
- •Application of Channel Fracturing in the Vaca Muerta Shale Formation
- •Introduction
- •Numerical Model for Predicting Fracture Conductivity under Closure Stress
- •The Relative Effect of Embedment upon Conventional and Channel Fracturing Performance
- •Relative role of aperture and proppant permeability damage
- •Influence of proppant mesh size
- •Discussions
- •Conclusions
- •Acknowledgments
- •References
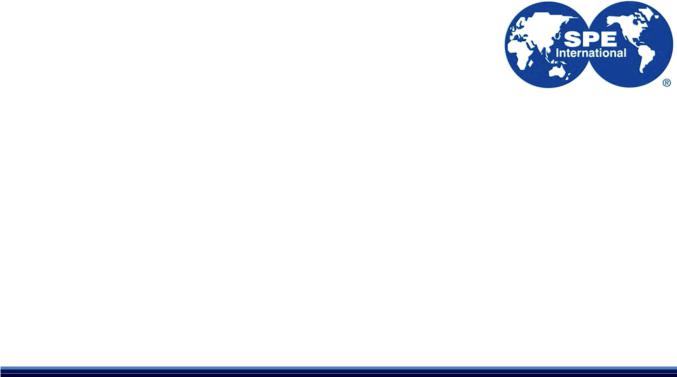
SPE-169383-MS
Application of Channel Fracturing in the Vaca Muerta Shale Formation
E.A. Ejofodomi, G. Cavazzoli, J. Morris, and R. Prioul, Schlumberger
Copyright 2014, Society of Petroleum Engineers
This paper was prepared for presentation at the SPE Latin American and Caribbean Petroleum Engineering Conference held in Maracaibo, Venezuela, 21–23 May 2014.
This paper was selected for presentation by an SPE program committee following review of information contained in an abstract submitted by the author(s). Contents of the paper have not been reviewed by the Society of Petroleum Engineers and are subject to correction by the author(s). The material does not necessarily reflect any position of the Society of Petroleum Engineers, its officers, or members. Electronic reproduction, distribution, or storage of any part of this paper without the written consent of the Society of Petroleum Engineers is prohibited. Permission to reproduce in print is restricted to an abstract of not more than 300 words; illustrations may not be copied. The abstract must contain conspicuous acknowledgment of SPE copyright.
Abstract
The channel hydraulic fracturing technique was recently introduced to the oil and gas industry as an alternative to the conventional hydraulic fracturing methodology. Channel fracturing involves the creation of a network of open channels or flow paths within the stimulated fracture volume, enabling the increased flow of hydrocarbons through the channels and into the wellbore. This paper investigates the applicability of this novel technique in the Vaca Muerta shale and presents a case history evaluating the potential production benefits of this methodology.
Starting with a calibrated geomechanical model, an index profile was created using the ratio between the young’s modulus and closure stress to determine if channel fracturing is applicable in the Vaca Muerta. The technique is compared to conventional hydraulic fracturing case to identify the differences in the created fracture properties. Based on the created channel fracturing index profile, the gross Vaca Muerta shale interval was divided into three intervals (lower, middle, and upper) and the process was repeated across each section to determine the incremental production impact across interval.
The result of this study shows that the channel fracturing technique is applicably in the Vaca Muerta shale and can potentially increase the production performance. The analysis also shows that this technique is more favorable primarily across the upper and middle Vaca Muerta intervals than the lower Vaca Muerta interval due to the high organics, low rock moduli, and high stress. Also, permeability degradation of the proppant pack does not negatively impact the overall conductivity in a Channel fracture system. Instead, loss of aperture reduces the conductivity by orders of magnitude.
Introduction
To date, about 220 wells have been drilled and completed with the Vaca Muerta Shale located in the Neuquén basin as the main production target, of which 90% are vertical completions. The majority of the wells require massive hydraulic fractures to achieve commercial rates. Typically, an average of four to five fracture stages are performed on a vertical well, while ten to seventeen stages are executed on horizontal well with lateral lengths between 3, 000 to 5, 000 ft. Today more than 1, 000 stages have been executed in Vaca Muerta Shale formation, with an average injection rate of 60 bpm at a maximum proppant concentration of 6 lbm/gal.
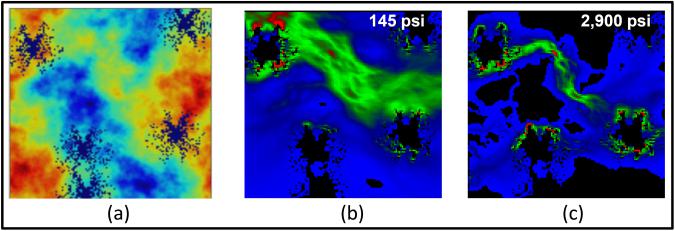
2 |
SPE-169383-MS |
|
|
Figure 1—Plan views of (a) aperture field and predicted fluid velocity fields within a heterogeneously propped fracture at (b) 145 psi and (c) 2, 900 psi closure stress respectively. Red corresponds to large aperture (a) or high local flow rate ((b) and (c)). Black indicates the presence of proppant. The numerical model supports detailed characterization of both variations in aperture due to fracture roughness and the heterogeneously placed proppant.
Channel fracturing technique was first performed in 2009 in Argentina on conventional reservoirs (Guillaret al., 2010). It was eventually introduced to the Vaca Muerta Shale formation in 2012, and to date, more than 50 wells having 210 fracture stages have successfully been treated with this technique. This has resulted in significant savings on the quantity of materials and chemicals required and also transportation costs. Additionally, the application of Channel fracturing has improved operational logistics with less time involved in the storage and handling of proppant. It is critical to properly understand its mechanisms and limitations with regards to the Vaca Muerta shale formation. With the large gross thickness of the Vaca Muerta(about 1,500 ft thick at the basin center (Lagaretta and Villar 2011)), it is imperative to determine if certain intervals are more favorable for Channel fracturing or not.
Numerical Model for Predicting Fracture Conductivity under Closure Stress
A detailed parametric study was performed to investigate the expected relative performance of conventional versus channel fracturing by explicitly addressing the spatial distribution of proppant within the fractures. For computational efficiency we employed a simulator based on the boundary element method. The fundamental concept for discretizing a detailed fracture surface and predicting its deformation under closure stress was presented by Pyrak-Nolte and Morris (2000) and has been modified to consider the mechanical influence of precipitated pillars within a fracture (Morris and Johnson, 2010). We have further extended the method to consider the combined influence of the formation deformation with a detailed discretization of the proppant within the fracture to predict the deformation and conductivity of the HPP channels. Using this approach, the performance of arbitrary distributions of proppant within a fracture can be simulated in detail. For example, Fig. 1 shows the prediction for a hypothetical heterogeneous distribution of proppant within a variable aperture fracture.
In this study, we assume that the conductivity reduction can be partitioned into two basic processes:
●Permeability damage within the proppant pack due to fines migration into the pack and proppant failure
●Aperture reduction due to embedement of the proppant packinto the formation
The computational model considers a periodic patch within the proppant distribution within the fracture. The conductivity model for the patch is initialized with a grid of cells containing a prescribed amount of proppant. In the case of conventional (homogenous) proppant placement, each cell of the calculation contains the same amount of proppant. For channel fracturing scenarios (heterogeneous
SPE-169383-MS |
3 |
|
|
proppant placement), each cell has its own individual quantity of proppant. For each scenario considered, proppant closure stress is progressively increased upon the fracture segment. For each level of stress applied the model calculates:
●Non-uniform spatial distribution of stress carried by the heterogeneous proppant within the fracture
●Detection of contact between fracture walls within channels and the stress carried by such points
●For each cell, given the local amount of proppant and the local stress, calculate the permeability and embedment
●Calculate the fracture segment conductivity, given the current distribution of compressed proppant and deformed aperture within the fracture segment
This process is repeated for each stress increment up to the maximum proppant stress considered. The model is sufficiently efficient that parameter studies exploring hundreds of scenarios may be performed on a laptop in a matter of minutes. In our study we repeated this approach for several hundred scenarios investigating the influence of properties related to the formation alone such as the moduli and stress as well as properties related to the interaction between the proppant and the formation such as the rate of embedment and ultimate embedment percentage. Here we present a subset of the results obtained, focusing upon the predicted behavior for a range of formation properties for the Vaca Muerta shale formation. Based on the varying log properties, the Vaca Muerta shale was divided into three separate intervals: upper, middle and lower. Fig. 2 shows a cross section of some of the log properties on the study well. A summary of the critical geomechnical data across each interval used for the analysis is shown in Table 1. Note that the embedment percentage is only reported for the final state at the end of the test. Consequently, the initial rate at which the embedment (and associated loss of aperture) occurs with increasing stress is not known. In our study we considered two end member cases:
●A “strong” scenario where the reported embedment occurred only as the ultimate stress of the test was reached at 9, 200 psi
●A “weak” scenario where the reported embedment was reached at a lower stress of 4, 500 psi
Weak and strong scenarios were simulated for each of the layers and predictions were made for increasing layers of 30/50 proppant (monolayer through 8 layers). The reduction in permeability of the proppant pack itself was inferred from conductivity measurements taken during the embedment tests (Fig. 3).
The Relative Effect of Embedment upon Conventional and Channel Fracturing Performance
With increasing stress, the conventional scenarios experience compaction of the proppant pack, embedment and permeability damage. For channel fracturing, such effects are also at work, but in addition there is the influence of progressive closure of the channels at very high proppant stress levels. At low stress, the channels remain open and provide orders of magnitude higher conductivity than the uniform pack. Consequently, for the same set of formation and proppant properties, we expect that at lower proppant stress-levels channel fracturing will provide the higher conductivity and that as the stress is progressively increased, at some point the channels will close and the conductivity will typically be less than the conventionally propped equivalent. With our model it is possible to predict the proppant stress level required to reduce channel fracturing conductivity to the level of conductivity achieved with conventional placement at that same stress. Furthermore, we can investigate how this crossover stress varies with formation-proppant properties and the geometry of the channels. Specifically we are interested in investigating the relative influence of formation modulus, embedment and permeability damage factors.
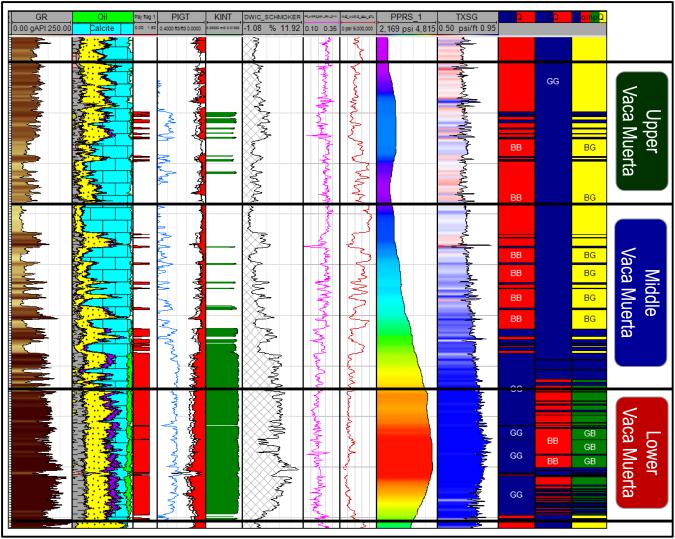
4 |
SPE-169383-MS |
|
|
Figure 2—Petrophysical and geomechanical logs across the Vaca Muerta shale formation. Track 1, gamma ray; Track 2, mineralogy; Track 3, pay flag; Track 4, water saturation (blue curve), total porosity (black curve), and effective porosity (red shading); Track 5, permeability; Track 6, Poisson’s ratio; Track 7, Young’s modulus; Track 9, pore pressure; Track 10, anisotropic minimum horizontal stress gradient (red 0.6 psi/ft, blue1 psi/ft); Track 11, reservoir quality index; Track 12, completion quality index; and Track 13, composite index.
Table 1—Average mechanical properties across the three Vaca Muerta shale sections used for simulation
Property |
Upper Vaca Muerta |
Middle Vaca Muerta |
Lower Vaca Muerta |
|
|
|
|
Young’s Modulus, MMpsi |
4.8 |
4.1 |
2.6 |
Poisson’s Ratio |
0.25 |
0.24 |
0.21 |
Min. Horizontal Stress Gradient, psi/ft |
0.72 |
0.75 |
0.9 |
Embedment % (1X) |
25 |
28 |
35 |
|
|
|
|
To summarize the results of the parameter study, we present the shift in the stress level at which channel fracturing intersects the conductivity achieved by uniformly distributed proppant (Fig. 4). We observe that the influence of embedment results in a relatively small shift of the crossover point. Furthermore, the relative shift in the crossover between conventional and channel fracturing is more sensitive to the variations in the rate of embedment, rather than the elastic properties of the formations. That is, all “strong” scenarios for the three sections resulted in a shift of approximately 5% or less in the crossover stress. In contrast, for one or two layers of proppant, the “weak” scenarios saw a shift of
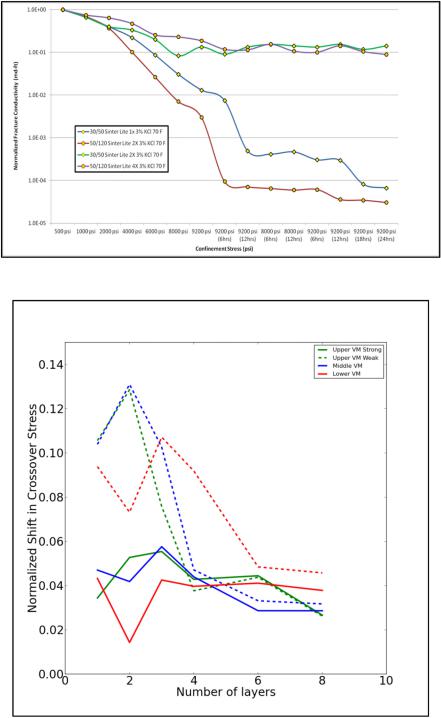
SPE-169383-MS |
5 |
|
|
Figure 3—Normalized fracture conductivity versus increasing closur stress.
Figure 4—The inclusion of embedment in the model results in a slight reduction in the proppant closure stress at which the channel fracturing conductivity intersections the conventional conductivity. Here we plot this change in crossover stress, normalized by the stress level at crossover. We see that embedment effects only shift the crossover by approximately 5% for the “strong” scenarios, independent of the number of 30/50 proppant layers. Although a larger shift is observed for the “weak” scenarios, increasing the numbers of layers of proppant to 4-5 layers reduces the shift to approximately 5%.
approximately 10%. However, with increasing proppant pack thickness, the “weak” scenarios saw a reduction in the shift and are comparable to the “strong” scenarios. In summary, these results indicate that for 4 or more layers of 30/50 proppant, the shift in the crossover stress should be 5% or less regardless of the rate of embedment assumed.