
Multiple Bonds Between Metal Atoms / 08-Rhenium Compounds
.pdf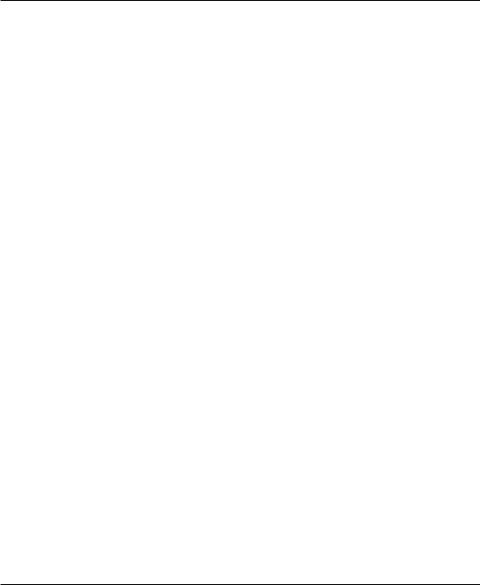
8
Rhenium Compounds
Richard A. Walton,
Purdue University
8.1The Last Naturally Occurring Element to Be Discovered
One reason so much of the chemistry of rhenium was not developed until the last few decades is that the element itself was not discovered until 1925. In its relationship to its group 7 congeners, rhenium is rather special. In all other groups of transition elements, the 4d element is about as common as the 5d element and in several cases it served as a guide to the properties that might be helpful in isolating the heaviest member of the group. This was the case with hafnium, for example, which was identified only two years earlier than rhenium. The 4d element in group 7 is, of course, technetium and it is not found in Nature. Indeed in its initial isolation from the products of deuteron bombardment of molybdenum a number of years later (1939) the standard scenario was reversed. Prior knowledge of the chemistry of rhenium helped in designing procedures to separate technetium. Of course, the 3d element can provide some guidance as to the chemistry of its heavier congeners, but it always differs far more from the heavier two than they do from each other.
Beginning in about 1922 Dr. Walter Noddack and Dr. Ida Tacke (who in 1926 became Mrs. Noddack), see Fig. 8.1, who were employed in the Physico-Technical Testing Office in Berlin began to search for both element 43 (technetium) and element 75 (rhenium) in a number of ores that were known to contain the elements with similar atomic numbers. It was already recognized in those days that elements of odd atomic number (Z) were systematically less abundant than those of even Z and Noddack and Tacke were able to make an approximate forecast of the extent to which they might have to concentrate various ores before either of the missing elements would become detectable. They counted on similarities to manganese chemistry in designing their concentration procedures and expected to use X-ray spectra to detect the new elements.
After several years of work, an approximately 100,000-fold concentration of the group 7 elements in a sample of the mineral gadolinite was accomplished. With Dr. Otto Berg of the Siemens Company, an X-ray spectroscopic analysis revealed that element 75 was present. Using the mineral columbite a ponderable quantity of the element was isolated as the oxide, Re2O7, and it was named rhenium after the river Rhine. The X-ray spectra were also thought to have lines for element 43, but later work leaves no doubt that this was an error. By 1928-1929 gram quantities of rhenium had been isolated and detailed study of the chemistry was begun.1
271
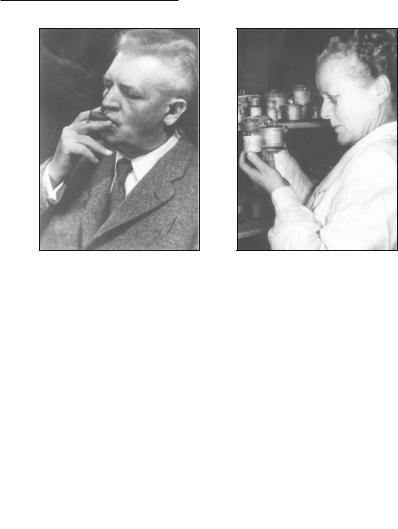
272Multiple Bonds Between Metal Atoms Chapter 8
Fig. 8.1. Walter and Ida (Tacke) Noddack, the discoverers of the element rhenium. These photographs were kindly provided by Professor Otto Glemser.
It is remarkable that rhenium chemistry has proved to be the vehicle for the discovery of the first examples of all the multiple bonds, double, triple, and quadruple, between transition metal atoms. Rhenium trichloride and bromide were discovered2,3 in 1933 and a short time later the complexes of empirical formula RbReCl4 and CsReCl4 were reported.4 However, these compounds were not correctly formulated as Re3 clusters with Re–Re double bonds until 1963. As late as 1962 in an authoritative review5 of “Recent Developments in the Chemistry of Rhenium and Technetium” it was stated that:
The well-known diamagnetic four-coordinate complexes of ReIII include the [ReCl4]- ion. The simple ReIII chloride and bromide are presumably dimeric with halogen bridges, viz.
The correct formulation6 of these compounds, with their double bonds, was soon followed by the recognition7 of the quadruple bond in [Re2Cl8]2- and the first triple bond8 in Re2Cl5(CH3SCH2CH2SCH3)2. The events leading to about this point are reviewed in Chapter 1. Our purpose now is to present systematically the chemistry of dirhenium compounds with Re–Re multiple bonds. The literature up to the end of 1991 was covered comprehensively in the two previous editions of Multiple Bonds Between Metal Atoms9,10 and in a review published in 1985.11 Since the publication of the second edition,10 a more limited review of recent aspects of the chemistry of Tc and Re compounds that contain M–M bonds has become available.12 The present chapter will cover the literature up to the end of 2003 in a fully comprehensive fashion, but some topics will not be afforded the same detailed coverage they received in the previous edition, so the earlier text10 may still serve as a useful reference.
Although the chemistry of triangular [Re3]9+ clusters that contain Re=Re bonds was covered in previous editions of this text,9,10 these compounds will not be reviewed in this new addition. Instead, all higher nuclearity halide clusters of rhenium in which Re–Re multiple bonds are present will be the subject of a separate review that will be published elsewhere.438

Rhenium Compounds 273
Walton
8.2Synthesis and Structure of the Octachlorodirhenate(III) Anion
While the high pressure reduction of KReO4 and NH4ReO4 by molecular hydrogen in concentrated hydrochloric acid is interesting for historical reasons as an early route to salts of the [Re2Cl8]2- anion,13,14 this method has subsequently been used little. Among the reasons for this are corrosion of the pressure bomb and other practical difficulties such as the competing formation of [ReCl6]2-.15-17 In addition, the salt (Bu4N)2Re2Cl8, whose favorable solubility properties in a wide range of organic solvents make it the obvious choice for exploring the chemical reactivity of [Re2Cl8]2-, is available in good yield by more desirable routes. Nonetheless, the hydrogen reduction method does have certain features of note. It has in the past been the only source of the potassium and ammonium salts of [Re2Cl8]2-. These salts were in turn used as intermediates for the synthesis of other alkali metal salts of [Re2Cl8]2-, such as the cesium compound Cs2Re2Cl8·H2O.18 More recently, the autoclave reduction of (Et4N)ReO4 by dihydrogen in hydrochloric acid has been used to prepare (Et4N)2Re2Cl8.19 As might be expected, the ammonium and rubidium compounds, which are isolated as dihydrates, are isostructural with K2Re2Cl8·2H2O,20 whereas Cs2Re2Cl8·H2O has a different structure,18,20,21 although all of them contain the [Re2Cl8]2- anion.
The synthesis of the tetra-n-butylammonium salt (Bu4N)2Re2Cl8 in 1965 by the hypophosphorous acid reaction of [ReO4]- in aqueous hydrochloric acid provided ready access to a salt of [Re2Cl8]2- that had good solubility in a variety of polar organic solvents;14,22 this was important in enabling studies of the reaction chemistry of [Re2Cl8]2- to be carried out in non-aqueous media. The major disadvantage of this synthetic method is the relatively low yield (<40%) in which this salt is isolated, so that over the years alternative synthetic routes have been sought. These have included the reaction of Re3Cl9 with an excess of molten diethylammonium chloride which leads to the disruption of the Re3 cluster and the formation of (Et2NH2)2Re2Cl8.23,24 This method in turn suffers from one important disadvantage, namely, it requires a ready source of Re3Cl9. Another route involves the reaction of the rhenium(III) benzoate complex Re2(O2CPh)4Cl2, itself a compound that contains a Re–Re quadruple bond (see Section 8.4.2), with gaseous hydrogen chloride in methanol in the presence of cations such as tetra-n-bu- tylammonium and tetraphenylarsonium.25 This non-aqueous procedure has been adapted to produce the [Re2Br8]2- and [Re2I8]2- anions (see Section 8.3). A slight variation of this method, employing alkyl carboxylates of the type Re2(O2CR)4Cl2 in concentrated hydrochloric acid, has been used to prepare (Ph4As)2Re2Cl8 and Cs2Re2Cl8·H2O.14,21 There are other reactions in which [Re2Cl8]2- can be generated,26-28 including some that involve mononuclear starting materials,26,27 but these are not useful synthetic routes.
All of the preceding synthetic routes to (Bu4N)2Re2Cl8 and other salts with solubilizing organic cations have now been replaced by a much more convenient method. This straightforward one-pot synthesis involves the reaction of (Bu4N)ReO4 with refluxing benzoyl chloride at c. 210 °C, followed by the addition of an HCl(g) saturated solution of [Bu4N]+ in ethanol.29 By this means, (Bu4N)2Re2Cl8 can be prepared easily, quickly and in very high yield (c. 90%).
2(Bu4N)ReO4 + 8PhCOCl Α (Bu4N)2Re2Cl8 + organic products
It is believed29(a) that this reaction proceeds via the intermediacy of Re2(O2CPh)2Cl4, with the role of PhCOCl being to reduce and chlorinate the rhenium centers and couple them via the agency of benzoate bridges. The complex ReOCl3(PPh3)2 can be used as an alternative starting material,29(a) but since it is itself prepared from [ReO4]- this offers no real advantage.
The compound (Bu4N)2Re2Cl8 undergoes cation exchange reactions with various organic monoand dications to afford other salts of the [Re2Cl8]2- anion.30,31 In the case of (R3PCH2CH2PR3)Re2Cl8 (R = Cy or Ph), the reactions of the phosphine R3P with either
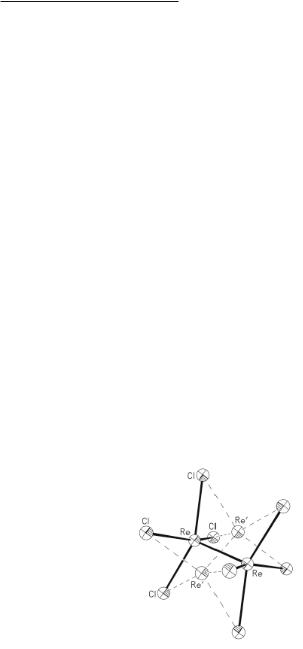
274Multiple Bonds Between Metal Atoms Chapter 8
(Bu4N)2Re2Cl8 or cis-Re2(µ-O2CCH3)2Cl4(py)2 in refluxing 1,2-dichloroethane can be used to give these salts.31 Alternatively, salts with organic cations can be obtained32 directly from solutions of [Re2Cl8]2- that are generated by the reaction of (Bu4N)ReO4 with PhCOCl,29 without first isolating (Bu4N)2Re2Cl8.
Crystal structure determinations on the salts Cs2Re2Cl8·H2O and (Bu4N)2Re2Cl8 have, in recent years, confirmed the essential structural features first established for the [Re2Cl8]2- anion in its potassium7(c) and pyridinium salts and discussed at some length in Chapter 1. The unit cell of the cesium salt Cs2Re2Cl8·H2O contains four [Re2Cl8]2- anions, two of which are anhydrous and the other two hydrated, with both axial positions occupied by water molecules (r(Re–O) = 2.66(3) Å).21 The Re–Re distance in the hydrated anion is, as expected, slightly longer (2.252(2) Å) than in the anhydrous species (2.237(2) Å). This result corrected an earlier structure determination18,20 that had led to the erroneous conclusion that [Re2Cl8(H2O)2]2- had an appreciably shorter Re–Re distance than [Re2Cl8]2- (2.210 Å versus 2.226 Å).
The structure of the tetra-n-butylammonium salt (Bu4N)2Re2Cl8 is of importance for two reasons. First it established the structure of the salt from which most of the reaction chemistry of the [Re2Cl8]2- anion has been developed (vide infra). Second, the study of the polarized crystal spectrum33 of this complex, so important to unraveling the details of the electronic structure of this molecule, required prior knowledge of the crystal structure. The usual eclipsed rotational conformation with a Re–Re distance of 2.222(2) Å was found33 in the structure determination. However, a complication in the structure solution was the observation that (Bu4N)2Re2Cl8 possesses a subtle form of disorder (Fig. 8.2), which is of a kind found subsequently with many other species of the type M2X8, including other salts of the [Re2X8]2- anions. When this type of orientational disorder is encountered it is usually a 2-fold disorder like that in (Bu4N)2Re2Cl8, although examples of 3-fold disorder also are known.19,31,32,34 The effect of high pressure on the structure of (Bu4N)2Re2Cl8 in dichloromethane has subsequently been investigated35 and the gas-phase electronic structure of the intact [Re2Cl8]2- anion has also been probed.36
Fig. 8.2. The structure of the [Re2Cl8]2- anion in (Bu4N)2Re2Cl8. The Re atoms are in positions with an occupation number of 73.89% while the Re' atoms are those with an occupation number of 26.11%. The common midpoint of the two Re-Re lines is a crystallographic center of inversion.
Since the report of the full structural characterization of (Bu4N)2Re2Cl8 in 1976,33 crystal structure determinations have been carried out on a large number of salts that contain the [Re2Cl8]2- anion.19,28,30-32,37-42 In most instances the anions are required by crystallographic symmetry to possess rigorously eclipsed rotational geometries (as reflected by a twist angle

Rhenium Compounds 275
Walton
ρ of zero), but in a few cases a small twisting is encountered. The Re–Re distances that have been determined for all the [Re2Cl8]2- salts are presented in Table 8.1, together with all other available structural data for compounds, to be discussed in due course, that contain Re–Re quadruple bonds, including salts of other octahalodirhenate(III) anions (Section 8.3). Some of these data are also included in a fairly extensive review published by Koz’min and Surazhskaya in 1980.43 The individual references should be consulted for information on the salts that show orientational disorder of the [Re2X8]2- anions (vide supra), although much of the work published pre-1995 has been reviewed.32,34 See also section 16.1.5.
Table 8.1. Structural Data for Dirhenium(III) Compounds Containing Re–Re Quadruple Bonds
|
|
Twist |
|
Compound |
r(Re–Re)(Å)a |
Angle (°)b |
ref. |
A. Compounds with No Bridging Ligands |
|
|
|
(Bu4N)2Re2F8·2Et2O |
2.188(3) |
c |
48 |
K2Re2Cl8·2H2O |
2.241(7) |
0 |
7(c) |
Cs2Re2Cl8·H2O: [Re2Cl8]2- |
2.237(2) |
0 |
21 |
[Re2Cl8(H2O)2]2- |
2.252(2) |
0 |
21 |
(NH4)2Re2Cl8·2H2O |
2.234(1) |
0 |
37 |
(C5H5NH)2Re2Cl8 |
2.244(15) |
0 |
44 |
[2,4,6-(CH3)3C5H2NH]2Re2Cl8 |
2.246(8) |
0 |
d |
(Et4N)2Re2Cl8 |
2.2146(7) |
0 |
19 |
(Bu4N)2Re2Cl8 |
2.222(2) |
0 |
33 |
[(DMF)2H]2Re2Cl8 |
2.221(1) |
0 |
38 |
[(CH3)2NH2]2Re2Cl8 |
2.235(2) |
c |
38 |
|
2.234(2) |
c |
38 |
(Prn3PH)2Re2Cl8 |
2.216(2) |
0 |
39 |
(Ph3MeP)2Re2Cl8 |
2.218(1) |
0 |
39 |
[ReCl2(depe)2]2Re2Cl8 |
2.213(1) |
0 |
40 |
[Rh2(O2CCH3)2(NCMe)6]Re2Cl8 |
2.226(4) |
c |
41 |
|
2.216(3) |
c |
41 |
|
2.211(3) |
c |
41 |
(Ph4P)2Re2Cl8·2CH2Cl2 |
2.222(1) |
0 |
42 |
(Ph4P)2Re2Cl8·2CH3CN |
2.229(2) |
0 |
28 |
(morphH)2Re2Cl8 |
2.222(1) |
0 |
30 |
[1,6-C6H12(NH3)2]Re2Cl8 |
2.2326(7) |
0 |
32 |
(Ph3PCH2CH2PPh3)Re2Cl8 |
2.2221(6) |
0 |
31 |
[(p-MeOC6H4)3MeP]2Re2Cl8 |
2.2231(6) |
0 |
142(b) |
|
2.2157(7) |
0 |
142(b) |
Cs2Re2Br8 |
2.228(4) |
0 |
49 |
(Bu4N)2Re2Br8 |
2.226(4) |
0 |
51 |
(Ph3MeP)2Re2Br8 |
2.226(1) |
0 |
39 |
(Bu4N)2Re2I8 |
2.245(3) |
0 |
60 |
(Ph4As)2Re2(NCS)8[(CH3)2CO]2 |
2.270(1) |
0 |
67 |
(Ph4As)2Re2(NCS)8(C5H5N)2 |
2.296(1) |
0 |
67 |
(Bu4N)2Re2(NCS)8(dto) |
2.2854(3) |
0 |
68 |
Li2Re2(CH3)8·2(C2H5)2O |
2.178(1) |
0 |
72 |
Re2Cl4(acac)2(DMSO)2 |
2.2650(5) |
0 |
145 |
Re2Cl4(acac)2(acacH)2 |
2.236(1) |
0 |
146 |

276Multiple Bonds Between Metal Atoms Chapter 8
|
|
|
Twist |
|
Compound |
r(Re–Re)(Å)a |
Angle (°)b |
ref. |
|
(Ph4As)Re2Cl7(PBun3) |
|
2.219(3) |
c |
194 |
(Ph4As)Re2Cl7(PBun2Ph) |
|
2.209(1) |
8.7 |
195 |
|
|
2.218(1) |
1.7 |
195 |
(Ph4As)Re2Cl7(PBunPh2) |
|
2.220(1) |
4.7 |
194 |
(Ph4As)Re2Cl7(PPhBzMe) |
2.2196(8) |
11.6 |
193 |
|
(Bu4N)4[Re2Cl7(PMe3)]2[Re2Cl8]·2CH2Cl2 |
2.210(1)e |
4.9 |
185 |
|
Re2Cl6(PMe3)2 |
(cubic form) |
2.208(1) |
0 |
179 |
|
(monoclinic form) |
2.209(1)f |
0 |
185 |
Re2Cl6(PEt3)2 |
|
2.222(3) |
0 |
187(a) |
Re2Cl6(PEt3)2·C7H8 |
|
2.219(1) |
0 |
187(b) |
Re2Cl6(PMe2Ph)2 |
|
2.215(1) |
0 |
180 |
|
|
2.212(1) |
0 |
180 |
Re2Cl6(PMePh2)2 |
|
2.227(1) |
0 |
188 |
Re2Cl4(OEt)2(PPh3)2 |
|
2.231(1) |
1.4 |
98 |
Re2Cl4(OMe)2[P(p-MeOPh)3]2 |
2.2476(4) |
§0 |
143 |
|
Re2Cl3(OEt)3(PPh3)2 |
|
2.2399(12) |
1.2 |
143 |
1,3-Re2Cl6(dppf) |
|
2.2444(3) |
4.8 |
190 |
1,3-Re2Cl6(dppf)·4C6H4Cl2 |
2.2390(6) |
§0 |
190 |
|
[1,3,6,8-Re2Cl4(PMe2Ph)4](PF6)2·CH3CN |
2.215(2) |
§0 |
243 |
|
(Bu4N)Re2Cl7(dth) |
|
2.257(1) |
2.4 |
211 |
(Bu4N)Re2Cl7(dto) |
|
2.248(1) |
2.3 |
211 |
|
B. Compounds with Carboxylato Bridges |
|
|
|
Re2(O2CC6H5)4Cl2·2CHCl3 |
2.235(2) |
0 |
89 |
|
Re2[O2CC(CH3)3]4Cl2 |
|
2.236(1) |
0 |
90 |
Re2[O2CC(CH3)3]4Br2 |
|
2.234(1) |
0 |
90 |
Re2(O2CC3H7)4(ReO4)2 |
|
2.251(2) |
0 |
78 |
Re2(O2CCH3)4Cl2 |
|
2.2240(5) |
0 |
91 |
Re2[O2C(2-biphenyl)]4Cl2·2CH2Cl2 |
2.2363(7) |
0 |
83 |
|
Re2(O2CAd)4Cl2·4CHCl3 |
|
2.2300(5) |
0 |
81 |
Re2[O2CCCHCo2(CO)6]4Cl2 |
2.240(1) |
0 |
88 |
|
Re2[O2CC(CH3)3]3Cl3 |
|
2.229(2) |
§0 |
114 |
Re2(O2CH)3Cl3 |
|
2.223(1) |
c |
107 |
Re2[O2CCH(CH3)2]3Cl2(ReO4) |
2.259(3) |
2.6 |
77 |
|
Re2(O2CCH3)2Cl4 |
|
2.208(1) |
0 |
110,111 |
Re2(O2CCH3)2Br4 |
|
2.216(3) |
0 |
112 |
Re2(O2CC6H5)2I4 |
|
2.198(1) |
0 |
58 |
Re2[O2CC(CH3)3]2Cl4 |
|
2.209(2) |
§0 |
114 |
Re2(O2CCH3)2Cl4(H2O)2 |
|
2.224(5) |
5.8 |
120 |
Re2(O2CCH3)2Cl4(DMSO)2 |
2.237(1) |
0 |
121 |
|
Re2(O2CCH3)2Cl4(DMF)2 |
2.239(2) |
c |
122 |
|
[Re2(O2CCH3)2Cl4(pyz)]2(µ-pyz) |
2.240(2) |
§0 |
102 |
|
[Re2(O2CCH3)2Cl4(µ-pyz)]n |
2.2358(8) |
§0 |
102 |
|
[Re2(O2CCH3)2Cl4(µ-4,4'-bpy)]n |
2.2512(4) |
§0 |
102 |
|
[Re2(O2CCH3)2Cl4(µ-dppmO2)]n |
2.2438(4) |
§0 |
102 |
|
[Re2(O2CCH3)2Cl4(INA)2]n |
2.2493(4) |
0 |
124 |
|
(Bu4N)Re2(O2CCH3)2Cl5·(CH3)2CO |
2.236(1) |
§0 |
101 |

Rhenium Compounds 277
|
|
|
Walton |
|
|
Twist |
|
Compound |
r(Re–Re)(Å)a |
Angle (°)b |
ref. |
Re2(O2CH)2Cl4(DPF)2 |
2.238(2) |
c |
104 |
[(C2H5)3NH]Re2(O2CH)3Cl4·HCO2Hg |
2.244(3) |
0 |
106 |
(NH4)2Re2(O2CH)2Cl6 |
2.260(5) |
0 |
123 |
Re2(O2CPh)2Cl4(THF)2·THF |
2.225(1) |
0 |
101 |
[ReCl2(dpcp)2]Re2(O2CPh)2Cl6·CHCl3 |
2.237(2) |
0 |
101 |
(Bu4N)Re2(O2CCF3)Cl6 |
2.2361(5) |
c |
88 |
Re2(O2CCH3)2(CH3)2(δ1-O2CCH3)2 |
2.177(1) |
0 |
132 |
Re2(O2CCH3)2Cl2(CH3)2(DMSO) |
2.184(1) |
c |
132 |
Re2(O2CC2H5)2(9-EtA)2Cl2·EtOH·C6H14 |
2.2455(10) |
0 |
134 |
Re2(O2CC2H5)(mhp)2Cl3 |
2.204(1) |
1.8 |
136 |
Re2(O2CCH3)Cl3(OMe)2(PCyPh2)2 |
2.2872(15) |
§0 |
143 |
|
2.2851(6) |
§0 |
143 |
C. Other Compounds |
|
|
|
Na2[Re2(SO4)4(H2O)2]·6H2O |
2.214(1) |
0 |
150 |
Cs2[Re2(HPO4)4(H3PO4)2] |
2.224(1) |
0 |
153 |
Re2(hp)4Cl2 |
2.206(2) |
0 |
154 |
Re2(mhp)2Cl4(Hmhp)·(CH3)2CO |
2.210(1) |
3.6 |
136 |
Re2(chp)3Cl3 |
2.2015(7) |
1.4 |
156 |
Re2(mp)4Cl2·2C6H6 |
2.2453(4) |
4.0 |
157 |
Re2(C7H4NS2)4Cl2·CH2Cl2 |
2.2716(3) |
18.0 |
158 |
Re2[(PhN)2CPh]2Cl4 |
2.177(2) |
0 |
159 |
Re2[(PhN)2CPh]2Cl4·THF |
2.209(1) |
6.0 |
159 |
Re2[(PhN)2CCH3]2Cl4 |
2.178(1) |
3.8 |
160 |
Re2[(CH3N)2CPh]4Cl2·CCl4 |
2.208(2) |
0 |
160 |
Re2[(p-CH3C6H4N)2CH]4Cl2·3C6H6 |
2.2759(3) |
0 |
161 |
Re2[(p-CH3C6H4N)2CH]4Cl2·2CH2Cl2 |
2.2705(5) |
1.6 |
161 |
Re2[(p-CH3C6H4N)2CH]4(OMe)2·3C6H6 |
2.3047(2) |
§0 |
161 |
Re2[(p-MeOC6H4N)2CH]4Cl2 |
2.2777(3) |
§0 |
162 |
{Re2[(p-MeOC6H4N)2CH]4Cl}BF4 |
2.2239(9) |
§0 |
163 |
Re2[(m-MeOC6H4N)2CH]4Cl2·2CH2Cl2 |
2.2765(6) |
§0 |
162 |
Re2[(3,4-Cl2C6H3N)2CH]4Cl2·2CH2Cl2 |
2.2783(4) |
§0 |
162 |
Re2[(3,5-Cl2C6H3N)2CH]4Cl2·4CH2Cl2 |
2.2734(3) |
§0 |
162 |
Re2[(3,5-Cl2C6H3N)2CH]4Cl2·THF |
2.2840(5) |
§0 |
162 |
Re2[(PhN)2CH]3Cl3 |
2.2318(8) |
c |
164 |
Re2[(PhN)2CH]3Cl3·2CH3CN |
2.2288(9) |
c |
164 |
Re2[(PhN)2CH]2Cl4 |
2.177(1)g |
§0 |
164 |
Re2[(PhN)2CH]2Cl4(H2O)·2THF |
2.2198(3) |
§0 |
165 |
Re2(hpp)4Cl2 |
2.1913(12) |
0 |
166 |
Re2(hpp)3Cl3·(CH3)2CO |
2.189(2) |
§0 |
166 |
cis-Re2(PhNCHO)4Cl2 |
2.2491(5) |
0 |
165 |
cis-Re2[PhNC(CH3)O]4Cl2·2CH3CN |
2.2304(6) |
§0 |
168 |
cis-Re2[HNC(Ph)O]4Cl2 |
2.2218(8) |
1.3 |
168 |
|
2.2181(6) |
§0 |
168 |
cis-Re2[PhNC(Ph)O]4Cl2·2CH2Cl2 |
2.2350(4) |
1.8 |
168 |
trans-Re2[µ-XylNC(CH3)O]4Cl2·2Et2O |
2.2364(5) |
1.7 |
168 |
trans-Re2[µ-XylNC(CH3)O]4(N3)2·CH2Cl2 |
2.2477(3) |
c |
169 |

278 Multiple Bonds Between Metal Atoms
Chapter 8
|
|
Twist |
|
Compound |
r(Re–Re)(Å)a |
Angle (°)b |
ref. |
trans-Re2[µ-XylNC(CH3)O]4(NCS)2·0.83CHCl3 |
2.2324(5) |
c |
169 |
(Bu4N){Re2[µ-HNC(CH3)O2]2Cl5}·3CH2Cl2 |
2.2395(5) |
c |
171 |
(Bu4N)2{Re2[µ-HNC(CH3O]Cl6}2 |
2.229(1) |
0 |
172 |
(Bu4N){Re2[µ-HNC(Ph)O]Cl6}·0.5CH2Cl2 |
2.2209(5) |
c |
173 |
(Bu4N){[Re2Cl6(DMF)2]2[µ-HNC(O)C6H4C(O)NH]} |
2.2317(7) |
c |
174 |
(Bu4N)Re2Cl7(bdppp)·CH2Cl2 |
2.275(1) |
h |
196 |
|
2.280(1) |
h |
196 |
Re2Cl6(S,S-isodiop)·CH2Cl2 |
2.224(2) |
§0 |
201 |
aWhere more than one set of data is given for any complex this signifies that more than one crystallographically independent molecule is present in the crystal. In cases where orientational disorder occurs, the Re–Re distance given is that for the dirhenium unit with the highest occupancy or is a weighted average of the
|
distances. |
b |
This is the average torsion angle ρav. An angle of §0 means 1.0° or less. |
c |
Not reported but evidently close to zero. |
d |
W. R. Robinson, Ph.D. Thesis, Massachusetts Institute of Technology, 1966. |
e |
This is the Re–Re distance for the [Re2Cl7(PMe3)]- anion. |
f |
This distance is the average for two crystallographically independent molecules in the asymmetric unit. |
g |
– |
|
In ref. 106 the space group is reported as P21/c with Z = 2. This would require 1 crystal symmetry, which is |
|
inconsistent with the reported structure. Accordingly, Z must equal 4 not 2. |
h |
All the L–Re–Re–L torsion angles are reported as being less than 12° for the two independent molecules. |
8.3Synthesis and Structure of the Other Octahalodirhenate(III) Anions
The other three octahalodirhenate(III) anions are all known though none is as extensively characterized nor so important in the discovery and development of the chemistry of compounds containing M–M multiple bonds as the classic [Re2Cl8]2- anion. Of the remaining anions, the most recent one to be discovered is the [Re2F8]2- anion, which has been prepared by reacting an excess of Bu4NF·3H2O with (Bu4N)2Re2Cl8 in freshly distilled dichloromethane.45 While the structure of the resulting salt, (Bu4N)2Re2F8·4H2O, was not confirmed for some time, its spectroscopic properties45,46 provided good support for this formulation. Later, this was substantiated by an EXAFS structure determination which yielded a Re–Re bond distance of 2.22 Å.47 Subsequently, the dark blue etherate (Bu4N)2Re2F8·2Et2O was prepared48 by the reaction of (Bu4N)2Re2Cl8 with Bu4NF in anhydrous CH2Cl2 followed by recrystallization from acetone/diethylether. Its crystal structure shows48 the Re–Re distance to be 2.188(3) Å and that a Et2O molecule is coordinated to one of the Re atoms thereby lowering the symmetry of the anion. The shortness of the Re–F distances in both structure determinations47,48 suggests the presence of significant Re–F / interactions. Surprisingly, studies on salts of the [Re2F8]2- anion have been very limited.
The first accurate structure determination of the [Re2Br8]2- anion was carried out on the cesium salt Cs2Re2Br8, which was prepared by the hypophosphorous acid reduction of KReO4 in 48% aqueous hydrobromic acid with CsBr present.49 The Re–Re bond length of 2.228(4) Å is very similar to that found for salts of the [Re2Cl8]2- anion (Table 8.1), and it has the same eclipsed rotational geometry. This structure determination on Cs2Re2Br8 was published several years after an early structure determination on the pyridinium salt “(C5H5NH)HReBr4” by Koz’min et al.50 It was concluded that this substance exists in two crystalline modifications with both structures being “constructed from the dimeric anions [Br4Re>ReBr4]4- (or [HBr4Re>ReBr4H]2-) and the pyridinium cations [C5H5NH]+.” The Re–Re bond lengths for these two forms were said50 to range from 2.207(3) to 2.27 Å. In view of the structural data for
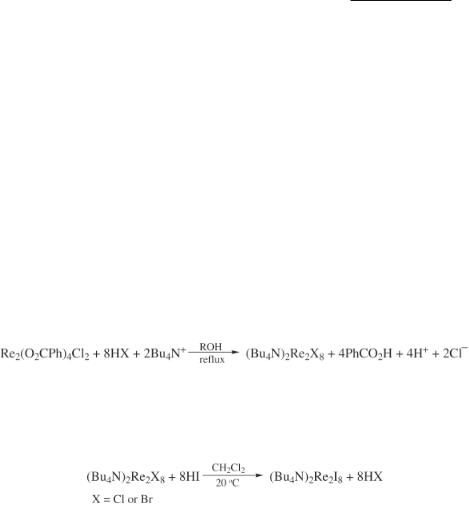
Rhenium Compounds 279
Walton
[Re2Cl8]2- which are listed in Table 8.1, the first of these distances seems too short while the second one is too long.
In addition to the preparative method described above for Cs2Re2Br8,49 a much simpler method for the synthesis of many salts of the octabromodirhenate(III) anion involves the halide exchange reactions of [Re2Cl8]2-. This necessitates the evaporation of a methanol solution of the appropriate [Re2Cl8]2- salt that contains 48% aqueous hydrobromic acid until crystallization of the olive-green salt is complete.14,22,24 This exchange reaction proceeds in almost quantitative yield and is ideal for the preparation of (Bu4N)2Re2Br8. The crystal structure of this salt shows51 a structure similar to that of its chloro analog including the same type of crystallographic disorder (see Section 8.2). The only other structural determinations on salts of the [Re2Br8]2- anion has been carried out on [(DMA)2H]2Re2Br8 (DMA = dimethylacetamide)52,53 and (Ph3MeP)2Re2Br8.39 In contrast to the structures of (Bu4N)2Re2Br8 and (Ph3MeP)2Re2Br8, where there are two orientations of the Re2 units,39,51 in the case of [(DMA)2H]2Re2Br8 the disorder involves three orientations of the mutually perpendicular Re2 units within the ordered arrangement of bromide ligands.53
An alternative method for preparing (Bu4N)2Re2Br8 involves the treatment of solutions of the dirhenium(III) benzoate complex Re2(O2CPh)4Cl2 (see Section 8.4.2) in methanol or ethanol with hydrogen bromide in the presence of tetra-n-butylammonium bromide.25 This strategy works equally well for the preparation of (Bu4N)2Re2Cl8 and (Bu4N)2Re2I8:
Not only did this reaction constitute the first general synthetic route to all three of these halo-anions, but it was the first time that a route to the [Re2I8]2- anion had been discovered.25 One year later (1979), a procedure related to the one described above for the synthesis of (Bu4N)2Re2I8 was reported.54 This reaction, which is the more convenient one of the two synthetic procedures, is as follows:
The conversion of the edge-sharing bioctahedral dirhenium(IV) complex (Bu4N)2Re2Br10 to (Bu4N)2Re2Br8 has been reported to occur when the former compound is reacted with Hg at 100 °C in a vacuum.55 While other salts of the [Re2Br8]2- anion have been reported, specifically (morphH)2Re2Br830 and [(δ5-C5H5)2Fe]2Re2Br8,56 neither has been fully characterized by X-ray crystallography although the spectroscopic and magnetic properties of the ferrocenium salt have been reported.56
As it turns out, the use of a non-aqueous solvent is essential for the preparation of pure [Re2I8]2- (vide supra) since it had been demonstrated in earlier studies,57,58 that the treatment of dirhenium(III) carboxylates with 55% aqueous HI produces Re2(O2CR)4I2 and/or Re2(O2CR)2I4 but not [Re2I8]2-. At that time, it was not at all clear that [Re2I8]2- would exist, although it had been noted58 that if the most likely bonded and non-bonded Re–Re, Re–I and I–I contacts for [Re2I8]2- were considered, then there was no obvious steric reason why this anion would not exist. Prior to the full structure determination of (Bu4N)2Re2I8 its Raman spectrum had been studied46,59 and interpreted in terms of the expected eclipsed structure for the [Re2I8]2- anion. The crystal structure of this complex, which was determined in 1988,60 confirms its close structural relationship to the chloro and bromo analogs. However, unlike (Bu4N)2Re2X8 (X = Cl or Br) there is a three-fold disorder of the Re2 units within the essentially cubic ar-

280Multiple Bonds Between Metal Atoms Chapter 8
ray of eight iodide ligands.60 The Re–Re distance of 2.245(3) Å is close to Re–Re distances in other [Re2X8]2-species (X = Cl or Br) (see Table 8.1). While on the subject of the structural identity of the [Re2I8]2- anion, the isolation of the compound Re4I8(CO)6 is of relevance.61 It is prepared by the I2 oxidation of Re2I2(CO)6(THF)2 in heptane, and has a structure that can be viewed formally as involving interactions between two [Re(CO)3]+ fragments and [Re2I8]2-; this association occurs via bent Re–I–Re bridges that involve six of the iodine atoms of [Re2I8]2-. As a consequence of this interaction, which gives rise to [(CO)3ReI3] units, the rotational conformation within the Re2I8 unit is staggered rather than eclipsed and, as a result, the Re–Re bond length is longer (2.279(1) Å)61 than that found in the eclipsed [Re2I8]2- species (Table 8.1). In accord with the usual bonding scheme for Re2L8 species, as the conformation changes from eclipsed to staggered, the dxy–dxy overlap diminishes and the metal-metal bond order decreases from four to three as the β contribution is lost.
8.4Substitution Reactions of the Octahalodirhenate(III) Anions that Proceed with Retention of the Re26+ Core
The synthesis and structural elucidation of the octahalodirhenate(III) anions has been paralleled by studies of their chemical reactivity particularly in the cases of [Re2Cl8]2- and [Re2Br8]2-. The reactions of these anions fall into three main categories: (1) substitution reactions in which a metal-metal bonded Re26+ core is retained, (2) redox reactions that give rise to products wherein the Re–Re bond order is less than four and in which ligand substitution reactions may also have occurred, and (3) reactions in which the metal-metal bond is disrupted. Since the latter reaction course is also encountered with compounds that contain Re–Re bond orders other than four (especially Re–Re triple bonds), such reactions are most conveniently treated all together in a separate section (see Section 8.7).
In this section, we consider the non-redox substitution reactions of the octahalodirhenate(III) anions and the chemistry of the compounds that result. We will also consider those compounds that while they may not have been prepared directly from the [Re2X8]2- anions, are derived formally from them by ligand for halide substitutions. Since the chemistry of the dirhenium(III) carboxylates is so extensive, these compounds will be considered on their own in Section 8.4.2.
8.4.1 Monodentate anionic ligands
Halide exchange reactions occur very readily, as has already been noted in Section 8.2. Thus, the reactions of (Bu4N)2Re2Cl8 with 48% aqueous hydrobromic acid have been used to prepare (Bu4N)2Re2Br814,22 and, in addition, the mixed haloanions [Re2Cl2Br6]2-, [Re2Cl3Br5]2- and [Re2Cl6Br2]2-.62 Since few experimental details were reported for the mixed haloanions62 it is not clear how easy it is to control the stoichiometry of these reactions. However, a few other methods exist for the preparation of mixed haloanions that involve less obvious but more controllable procedures, such as the preparations of (Et3PCl)2Re2Cl4Br4 from Re2Br4(PEt3)4 (see Section 8.5.4) and (Bu4N)2Re2Cl6Br2 from Re2Cl6(AsBun2Ph)2 (see Section 8.4.4).
Since the Re26+ core is preserved in exchange reactions between (Bu4N)2Re2Cl8 and HBr(aq), it can be expected that other coordinating anions will react in a similar fashion towards [Re2X8]2-. Among the first systems to be investigated were those involving the reactions between (Bu4N)2Re2Cl8 and an excess of thiocyanate and selenocyanate.63-65 Both NaSCN and KSeCN react with (Bu4N)2Re2Cl8 in non-aqueous media to produce dark red (Bu4N)2Re2(NCS)8 and purple (Bu4N)2Re2(NCSe)8, respectively.63-65 In the case of [Re2(NCS)8]2-, cation replacement reactions have been used63 to produce other salts, all of which display the same spectral properties as (Bu4N)2Re2(NCS)8. Initial infrared spectral characterizations on salts of both