reaction of HCl(g) with W2(mhp)4 or W2(dmhp)4 in methanol or ethanol in the presence of PEt3 or PPrn3 affords a route to the doubly bonded W26+ complexes W2(µ-OR)2Cl4(OR)2(ROH)2 (R = CH3 or C2H5).75,76 These compounds contain a μ2/2 ground state electronic configuration and have been the subject of detailed structural, spectroscopic, and theoretical studies76,77 as well as studies of their chemical reactivity.78-80 The W26+ complexes W2(µ-H)(µ-Cl)Cl4(µ-dppm)2 and W2(µ-H)(µ-Cl)Cl4(py)4 have been prepared57,81 by reactions of W2(mhp)4 with Me3SiCl and dppm or pyridine in methanol. The dppm complex has been structurally characterized,57 as has the 4-ethylpyridine adduct synthesized from W2(µ-H)(µ-Cl)Cl4(py)4 by ligand exchange at 100 ˚C.81 The conversion of W2(mhp)4 to the W25+ complex W2(mhp)3Cl2 and subsequent structural characterization of the dichloromethane solvate has shown that a very short W–W bond is retained (2.214(2) Å).82 Other oxidative addition reactions include the addition of chloroalkanes to W2(hpp)4 to yield W2(hpp)4Cl2.74
When the tetraformamidinate complex W2(DCl2PhF)4 is dissolved in a toluene/hexanes solution and exposed to moisture and oxygen, oxidative addition occurs to result in W2(µ-OH)2(µ-DCl2PhF)2(δ2-DCl2PhF)2.14 This edge sharing bioctahedral W26+ compound is shown in Fig. 5.4. It has a rather short W–W bond length of 2.3508(3) Å, indicating a strong μ2/2β2 interaction.
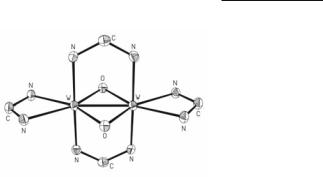
Tungsten Compounds 191
Eglin
Fig. 5.4. The core of W (µ-OH)2(µ-DCl2PhF) (δ2-DCl2PhF)2. |
|
2 |
2 |
5.4W24+ Complexes without Bridging Ligands
5.4.1 Compounds coordinated by only anionic ligands
The first species prepared and unambiguously shown to possess W–W quadruple bonds were salts of the anion [W2(CH3)8]4- and the partially chlorinated ana1ogs.25,83,84 Reaction of either WCl4 or WCl5 with methyllithium at temperatures below 0 °C leads to the red anion [W2(CH3)8]4- when a 1-2 molar excess of LiCH3 is used.84 Li4W2(CH3)8 can be crystallized as either the diethyl ether or tetrahydrofuran solvate Li4W2(CH3)8·4L. With only about a 0.5 molar excess of LiCH3, reduction to WII is accomplished but there is insufficient LiCH3 remaining in solution to displace all of the Cl ligands by CH3 and accordingly the mixed methyl-chloro species Li4W2(CH3)8-xClx·4L (L = Et2O or THF) are formed.83,84 For the latter, different reaction conditions yield different CH3:Cl ratios (2.7-4.6) but with no obvious preference for any particular stoichiometry. These methyl compounds are extremely sensitive to air and moisture and are thermally unstable except at low temperatures.84 Crystal structure determinations on Li4W2(CH3)8·4Et2O and Li4W2(CH3) 8-xClx·4THF confirmed the existence of an eclipsed W2L8 geometry of idealized D4h symmetry. and short W–W bond lengths.25,83
These [W2(CH3)8]4- species are historically very important not only because these complete the first triad of homologous compounds containing metal-metal multiple bonds but more importantly because the existence of [W2(CH3)8]4- suggested that compounds containing the [W2Cl8]4- anion should be isolable. This conclusion was supported by SCF-X_-SW calculations on [W2Cl8]4- which predicted an electronic structure similar to that for [Mo2Cl8]4-.85
While several phosphine-containing derivatives of [W2Cl8]4- were prepared first,86 the development of a successful synthetic route to salts of [W2Cl8]4- was reported in 1982.26 The Na/Hg reduction of WCl4 in THF proceeds first to a green W26+ complex,49,86,87 and then to an intensely blue colored species. Work-up of this solution at 0 °C has afforded Na4(THF)xW2Cl8 as a reactive blue powder.26,87 Attempts to increase the yield of [W2Cl8]4- by performing a reduction of WCl4 with Na/Hg in the presence of additional chloride ions were unsuccessful.87 In one such instance, [(Ph3P)2N]Cl was used as the chloride source but this resulted in dark violet crystals of [(Ph3P)2N]2W2Cl9.88,89
The THF molecules in Na4(THF)xW2Cl8(x = 1-2) can be replaced by bidentate ethers and amines such as dimethoxyethane (DME) and tetramethylethylenediamine (TMEDA).26,87 The salts, Na4(DME)4W2Cl8 and Na4(TMEDA)4W2Cl8, display a band at ~600 nm in their electronic absorption spectra that can be assigned to the βΑβ* transition of the [W2Cl8]4-anion.26,87 A crystal structure of Na4(TMEDA)4W2Cl8 has confirmed the existence of the [W2Cl8]4- anion in this salt.26 The W–W bond lengths of 2.259(1) and 2.254(1) Å for the two independent
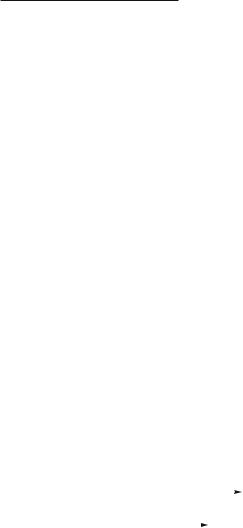
192Multiple Bonds Between Metal Atoms Chapter 5
[W2Cl8]4- ions in the unit cell are about 0.11-0.13 Å longer than the Mo–Mo lengths in salts of [Mo2Cl8]4-. This M–M bond lengthening is typical of that found between analogous quadruply bonded W–W and Mo–Mo species.
Samples of Na4(THF)xW2Cl8 react with phosphine ligands (PMe3 and PBun3) to form W2Cl4(PR3)4 in essentially quantitative yield.87 The [W2Cl8]4- ion also reacts87 with 6-methyl- 2-hydroxypyridine (Hmhp) in the presence of Et3N to yield the known quadruply bonded W24+ complex W2(mhp)4 (Section 5.3). A similar reaction with a THF solution containing pivalic acid and Et3N at -30 °C has been used to obtain W2(O2CCMe3)4 as a yellow powder.87
Another nitrogen based ligand, tetraphenylporphyrin (TPPH2) reacts with W(CO)6 in refluxing decalin for 24 h to yield the black product W2(TPP)2 in 90% yield.90 This compound has a long W–W bond length of 2.352(1) Å,90 and a barrier to rotation of the porphyrin ligand of 11.3 kcal mol-1, as estimated from NMR line shape analysis. The rotational barrier has been taken as a measure of the β bond strength, but it should be noted that steric interactions between the porphyrin ligands and non-bonding electronic interactions are reflected in the rotational barrier. Other porphyrin complexes include derivatives of 2,3,7,8,12,13,17,18octaethylporphyrin (OEP)91 and meso-(4'-tolyl)octaethylporphyrin) (TOEP),92 e. g., W2(OEP)2 and W2(TOEP)2, respectively. With a rotational barrier of 12.9 kcal mol-1 for W2(TOEP)2, the strength of the ditungsten β bond strength appears greater than the dimolybdenum β bond strength in isostructural metalloporphyrin compounds.92
In addition to these known compounds, a recent article predicts the presence of a tungstentungsten quadruple bond in complexes of the hypothetical phase Ca2W6O8.93 Based on Hückel analysis of the bonding, metallic behavior is expected.
5.4.2 Compounds coordinated by four anionic ligands and four neutral ligands
While W2Cl4(PR3)4 complexes have been prepared by the reaction of PR3 with Na4(THF)xW2Cl8, the most convenient preparative route involves reduction of a mixture of WCl4 and the monodentate phosphine ligand in THF with Na/Hg or NaBEt3H as shown
below.35,36,42,86,87
|
|
2WCl |
4 |
+ 4Na/Hg + 4PR |
3 |
THF |
W |
Cl (PR |
) |
4 |
+ 4NaCl |
|
|
|||||
|
|
|
|
|
||||||||||||||
|
|
|
|
|
|
2 |
|
4 |
3 |
|
|
|
|
|
||||
2WCl |
4 |
+ 4NaBEt H + 4PR |
THF |
W Cl (PR |
) |
4 |
+ 4NaCl + 2H |
2 |
+ 4BEt |
3 |
||||||||
|
|
|
3 |
3 |
|
2 |
4 |
3 |
|
|
|
|
|
|
(PR3 = PMe3, PMe2Ph, PMePh2 or PBun3)
If only one equivalent of Na/Hg or NaBEt3H is used in the preceding reaction, red crystalline, edge-sharing bioctahedral complexes, W2Cl6(PR3)4, are obtained.86,94 Upon treatment with a further equivalent of Na/Hg, W2Cl6(PR3)4 is converted to W2Cl4(PR3)4 for PR3 = PMe3 and PMe2Ph.86,94 When the quantity of the phosphine is limited to 1.5 equiv, then the facesharing bioctahedral complexes such as W2Cl6(PMe2Ph)3 and W2Cl6(PBun3)3 are formed.94 The first complexes of the type W2Br6(PMe2Ph)3 and W2Br6(PMe3)3 were synthesized by replacing WCl4 with WBr5 and adjusting the amounts of the reducing agents Na/Hg or NaBEt3H to compensate for the change in oxidation state.94 Reduction of WBr5 with 3 equiv of NaBEt3H and subsequent addition of PMe2Ph or PMePh2 resulted in the first synthesis of compounds with a W2Br4 core and formation of W2Br4(PMe2Ph)4 and W2Br4(PMePh2)4.31
Based upon 31P{1H} NMR data,87 the Raman spectrum of W2Cl4(PBun3)4 (ι(W–W) at 260±10 cm-1),86 detailed electronic absorption29,95 and photoelectron96 studies, and crystal structure determinations of W2Cl4(PMe3)429,30 and W2Cl4(PBun3)4,32 these ditungsten complexes are isostructural with their Mo24+ analogs. Using 31P{1H} NMR spectroscopy to monitor the