
Garrett R.H., Grisham C.M. - Biochemistry (1999)(2nd ed.)(en)
.pdf
21.4 ● Complex I: NADH–Coenzyme Q Reductase |
681 |
The components of the electron transport chain can be purified from the mitochondrial inner membrane. Solubilization of the membranes containing the electron transport chain results in the isolation of four distinct protein complexes, and the complete chain can thus be considered to be composed of four parts: (I) NADH–coenzyme Q reductase, (II) succinate–coenzyme Q reductase, (III) coenzyme Q–cytochrome c reductase, and (IV) cytochrome c oxidase (Figure 21.4). Complex I accepts electrons from NADH, serving as a link between glycolysis, the TCA cycle, fatty acid oxidation, and the electron transport chain. Complex II includes succinate dehydrogenase and thus forms a direct link between the TCA cycle and electron transport. Complexes I and II produce a common product, reduced coenzyme Q (UQH2), which is the substrate for coenzyme Q–cytochrome c reductase (Complex III). As shown in Figure 21.4, there are two other ways to feed electrons to UQ: the electrontransferring flavoprotein, which transfers electrons from the flavoproteinlinked step of fatty acyl-CoA dehydrogenase, and sn-glycerophosphate dehydrogenase. Complex III oxidizes UQH2 while reducing cytochrome c, which in turn is the substrate for Complex IV, cytochrome c oxidase. Complex IV is responsible for reducing molecular oxygen. Each of the complexes shown in Figure 21.4 is a large multisubunit complex embedded within the inner mitochondrial membrane.
21.4 ● Complex I: NADH–Coenzyme Q Reductase
As its name implies, this complex transfers a pair of electrons from NADH to coenzyme Q, a small, hydrophobic, yellow compound. Another common name for this enzyme complex is NADH dehydrogenase. The complex (with an estimated mass of 850 kD) involves more than 30 polypeptide chains, one molecule of flavin mononucleotide (FMN), and as many as seven Fe-S clusters, together containing a total of 20 to 26 iron atoms (Table 21.2). By virtue of its dependence on FMN, NADH–UQ reductase is a flavoprotein.
Table 21.2
Protein Complexes of the Mitochondrial Electron-Transport Chain
|
Mass |
|
Prosthetic |
|
Complex |
(kD) |
Subunits |
Group |
Binding Site for: |
|
|
|
|
|
NADH–UQ reductase |
850 |
30 |
FMN |
NADH (matrix side) |
|
|
|
Fe-S |
UQ (lipid core) |
Succinate–UQ reductase |
140 |
4 |
FAD |
Succinate (matrix side) |
|
|
|
Fe-S |
UQ (lipid core) |
UQ–Cyt c reductase |
250 |
9–10 |
Heme bL |
Cyt c (intermembrane |
|
|
|
Heme bH |
space side) |
|
|
|
Heme c1 |
|
|
|
|
Fe-S |
|
Cytochrome c |
13 |
1 |
Heme c |
Cyt c1 |
|
|
|
|
Cyt a |
Cytochrome c oxidase |
162 |
10 |
Heme a |
Cyt c (intermembrane |
|
|
|
Heme a3 |
space side) |
|
|
|
CuA |
|
|
|
|
CuB |
|
Adapted from: Hatefi, Y., 1985. The mitochondrial electron transport chain and oxidative phosphorylation system. Annual Review of Biochemistry 54:1015–1069; and DePierre, J., and Ernster, L., 1977. Enzyme topology of intracellular membranes. Annual Review of Biochemistry 46:201–262.

682 Chapter 21 ● Electron Transport and Oxidative Phosphorylation
Although the precise mechanism of the NADH–UQ reductase is not known, the first step involves binding of NADH to the enzyme on the matrix side of the inner mitochondrial membrane, and transfer of electrons from
NADH to tightly bound FMN: |
|
NADH [FMN] H 88n [FMNH2] NAD |
(21.17) |
The second step involves the transfer of electrons from the reduced [FMNH2] to a series of Fe-S proteins, including both 2Fe-2S and 4Fe-4S clusters (see Figures 20.8 and 20.16). The unique redox properties of the flavin group of FMN are probably important here. NADH is a two-electron donor, whereas the Fe-S proteins are one-electron transfer agents. The flavin of FMN has three redox states—the oxidized, semiquinone, and reduced states. It can act as either a one-electron or a two-electron transfer agent and may serve as a critical link between NADH and the Fe-S proteins.
The final step of the reaction involves the transfer of two electrons from iron–sulfur clusters to coenzyme Q. Coenzyme Q is a mobile electron carrier. Its isoprenoid tail makes it highly hydrophobic, and it diffuses freely in the hydrophobic core of the inner mitochondrial membrane. As a result, it shuttles electrons from Complexes I and II to Complex III. The redox cycle of UQ is shown in Figure 21.5, and the overall scheme is shown schematically in Figure 21.6.
Complex I Transports Protons from the Matrix to the Cytosol
The oxidation of one NADH and the reduction of one UQ by NADH–UQ reductase results in the net transport of protons from the matrix side to the cytosolic side of the inner membrane. The cytosolic side, where H accumulates, is referred to as the P (for positive) face; similarly, the matrix side is the N (for negative) face. Some of the energy liberated by the flow of electrons
(a) |
O |
|
|
|
|
|
|
|
|
|
|
|
|
|
e– + H+ |
O• |
|
|
|
e– + H+ |
|
|
OH |
|
|
|
|||||||||
|
|
|
|
|
|
|
|
|
|
|
|
|
|
|
CH3 |
|
|
|
|
|
|
|
|
|
|
|
|||||||||
H3CO |
|
|
|
|
|
|
|
|
CH3 |
|
|
|
CH3O |
CH3 |
|
H3CO |
|
|
|
|
|
|
|
CH3 |
|||||||||||
|
|
|
|
|
|
|
|
|
|||||||||||||||||||||||||||
|
|
|
|
|
|
|
|
|
|
|
|
|
|
|
|
|
|
|
|
|
|
|
|
|
|
|
|
|
|
|
|
|
|
|
|
|
|
|
|
|
|
|
|
|
|
|
|
|
|
|
|
|
|
|
|
|
|
|
|
|
|
|
|
|
|
|
|
|
|
|
|
H3CO |
|
|
|
|
|
|
|
|
|
(CH2 |
|
CH |
|
C |
|
CH2)10 |
|
H |
|
CH3O |
R |
|
H3CO |
|
|
|
|
|
|
|
R |
||||
|
|
|
|
|
|
|
|
|
|
|
|
|
|
|
|
|
|
|
|
|
|
||||||||||||||
|
|
|
|
|
|
|
|
|
|
|
|
|
|||||||||||||||||||||||
|
|
|
|
|
|
|
|
|
|
|
|
|
|
|
|
|
|
|
|
||||||||||||||||
|
|
|
|
|
|
|
|
|
|
|
|
|
|
|
|
|
|
|
|
|
|
|
|
|
|
|
|
|
|
|
|
|
|
|
|
|
|
|
|
|
|
|
|
|
|
|
|
|
|
|
|
|
|
|
|
|
|
OH |
|
|
|
|
|
|
OH |
|
|
|
|||
|
|
|
|
|
O |
|
|
|
|
|
|
|
|
|
|
|
|
|
|
|
|
|
|
|
|
|
|
|
|
||||||
|
|
|
|
|
|
Coenzyme Q, oxidized form |
|
Semiquinone |
|
|
|
Coenzyme Q, |
|||||||||||||||||||||||
|
|
|
|
|
|
|
|
|
|
(Q, ubiquinone) |
|
intermediate |
|
|
|
reduced form |
|||||||||||||||||||
|
|
|
|
|
|
|
|
|
|
|
|
|
|
|
|
|
|
|
|
|
(QH •) |
|
|
(QH2, ubiquinol) |
(b)
● (a) The three oxidation states of coenzyme Q.
(b) A space-filling model of coenzyme Q.

21.5 ● Complex II: Succinate–Coenzyme Q Reductase |
683 |
|
2 H+ |
2 H+ |
|
|
Intermembrane |
|
|
|
|
space |
|
|
|
|
(P-Phase) |
|
|
|
|
|
|
2 |
2 |
|
|
|
e– |
UQH2 |
|
UQH2 |
UQ |
2Fe-S |
e– |
|
Centers |
UQ |
|||
|
|
|
|
|
|
2Fe-S |
|
|
|
2 H+ |
Centers |
|
HP |
|
|
|
2 H+ |
||
Matrix |
|
|
|
|
(N-Phase) |
|
|
2 H+ |
|
2 H+
FMNH2 FMN
FP + IP
NAD+ NADH + H+
FIGURE 21.6 ● Proposed structure and electron transport pathway for Complex I. Three protein complexes have been isolated, including the flavoprotein (FP), iron–sulfur protein (IP), and hydrophobic protein (HP). FP contains three peptides (of mass 51, 24, and 10 kD) and bound FMN and has 2 Fe-S centers (a 2Fe-2S center and a 4Fe-4S center). IP contains six peptides and at least 3 Fe-S centers. HP contains at least seven peptides and one Fe-S center.
through this complex is used in a coupled process to drive the transport of protons across the membrane. (This is an example of active transport, a phenomenon examined in detail in Chapter 10.) Available experimental evidence suggests a stoichiometry of four H transported per two electrons passed from NADH to UQ.
21.5 ● Complex II: Succinate–Coenzyme Q Reductase
Complex II is perhaps better known by its other name—succinate dehydrogenase, the only TCA cycle enzyme that is an integral membrane protein in the inner mitochondrial membrane. This enzyme has a mass of approximately 100 to 140 kD and is composed of four subunits: two Fe-S proteins of masses 70 kD and 27 kD, and two other peptides of masses 15 kD and 13 kD. Also known as flavoprotein 2 (FP2), it contains an FAD covalently bound to a histidine residue (see Figure 20.15), and three Fe-S centers: a 4Fe-4S cluster, a 3Fe-4S cluster, and a 2Fe-2S cluster. When succinate is converted to fumarate in the TCA cycle, concomitant reduction of bound FAD to FADH2 occurs in succinate dehydrogenase. This FADH2 transfers its electrons immediately to Fe-S centers, which pass them on to UQ. Electron flow from succinate to UQ,
Succinate 88n fumarate 2 H 2 e |
(21.18) |
UQ 2 H 2 e 88n UQH2 |
(21.19) |
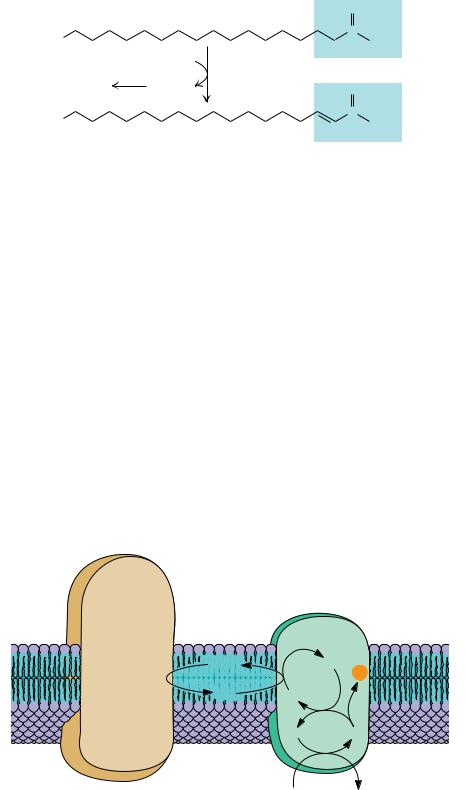
684 Chapter 21 ● Electron Transport and Oxidative Phosphorylation
|
|
O |
H3C |
|
C |
|
SCoA |
|
|
[FAD] |
|
Complex II |
[FADH2] |
O |
H3C |
|
C |
|
SCoA |
● The fatty acyl-CoA dehydrogenase reaction, emphasizing that the reaction involves reduction of enzyme-bound FAD (indicated by brackets).
Net rxn: Succinate UQ 88n fumarate UQH2 |
0.029V |
(21.20) |
|
° |
|
|
G ° 5.6 kJ mol |
|
yields a net reduction potential of 0.029 V. (Note that the first half-reaction is written in the direction of the e flow. As always, o is calculated according to Equation 21.9.) The small free energy change of this reaction is not sufficient to drive the transport of protons across the inner mitochondrial membrane.
This is a crucial point because (as we will see) proton transport is coupled with ATP synthesis. Oxidation of one FADH2 in the electron transport chain results in synthesis of approximately two molecules of ATP, compared with the approximately three ATPs produced by the oxidation of one NADH. Other enzymes can also supply electrons to UQ, including mitochondrial sn-glyc- erophosphate dehydrogenase, an inner membrane–bound shuttle enzyme, and the fatty acyl–CoA dehydrogenases, three soluble matrix enzymes involved in fatty acid oxidation (Figure 21.7; also see Chapter 24). The path of electrons from succinate to UQ is shown in Figure 21.8.
Complex III
|
Complex II |
Intermembrane |
|
|
space |
||
UQH2 |
|
2Fe3+ |
|
|
|
|
2 H+ |
UQ |
2Fe2+ |
|
|
|
FAD |
|
FADH2 |
|
|
|
Matrix |
|
Succinate |
|
Fumarate |
FIGURE 21.8 ● A probable scheme for electron flow in Complex II. Oxidation of succinate occurs with reduction of [FAD]. Electrons are then passed to Fe-S centers and then to coenzyme Q (UQ). Proton transport does not occur in this complex.


686 Chapter 21 ● Electron Transport and Oxidative Phosphorylation
FIGURE 21.11 ● The structure of UQ-cyt c reductase, also known as the cytochrome bc1 complex. The alpha helices of cytochrome b (pale green) define the transmembrane domain of the protein. The bottom of the
structure as shown extends approximately 75 A into the mitochondrial matrix, and the top of
the structure as shown extends about 38 A into the intermembrane space. (Photograph kindly provided by Di Xia and Johann Deisenhofer [From Xia, D., Yu, C.-A., Kim, H., Xia, J.-Z., Kachurin, A. M., Zhang, L., Yu, L., and Deisenhofer, J., 1997. The crystal structure of the cytochrome bc1 complex from bovine heart mitochondria. Science 277:60–66.])
reductase contains a b-type cytochrome, of 30 to 40 kD, with two different heme sites (Figure 21.11) and one c-type cytochrome. (One other variation, heme a, contains a 15-carbon isoprenoid chain on a modified vinyl group, and a formyl group in place of one of the methyls [see Figure 21.10]. Cytochrome a is found in two forms in Complex IV of the electron transport chain, as we shall see.) The two hemes on the b cytochrome polypeptide in UQ–cyt c reductase are distinguished by their reduction potentials and the wavelength ( max) of the socalled band (see Figure 21.9). One of these hemes, known as bL or b566, has a standard reduction potential, ° , of 0.100 V and a wavelength of maximal absorbance ( max) of 566 nm. The other, known as bH or b562, has a standard reduction potential of 0.050 V and a max of 562 nm. (H and L here refer to high and low reduction potential.)
The structure of the UQ–cyt c reductase, also known as the cytochrome bc1 complex, has been determined by Johann Deisenhofer and his colleagues. (Deisenhofer was a co-recipient of the Nobel Prize in Chemistry for his work on the structure of a photosynthetic reaction center [see Chapter 22]). The complex is a dimer, with each monomer consisting of 11 protein subunits and 2165 amino acid residues (monomer mass, 248 kD). The dimeric structure is pear-shaped and consists of a large domain that extends 75 Å into the mito-



