
Fundamentals of the Physics of Solids / 07-The Structure of Crystals
.pdf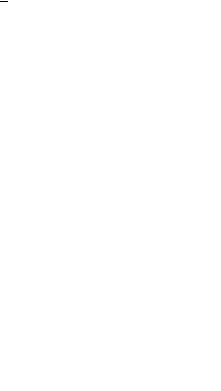
7.2 Cubic Crystal Structures |
223 |
1 1 1 . The coordinates for the eight ions in the Bravais cell are
4 4 4
Zn : 000, 0 |
1 1 |
, |
1 |
0 |
1 |
, |
1 1 |
0 , |
S : |
1 1 1 |
, |
1 3 3 |
, |
3 1 3 |
, |
3 3 1 . |
|
2 2 |
|
2 |
|
2 |
|
2 2 |
|
|
4 4 4 |
|
4 4 4 |
|
4 4 4 |
|
4 4 4 |
Compared to the diamond structure, the symmetry is lower. Inversion is no longer a symmetry, even when combined with a translation. The space group
¯ |
2 |
is therefore F 43m (Td ). Apart from the prototype, ZnS, several other com-
pounds of composition AB crystallize in this form, many of which are important in semiconductor technology. For example, A = Zn, Cd, Hg, and B = Se, Te, S, or A = Ga, Al, In, and B = P, As, Sb.
Starting with the sphalerite structure, the addition of a third kind of atom to each empty octahedral site of face-centered Bravais cell leads to C1b structure (in the Pearson notation: cF12). The prototype is AgAsMg. The space group is again F 43m (Td2). This arrangement is also called a half-Heusler structure as it can be conceptually derived from a Heusler alloy (AlMnCu2) by leaving half of the tetrahedral sites empty.
¯ 7
Contrarily, the space group F d3m (Oh) of the diamond structure can be preserved by a suitable choice of filling its empty octant sites. This occurs in the C15 or Cu2Mg structure (Fig. 7.17), the prototype for a class of Laves phase alloys. Just like in diamond, the eight magnesium atoms are arranged tetrahedrally in the Bravais cell: at the vertices and face centers plus at the centers of four of eight octants, while the sixteen Cu atoms are grouped four by four, in tetrahedra around the centers of the other four octants. The edge length of these small tetrahedra is such that the four Cu atoms closest to the center of the Bravais cell form an identical tetrahedron.
Fig. 7.17. Cu2Mg, the prototype of C15 (cF24) structures
¯ |
7 |
The same space group F d3m (Oh) is present in the spinel (or H11) struc-
ture, named after the mineral MgAl2O4. The general composition of the compounds that crystallize in this structure is X2+Y3+2 O4, where X stands for Mg, Fe, Zn, Mn, etc., and Y for Al, Fe, or Cr. Accordingly, one speaks of aluminate, ferrite, and chromite spinels. This structure type is of particular

224 7 The Structure of Crystals
importance because antiferromagnetic and ferrimagnetic ferrites that are extensively used in electrotechnology crystallize in this geometry. Oxygen atoms are located at the vertices and face centers of the cubic lattice, X2+ cations occupy one-eighth of the tetrahedral interstices, and Y3+ cations – one-half of the octahedral ones. As the cations are not at the same positions in each cube, the Bravais cell contains eight cubes and eight molecules – 56 atoms in all (cF56). In the inverse spinel structure divalent cations are at octahedral sites and half of the trivalent cations are at tetrahedral sites. Such an inverse spinel structure is observed in magnetite, in which X2+ and Y3+ are diand trivalent ions of the same element, iron.
7.3 Hexagonal Crystal Structures
We have already seen (Fig. 7.12) that atoms are arranged in a hexagonal array in the (111) plane of a face-centered cubic crystal. Within the plane, each atom is surrounded by six neighbors, which is the closest packing in two dimensions. When such a hexagonal planar lattice is repeated at regular intervals along the perpendicular direction, as shown in 7.18(a), a simple hexagonal lattice is obtained.
(a) |
(b) |
(c) |
(d) |
Fig. 7.18. Hexagonal structures: (a): simple; (b) and (c): close-packed; (d) double close-packed
Denoting the lattice constant in the hexagonal plane by a and in the perpendicular direction by c, a possible choice for the primitive vectors in
Cartesian coordinates is |
|
|
|
|
|
|||
|
√ |
|
|
1 |
|
|
|
|
a1 = |
|
3 |
axˆ − |
ayˆ , |
a2 = ayˆ , |
a3 = czˆ . |
(7.3.1) |
|
2 |
2 |

|
|
|
|
|
|
|
|
|
7.3 |
Hexagonal Crystal Structures |
225 |
||||||||||||
Matrices A and B are |
|
|
|
|
|
|
|
|
|
|
|
|
|
|
|
|
|
||||||
Ahex = |
√ |
|
|
|
|
|
, |
|
|
|
|
|
|
|
|
√ |
|
|
|
|
. |
|
|
|
a/2 a 0 |
|
Bhex = 2π |
1/(√3a) 1/a |
0 |
(7.3.2) |
|||||||||||||||||
|
|
3a/2 0 0 |
|
|
|
|
|
|
|
|
|
2/( |
3a) 0 0 |
|
|
||||||||
−0 0 c |
|
|
|
|
|
|
|
|
|
0 |
|
0 1/c |
|
|
|||||||||
|
|
|
|
|
|
|
|
|
|
|
|
|
|
|
|
|
|
|
|
|
|
|
|
The primitive vectors of the reciprocal lattice are then |
|
|
|
||||||||||||||||||||
|
|
= |
4π |
|
|
= |
2π |
+ |
|
2π |
|
b3 = |
2π |
|
|
|
|||||||
b1 |
√ |
|
xˆ , |
b2 |
√ |
|
xˆ |
|
|
yˆ , |
|
zˆ . |
(7.3.3) |
||||||||||
|
a |
c |
|||||||||||||||||||||
|
|
|
|||||||||||||||||||||
|
|
|
|
|
3a |
|
|
|
|
3a |
|
|
|
|
|
|
|
|
|
|
|
As shown in Fig. 7.19, these vectors also generate a hexagonal lattice with
√
lattice parameters a = 4π/ 3a and c = 2π/c, however, the reciprocallattice primitive vectors are rotated around the z-axis with respect to their direct-lattice counterparts, and their angle is 60◦ instead of 120◦.
kz |
kz |
4 a |
|
|
|
|
|
|
|
b3 |
|
L |
R |
A S H |
|
|
|
|
|
S' |
|
|
2 c |
|
|
U |
|
|
|
|
|
P |
|
|||
b1 |
|
k |
k |
|||
|
b2 |
y |
|
|
|
y |
|
k |
M |
|
T K |
|
|
|
|
|
|
|||
|
|
|
x |
T' |
|
|
kx |
|
|
|
|
||
|
|
|
|
|
|
Fig. 7.19. The reciprocal lattice of a hexagonal lattice and its Brillouin zone with special points
The lattice points of a simple hexagonal lattice are directly above each other along the z direction. When this lattice is decorated with a single atom of radius r, the lattice is most densely filled when the atoms are in contact with each other both in the hexagonal plane and in the perpendicular direction. This occurs for a = c = 2r, and thus the volume of the hexagonal cell is
|
|
√ |
|
|
|
|
√ |
|
|
|
|
|
|
|
|
|
3 |
2 |
|
|
|
3 |
|
|
|||
|
|
|
|
|
|
|
|
||||||
|
|
|
|
a |
|
c = 4 |
|
3r |
|
. |
(7.3.4) |
||
|
2 |
|
|
|
|
||||||||
The packing fraction is therefore |
|
− |
|
3√3 |
|
|
|||||||
3 r3 |
π 4√3r3 |
= |
= 0.605 . |
(7.3.5) |
|||||||||
4 |
|
|
|
|
|
|
1 |
|
π |
|
|
|
This is 20% smaller than the atomic packing factor of the fcc structure. This is why the above simple hexagonal structure may not occur naturally.
Space filling is more e cient if the planes of hexagonally arranged atoms are not stacked directly on top of each other, but the atomic positions are slightly shifted in every second layer, and only the next-nearest layers come

226 7 The Structure of Crystals
exactly above each other, as shown in Fig. 7.18(b). It is readily established that in the closest-packed arrangement of two subsequent layers each atom in the upper layer sits exactly into the dip among three touching spheres of the lower layer. Then the height di erence between the centers of the spheres of
the two layers is 8/3r. Since the lattice parameter in the hexagonal plane is 2r and the periodicity in the perpendicular direction is twice the repeat
distance of the layers, close packing is achieved if c/a = 8/3 ≈ 1.633. As the cell now contains two atoms, the packing fraction is
2 |
/ |
8 |
|
|
3√3 |
= |
3√2 |
= 0.740 . |
(7.3.6) |
||
|
|
3 |
|
|
π |
|
π |
|
|
||
|
|
|
|
|
|
|
|
|
|
|
|
This is the same as the atomic packing factor of the close-packed fcc lattice. The structure with this particular c/a value is called the hexagonal closepacked (hcp) structure. It is also called Mg or A3 structure.
This structure can also be viewed as the superposition of two simple hexag-
onal Bravais lattices, with the sublattices displaced by |
|
|
|
||||||||||||||||||||||||
2 |
|
1 |
|
|
|
1 |
|
|
|
|
|
√ |
|
|
|
|
|
1 |
|
|
|
|
|||||
|
|
|
|
|
|
|
|
|
|
3 |
axˆ + |
|
|
|
|
||||||||||||
|
|
|
|
a1 + |
|
a2 + |
|
a3 |
= |
|
|
|
|
czˆ |
(7.3.7) |
||||||||||||
|
|
|
3 |
3 |
2 |
3 |
|
2 |
|||||||||||||||||||
or |
|
|
|
|
|
|
|
|
√ |
|
|
|
|
|
|
|
|
|
|
|
|
|
|||||
1 |
|
|
|
2 |
|
|
|
1 |
|
|
|
|
|
3 |
|
|
|
1 |
|
|
|
1 |
|
|
|||
|
|
a1 + |
|
a2 + |
|
a3 = |
|
|
axˆ |
+ |
|
ayˆ + |
|
czˆ |
(7.3.8) |
||||||||||||
|
3 |
3 |
2 |
|
6 |
2 |
2 |
with respect to one another. Such a crystal has a diatomic basis and thus no longer possesses each rotational symmetry of the hexagonal lattice. To facilitate the visualization of symmetries, atomic positions are shown in a displaced coordinate system in Fig. 7.18(c). In an oblique coordinate system particularly well suited to hexagonal symmetry, with the x- and y-axes making an angle of 120◦ with each other, the coordinates of the two atoms of the
primitive cell are 23 13 0 and 13 23 12 . It is readily seen that the z-axis is not a sixfold rotation axis now but a sixfold screw axis: if a rotation through 60◦ around the z-axis is followed by a translation along the z-axis through c/2, the crystal is taken into itself. The space group of a close-packed hexagonal crystal is therefore P 63/mmc (D64h).
A somewhat more complicated structure with the same symmetry is obtained by another way of stacking hexagonal layers. Four simple hexagonal
sublattices may be superposed in such a way that the first, second, and
third are displaced by 23 a1 + 13 a2 + 14 a3, 12 a3, and 13 a1 + 23 a2 + 34 a3 relative to the first. This leads to the double hexagonal close-packed (dhcp) struc-
ture, also called La structure or A3 structure. Ideal close-packing occurs for
c/a = 2 8/3 = 3.266.
Over 30 elements crystallize naturally in hcp or dhcp structure, e.g., Be, Cd, Co, He, La, Mg, Os, Sc, Ti, Y, Zn, Zr, as well as several lanthanoids and actinoids. In these materials the ratio of the two lattice constants is not the ideal value c/a = 1.633 or its double but it is usually very close to one of

7.3 Hexagonal Crystal Structures |
227 |
them. At high pressures, helium also crystallizes in a hexagonal structure, and packing is almost ideal: c/a = 1.631. The lattice constant ratio is very close to the ideal value also in alkali metals and the prototype Mg (c/a = 1.637 for Li, 1.634 in Na, and 1.624 in Mg). Lanthanum crystallizes in a dhcp structure with c/a = 2 × 1.6125. Much larger discrepancies occur in Be (c/a = 1.568), as well as in Zn and Cd (1.856 and 1.886). Nevertheless even these structures are called close-packed.
In these structures the close-packed layers are stacked on top of each other as close as possible. As shown in Fig. 7.20, there are two di erent ways to stack a hexagonal close-packed layer on another by exploiting the dips among the atoms. If the arrangement of the first plane is called A, that of the next is called B or C, depending on whether it is like part (a) or (b) in Fig. 7.20.
(a) |
(b) |
Fig. 7.20. Two possible ways of stacking hexagonal close-packed layers. Dashed-line circles (spheres) centered at asterisks ( ) mark the atoms of the lower layer. Relative to this layer (A), two arrangements – shown in (a) and (b) – are possible for the centers (•) of the spheres in the second layer. They are called type B and type C layers
The close-packed layers in a hcp crystal are stacked in such a way that two types alternate: ABAB . . . or ACAC . . . . The stacking order in a double hexagonal close-packed structure is ABACABAC . . . . Looking back at Fig. 7.12, the stacking order of the close-packed planes is seen to be ABCABC . . . along the [111] direction of the face-centered cubic crystal. The nearest neighbors of a selected atom are shown in Fig. 7.21 for both arrangements. In the fcc structure, the twelve neighbors are on the vertices of a cuboctahedron, while in the hcp structure they are at the vertices of an anticuboctahedron (also called triangular orthobicupola).
One may choose more complicated bases in the hexagonal structure, too. Figure 7.22 shows two such examples. Among two-component compounds of composition AB the NiAs structure or B8 structure is quite common. Atoms
of one kind sit at positions 000 and 00 |
1 |
, while atoms of the other at |
1 2 1 |
and |
|
|
|
2 |
|
3 3 4 |
|
2 1 3 |
. This structure may be regarded as a modification of the double hexag- |
||||
3 3 4 |
|
|
|
|
|

228 |
7 The Structure of Crystals |
|
|
|
|
|
C |
|
A |
|
|
B |
|
B |
|
(a) |
A |
(b) |
A |
|
|
|
Fig. 7.21. The arrangement of the 12 nearest neighbors of an atom in a (a) facecentered cubic; (b) hexagonal close-packed structure
onal close-packed structure, where di erent kinds of atoms sit in alternate layers, in such a way that in spite of the unequal radii relatively close packing is maintained with a stacking order ABAC ABAC . . . .
(a) |
(b) |
Fig. 7.22. Hexagonal crystal structures: (a) NiAs (B8) structure; (b) wurtzite (B4) structure
In addition to its cubic form, zinc sulfide (ZnS) may also crystallize in hexagonal geometry; it is then called wurtzite. In the wurtzite or B4 structure zinc atoms are located at 13 23 0 and 23 13 12 , and sulfur atoms at 13 23 z and 23 13 ( 12 + z), with z ≈ 3/8. Zinc atoms are coordinated tetrahedrally by sulfur atoms, similarly to the cubic ZnS structure, sphalerite, shown in Fig. 7.16; however, the two forms di er in the relative orientation of tetrahedra in subsequent layers. Figure 7.22(b) shows this structure in a displaced coordinate system where a zinc atom is at the origin. Here, too, hexagonal layers of zinc and sulfur atoms alternate, however, the stacking order is now AABB AABB . . . .
Like above, the sixfold rotation axis is replaced by a sixfold screw axis and inversion is no longer a symmetry unless it is followed by a translation. The space group is therefore P 63mc (C64v ).
The hexagonal planes may be stacked in various other ways, leading to even longer periodicities in the structure. Structures with a periodicity of five (ABCAB ABCAB . . . ) or six (ABCACB ABCACB . . . or ABABAC
7.5 Layered and Chain-Like Structures |
229 |
ABABAC . . . ) layers are equally possible. In a particular form of SiC a unit that extends over almost 600 layers is repeated.
The structure of graphite merits separate discussion (Section 7.5). Here we just mention that it can be regarded as four interpenetrating hexagonal Bravais lattices with origins at 000, 13 23 0, 00 21 , and 23 13 12 . This leads to the graphite structure shown in Fig. 7.23(a). In each plane carbon atoms form a honeycomb lattice, however, because of the displacement of subsequent layers only atoms in every second layer are exactly above each other.
7.4 Typical Sizes of Primitive Cells
We have not yet discussed the sizes of primitive cells, i.e., the characteristic distance of periodicity in the crystal. To fill this gap, we have listed the Bravais cell dimensions and nearest-neighbor distances for some of the cubic, tetragonal, and hexagonal structures that we had encountered. In line with common practice, lattice constants are given in angstroms – although using nm and pm units for atomic dimensions is more and more common.
1 Å = 0.1 nm = 100 pm.
As it can be seen in the table, atomic distances are a few angstroms in these simple ordered crystalline structures. As we shall see at the end of this chapter, these distances are often in good agreement with the values determined from atomic or ionic radii assuming close packing.
In more complicated structures the lattice constant may be much larger. The lattice constant of the fcc Bravais cell of fullerite is 14.16 Å at room temperature. The distance between centers of neighboring fullerene (C60) molecules of diameter 7.10 Å is 10.02 Å; within a molecule the distance between two adjacent carbon atoms is 1.46 Å for single and 1.40 Å for double bonds. This is hardly di erent from the C–C distances in the hexagonal rings of graphite.
The order of magnitude of these distances is of great importance since their experimental determination requires methods that work well on such scales. These methods will be presented in the next chapter.
7.5 Layered and Chain-Like Structures
Most of the examples that we have encountered so far are cubic or hexagonal crystals. These types occur most frequently in nature, even though over a hundred other categories are listed in crystallographic tables. Here we shall consider a few special structures that are of particular interest for physicists because of their properties directly related to structure.
In cubic structures atoms are spaced at equal distances in the three spatial directions. In hexagonal and tetragonal structures one direction has a privileged status over the two others, however, atomic spacing along the sixfold

230 7 The Structure of Crystals
Table 7.2. Size of the Bravais cell and nearest-neighbor distance in some common cubic, tetragonal, and hexagonal crystal structures
Name of |
Structure |
Size of the Bravais cell |
Nearest-neighbor |
|||
crystal |
type |
distance |
||||
|
|
|
||||
|
|
|
|
|
|
|
Po |
Ah |
a = 3.366 Å |
|
|
3.366 Å |
|
Ag |
A1 |
a = 4.086 Å |
|
|
2.889 Å |
|
Al |
A1 |
a = 4.049 Å |
|
|
2.863 Å |
|
Au |
A1 |
a = 4.078 Å |
|
|
2.884 Å |
|
Ca |
A1 |
a = 5.588 Å |
|
|
3.951 Å |
|
Cu |
A1 |
a = 3.615 Å |
|
|
2.556 Å |
|
Kr |
A1 |
a = 5.646 Å |
|
|
3.992 Å |
|
Ne |
A1 |
a = 4.462 Å |
|
|
3.155 Å |
|
Ni |
A1 |
a = 3.523 Å |
|
|
2.491 Å |
|
Pd |
A1 |
a = 3.890 Å |
|
|
2.751 Å |
|
Pt |
A1 |
a = 3.924 Å |
|
|
2.775 Å |
|
Cr |
A2 |
a = 2.884 Å |
|
|
2.498 Å |
|
Cs |
A2 |
a = 6.067 Å |
|
|
5.254 Å |
|
Fe |
A2 |
a = 2.867 Å |
|
|
2.483 Å |
|
K |
A2 |
a = 5.321 Å |
|
|
4.608 Å |
|
Na |
A2 |
a = 4.210 Å |
|
|
3.646 Å |
|
W |
A2 |
a = 3.165 Å |
|
|
2.741 Å |
|
Mg |
A3 |
a = 3.209 Å |
c = |
5.210 Å |
3.197 Å |
|
Na |
A3 |
a = 3.767 Å |
c = |
6.154 Å |
3.768 Å |
|
Zn |
A3 |
a = 2.664 Å |
c = |
4.949 Å |
2.665 Å |
|
La |
A3 |
a = 3.770 Å |
c = 12.159 Å |
3.739 Å |
||
C |
A4 |
a = 3.567 Å |
|
|
1.545 Å |
|
Si |
A4 |
a = 5.431 Å |
|
|
2.352 Å |
|
Ge |
A4 |
a = 5.657 Å |
|
|
2.450 Å |
|
C |
A9 |
a = 2.461 Å |
c = |
6.709 Å |
1.426 Å |
|
NaCl |
B1 |
a = 5.640 Å |
|
|
2.820 Å |
|
CsCl |
B2 |
a = 4.113 Å |
|
|
2.908 Å |
|
ZnS |
B3 |
a = 5.411 Å |
|
|
2.343 Å |
|
ZnS |
B4 |
a = 3.822 Å |
c = |
6.261 Å |
2.343 Å |
|
NiAs |
B81 |
a = 3.619 Å |
c = |
5.034 Å |
2.439 Å |
|
CaF2 |
C1 |
a = 5.462 Å |
|
|
2.365 Å |
|
Cu2Mg |
C15 |
a = 7.048 Å |
|
|
2.492 Å |
|
AuCu |
L10 |
a = 3 966 Å |
c = |
3 673 Å |
2.703 Å |
|
Cu3Au |
L12 |
a = 3.748 Å |
|
|
2.651 Å |
|
Mn3Pt |
L12 |
a = 3.833 Å |
|
|
2.710 Å |
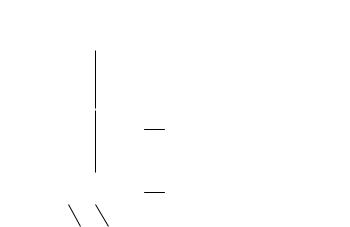
7.5 Layered and Chain-Like Structures |
231 |
or fourfold rotation axis and in the perpendicular directions are of the same order. In some materials much larger discrepancies may arise between inequivalent directions. This may be due to the shape of the molecules making up the crystal – or to the nature of the bonds that determine the structure.
Besides diamond, the lattice constants of another allotrope of carbon, graphite are also listed in Table 7.2. As it can be seen from the illustration of the structure, Fig. 7.23(a), in the plane perpendicular to the sixfold axis carbon atoms form a honeycomb lattice in which each atom is surrounded by three neighbors at a distance of 1.42 Å. The separation between adjacent layers is more than its double, 3.35 Å.
|
|
|
3.78 Å |
|
|
(La, Sr) O |
|
|
|
CuO2 |
|
|
|
(La, Sr) O |
Å |
|
|
13.22 |
|
|
|
(La, Sr) O |
|
|
|
|
|
|
|
3.35Å |
|
(a) |
|
(b) |
|
|
1.42Å |
|
La2 xSrxCuO4 |
|
|
|
Fig. 7.23. The layered structure of (a) graphite; (b) La2−x Srx CuO4
Solid-state physics is more and more concerned with the study of such structures, in which the separation of atoms within a plane is much smaller than the separation between the layers. Figure 7.23(b) shows the primitive cell of another material with layered structure, La2−xSrxCuO4, the first example for high-Tc superconductors. Lattice parameters can be read o from the figure. In this structure, CuO2 units form layers; the other atoms are located between these layers.
Another fairly common situation is that atoms are close together only along one direction, not in a plane. Figure 7.24 shows two structures in which the constituent atoms and molecules of the crystal form chains. The distances between the chains are quite large compared to those within the chains, and thus the bonds between the chains are weak. In an allotrope of selenium – γ-Se, the prototype of A8 structure – the crystal structure is hexagonal with lattice constants a = 4.36 Å and c = 4.95 Å. In the oblique coordinate system suited to hexagonal geometry, Se atoms are at x00, x¯x¯ 13 , and 0x 23 , where

232 7 The Structure of Crystals
x ≈ 0.22. The crystal is invariant under the combined operation of a rotation through 120◦ around the c-axis and a translation through one-third the lattice constant. The space group of the crystal is thus P 3121 (D34). As it is shown in Fig. 7.24(a), the distance between neighboring atoms along the spiral is smaller than the separation of the spirals. The structure is thus similar to a set of twisted chains.
x
z |
y |
z
(a) |
(b) |
Fig. 7.24. (a) The hexagonal A8 structure of γ-Se and its projection on the (001) plane. Atoms marked by •, and ◦ signs are on planes c/3 apart. (b) The projection of the atoms of HMTTF-TCNQ to the (010) and (100) planes
Chain-like structures arise when large flat molecules are stacked relatively tightly and the distance between the chains is larger than the separation of the molecules in them. Figure 7.24(b) shows two projections of the orthorhombic primitive cell of HMTTF-TCNQ – a material showing metallic characteristics in spite of being built up of two organic molecules, HMTTF and TCNQ. The space group is P mna (D27h). The lattice constants of the primitive cell are a = 12.470 Å, b = 3.906 Å, and c = 21.602 Å. HMTTF cations occupy sites 000 and 12 12 12 , while TCNQ anions are found at 12 12 0 and 00 21 . As the figure shows, the molecules are not precisely in the ac-plane but are inclined at 23.8◦ and 34.2◦ with respect to it. Thus in the direction of the b-axis the separation of TCNQ molecules is 3.23 Å, while that of HMTTF molecules is 3.57 Å. These distances are much smaller than intermolecular distances in the ac-plane, thus one is justified to say that HMTTF and TCNQ molecules form chains along the b-axis, and the interaction between the chains is relatively weak.
One possible consequence of the chain-like structure is the almost vanishing overlap of the electron clouds between the chain. In layered structures the