
Multiple Bonds Between Metal Atoms / 15-Extended Metal Atom Chains
.pdf
Extended Metal Atom Chains 699
|
|
|
|
Berry |
|
Table 15.3. Structural data for EMACs of DPyF and related ligands |
|
|
|||
Compound |
Space Group |
M···M |
µeff, µBa |
ref. |
|
[Cr3(DPyF)4](PF6)2·4CH3CN·2Et2O |
P21/c |
1.949(7) |
4.6 |
22 |
|
2.738(7) |
|||||
|
|
|
|
||
[Cu3(DPyF)4](PF6)2·4CH3CN·2Et2O |
P21/c |
2.649(1) |
NR |
61 |
|
2.649(1) |
|||||
|
|
|
|
||
[Co3(DPyF)4](PF6)2·4CH3CN·2Et2O |
P21/c |
2.865(1) |
6.60 |
61 |
|
2.849(1) |
|||||
|
|
|
|
||
[Fe3(DPyF)4](PF6)2·4CH3CN·2Et2O |
P21/c |
2.782(1) |
11.33 |
61 |
|
2.783(1) |
|||||
|
|
|
|
||
[Cu3(DPyF)4](OTf)2·1.5EtOH |
P21/c |
2.6618(8) |
2.6 |
59 |
|
2.6676(8) |
|||||
|
|
|
|
||
[Cu3(DPmF)4](OTf)2·0.5H2O |
– |
2.637(3) |
2.6 |
60 |
|
P1 |
2.625(3) |
||||
|
|
|
|
a NR = not reported.
Also relevant are some chains of CuI ions with N-donor ligands. These include the remarkable trigonal complexes of pentaazadienide ligands62 (shown in 15.23 and Fig. 15.11) among others.63,64
R R
N N
N N N
R R
N N
N N N
R R
N
N
N N N
15.23
Fig. 15.11. Structure of Cu3(TolN5Tol)3.

700Multiple Bonds Between Metal Atoms Chapter 15
The trigonal chain complexes Cu3(TolN5Tol)3 and Cu3(p-EtOPhN5PhOEt)3 feature some of the shortest known Cu···Cu distances: 2.36 and 2.35 Å, respectively. The weak paramagnetism of these compounds is not well understood.62
It is for nickel that the longest discrete EMACs are known. The Nin2n+ compounds with n = 4,8 5,7,65 7,8 and 99 have been synthesized and structurally characterized. Since these contain only d8 Ni(II) ions, no Ni–Ni bonds are present. Oxidation to Ni–Ni bonded Nin(2n+1)+ has only been achieved so far with pentanickel compounds (i.e. n = 5).65,66 There is some evidence to suggest that EMACs with axial NCS ligands adhere to Au (111) surfaces.67
The longer Nin2n+ chains are synthesized by the reaction of NiCl2 or Ni(OAc)2 with the free ligand (those shown in 15.4 as well as H2phdpda for the tetranickel chain and H2etpda, which are shown in 15.24) and KOBut in molten naphthalene.68,69 High temperatures appear to be necessary for the formation of the longer Nin chains. As seen already for the pentacobalt complexes, addition of excess NaX (X = CN, N3, SCN) to the reaction mixtures yields complexes with axial X ligands.68,69
N |
N |
N |
N |
N |
N |
N |
N |
|
|
phdpda |
|
|
|
etpda |
|
15.24
The pentanickel complexes are easier to oxidize than the corresponding trinickel complexes by ~0.4 V.65 Reaction of Ni5(tpda)4Cl2 or Ni5(etpda)4Cl2 with excess Ag+ gives the green oxidized complexes as follows:65,66
Ni5(tpda)4Cl2 + 3AgOTf |
CH2Cl2 |
[Ni5(tpda)4(OTf)2]OTf + 2AgCl + Ag |
|
CH2Cl2
Ni5(etpda)4Cl2 + 3AgPF6 [Ni5(etpda)4](PF6)3 + 2AgCl + Ag
By using AgBF4 in non-rigorously dry conditions the reaction occurs similarly, but the crystallographically characterized product has H2O coordinated to the Ni5 chain.66
The tetranuclear complex Ni4(phdpda)4 is unique among the longer EMACs of nickel because it has no axial ligands and is thus diamagnetic.8 All four of the Ni atoms have similar Ni–N bond lengths, and the average Ni···Ni separations (2.32 Å) are nearly the same, with the outer Ni···Ni separations longer than the inner one by 0.02 Å. The unsymmetrical ligands wrap the tetranickel chain in a cis 2:2 geometry with each ligand pointing the opposite direction from the ligand trans to it.
The Ni510+ EMACs with the tpda2- ligand7,68 and the etpda2- ligand65 have fairly short inner Ni···Ni separations in the range 2.29-2.31 Å. The outer Ni···Ni separations are longer (2.35 - 2.40 Å) due to interactions with the axial ligands. Similar to the trinickel chains, the outer Ni atoms are high spin. Thus, the Ni–N bond distances of the outer Ni atoms are typically ~ 0.2 Å longer than the corresponding distances to the inner Ni atoms.
As seen previously for Ni36+/7+ compounds, oxidation of Ni510+ to Ni511+ leads to major structural changes in the pentanickel chain. The inner Ni–Ni distances in the Ni511+ compounds shorten to between 2.23 and 2.28 Å and the outer Ni–Ni distances shrink to 2.29 - 2.36 Å consistent with fivecenter Ni–Ni bond formation. Attachment of Fe, Ru, Mo and W complexes to pentanickel chains

Extended Metal Atom Chains 701
Berry
through axial cyanide linkages leads to very complex cyclic voltammograms in which it is difficult to assign the waves as being due to oxidation of the pentanickel core or of the axial metal ions.38
For hepta-8,69 and nonanickel9 compounds (an example is shown in Fig. 15.12), few are known and no metal-metal bonded oxidation products have been synthesized yet. The compounds which are known follow trends adumbrated by the triand pentanickel compounds. The inner Ni atoms are square planar and diamagnetic with shorter Ni–N bond lengths (c. 1.92 Å) and shorter Ni···Ni distances in the range of 2.19 to 2.31 Å. The two terminal Ni atoms are high spin with S = 1 and have Ni–N distances ~ 0.2 Å longer than the inner Ni atoms and Ni···Ni distances in the range 2.35 - 2.39 Å.
Fig. 15.12. Structure of Ni9(peptea)4Cl2.
In Nin2n+ compounds with n = 3, 5, 7, and 9, the values of J for the antiferromagnetic coupling of the spins of the terminal Ni atoms of Ni3(dpa)4Cl2, Ni5(tpda)4Cl2, Ni7(teptra)4Cl2, and Ni9(peptea)4Cl2 are -198, -16.6, -7.6, and -3.4 cm-1, respectively.9,‡ These values are proportional to 1/d3, where d is the distance between terminal Ni atoms. The J value for Ni3(dpa)4Cl2, however, was redetermined by another group with the result that J = -218.2(7) cm-1.50 Also, the value of J for Ni5(tpda)4Cl2 given above has been shown to be in error and has been redetermined to be -33.54(4) cm-1.65 Despite the controversy in the derived J values, it is clear from the magnetic data that the trinickel compounds have the most strongly coupled spins, and the coupling appears to decline rapidly as the Nin chain becomes longer.
The magnetic properties of the oxidized Ni5(tpda)43+ complexes have been described and modeled by the view that only one of the two terminal Ni atoms is oxidized to Ni3+ and antiferromagnetic coupling between the terminal high spin Ni2+ (S = 1) and Ni3+ (S = ½) with J = -1110 and -636 cm-1 for [Ni5(tpda)4(H2O)(BF4)](BF4)2 and [Ni5(tpda)4(OTf)2]OTf occurs.66 This view has been contested and evidence for a delocalized Ni511+ unit with S = ½ has been shown for Ni5(etpda)4(PF6)3.65
15.5 EMACs of Ruthenium and Rhodium
The compounds Ru3(dpa)4Cl2 and Rh3(dpa)4Cl2 were described in a short communication lacking many basic experimental details.5 Ru3(dpa)4Cl2 was prepared in 2% yield from Ru2(OAc)4Cl, KOBut, and Hdpa in naphthalene. There is no indication of the preparation of Rh3(dpa)4Cl2 except that it is prepared “similarly.” Both complexes feature symmetrical chains
‡ These J values have all been normalized to accord with the Hamiltonian |
= -JS1·S2. Therefore, values which |
|
were determined in ref. 9 with the Hamiltonian |
= -2JS1·S2 have been multiplied by 2. |
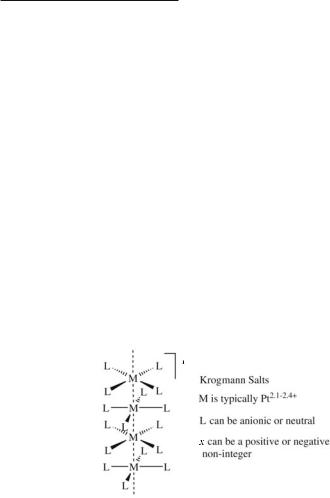
702Multiple Bonds Between Metal Atoms Chapter 15
with M–M distances of 2.25 (Ru3) and 2.39 Å (Rh3) and long M···Cl contacts > 2.5 Å. The Ru36+ complex is reported to be diamagnetic, and its 1H NMR spectrum is reported to consist, oddly, of seven unresolved multiplets which were not assigned. Rh3(dpa)4Cl2 is paramagnetic with one unpaired electron.
15.6 Other Metal Atom Chains
The compounds described above can be viewed as the newest addition to an old family of metal atom chains. The most notable of these are the platinum blues which have been known since 190870 and are briefly reviewed in Section 14.4.7, and Krogman salts (mixed-valence chains, shown schematically in 15.25) which were shown in the 1960s to possess metallic properties in one dimension71 and have been the subject of three monograph texts.72 More recently, face-sharing M3X12n- compounds73 and organometallic complexes with metal atom chains have been reported,74 along with rhodium and iridium blues75 and new rhodium chains.76 Certain aspects of this chemistry are similar to issues discussed above for polypyridylamido complexes and will be discussed briefly here, along with selected examples of chain compounds with metal-metal bonds from the recent literature. Strong d10···d10 interactions in compounds of heavy elements such as gold often give rise to extended chains as well, though these will not be discussed in detail here since formal Au–Au bonding is not involved. A major review by Pyykkö77 summarizes this chemistry rather comprehensively.
15.25
A major impetus for the study of solids with one-dimensional metal atom chains is their ability to conduct electrical current, although the photophysical properties78 and superconductivity79 have also been of interest. The study of conductivity in systems such as Krogmann salts has been intimately related with the band theory of solids which is far too complicated to describe in this monograph, though interested readers may consult some introductory texts.80 In a hypothetical infinite metal atom chain where the metal atoms are evenly spaced and allowed to form bonds to their neighbors, electron delocalization about the metal-based orbitals may be expected to give rise to conductivity in the same way that the / orbitals of polyacetylene81 (i.e. (CH)x) are conducting. But things are not always this straightforward. Peierls showed that in one dimensional systems, a conducting structure with equally spaced metal atoms is often unstable with respect to a phase with alternating long and short metal-metal distances.82 In one dimensional systems, such insulator-conductor phase transitions (also called Peierls transitions) are common as shown in 15.26.

Extended Metal Atom Chains 703
Berry
MM
M M conductor
MM M M insulator
15.26
It is useful here to look back at the Cr510+ and Co510+ compounds described above in connection with the Peierls instability. The issue which must be addressed is the relative stability of the localized M24+ units vs the delocalized M510+ unit. For chromium, the pairing of 8 d electrons to form a Cr24+ quadruple bond appears to favor the Peierls (insulator) state, which tends to agree with the experimental evidence that several unsymmetrical crystal structures of Cr5(tpda)4Cl2 are known with alternating long and short Cr–Cr distances. On the other hand, a Co24+ unit has only a single electron rich bond. In this case, the Co510+ unit appears to be favored, though the possibility of a Peierls phase transition at very low temperatures cannot be ruled out.
Square planar complexes of d8 metals such as Pt(CN)42- often pack to form metal atom chains in the solid state. Partial oxidation of these complexes to form mixed valence chains or Krogmann salts typically results in a one dimensional conducting material.71 Discrete oxidized oligomers of this type with Pt49+ chains are known as platinum blues for their deep blue color.83 This chemistry is similar to the chemistry of Ni36+/7+ and Ni510+/11+ chains discussed earlier. The unoxidized species have only d8 Ni2+ ions and no Ni–Ni bonds, but upon oxidation, Ni–Ni bonds form in the mixed-valence state and the compounds are expected to be conducting.
Similar chemistry is known in the oxidation to Pt38+ of a discrete Pt36+ compound with a linear [Pt(bpy)]3 chain stabilized by the ligand 7-amino-1,8-naphthyridin-2-one (see 15.27a).84 Also, linear Au···Pt···Au compounds with the bridging ylide ligand shown in 15.27b have been oxidized from Au2Pt4+ to Au2Pt6+ whereupon Au–Pt bonds (2.67 Å) form.85 It is worth noting that the corresponding Au2Pb4+ complex does not undergo similar oxidation.86
15.27

704Multiple Bonds Between Metal Atoms Chapter 15
References
1.(a) L.-G. Zhu and S.-M. Peng, Wuji Huaxue Xuebao, 2002, 18, 117. (b) J. K. Bera and K. R. Dunbar,
Angew. Chem., Int. Ed. 2002, 41, 4453.
2.T. J. Hurley and M. A. Robinson, Inorg. Chem. 1968, 7, 33.
3.S. Aduldecha and B. Hathaway, J. Chem. Soc., Dalton Trans. 1991, 993.
4.E.-C. Yang, M.-C. Cheng, M.-S. Tsai and S.-M. Peng, J. Chem. Soc., Chem. Commun. 1994, 20, 2377.
5.J.-T. Sheu, C.-C. Lin, I. Chao, C.-C. Wang and S.-M. Peng, Chem. Commun. 1996, 3, 315.
6.F.-A. Cotton, L. M. Daniels, C. A. Murillo and I. Pascual, J. Am. Chem. Soc. 1997, 119, 10223.
7.S.-J. Shieh, C.-C. Chao, G.-H. Lee, C.-C. Wang and S.-M. Peng, Angew. Chem., Int. Ed. Engl. 1997, 36, 56.
8.S.-Y. Lai, T.-W. Lin, Y.-H. Chen, C.-C. Wang, G.-H. Lee, M.-H. Yang, M.-K. Leung and S.-M. Peng,
J.Am. Chem. Soc. 1999, 121, 250.
9.S.-M. Peng, C.-C. Wang, Y.-L. Jang, Y.-H. Chen, F.-Y. Li, C.-Y. Mou and M.-K. Leung, J. Magn. Magn. Mater. 2000, 209, 80.
10.R. Clérac, F. A. Cotton, L. M. Daniels, K. R. Dunbar, K. Kirschbaum, C. A. Murillo, A. A. Pinkerton,
A.J. Schultz and X. Wang, J. Am. Chem. Soc. 2000, 122, 6226.
11.R. Clérac, F. A. Cotton, L. M. Daniels, K. R. Dunbar, C. A. Murillo and I. Pascual, Inorg. Chem. 2000, 39, 748.
12.F. A. Cotton, L. M. Daniels, T. Lu, C. A. Murillo and X. Wang, J. Chem. Soc., Dalton Trans. 1999, 517.
13.(a) M.-M. Rohmer and M. Bénard, J. Am. Chem. Soc. 1998, 120, 9372. (b) M.-M. Rohmer, A. Strich,
M.Bénard and J.-P. Malrieu, J. Am. Chem. Soc. 2001, 123, 9126.
14.N. Benbellat, M.-M. Rohmer and M. Bénard, Chem. Commun. 2001, 2368.
15.J. F. Berry, F. A. Cotton, L. M. Daniels, C. A. Murillo and X. Wang, Inorg. Chem. 2003, 42, 2418.
16.C.-Y. Yeh, C.-H. Chou, K.-C. Pan, C.-C. Wang, G.-H. Lee, Y.-O. Su and S.-M. Peng, J. Chem. Soc., Dalton Trans. 2002, 2670.
17.Y.-H. Chen, C.-C. Lee, C.-C. Wang, G.-H. Lee, S.-Y. Lai, F.-Y. Li, C.-Y. Mou and S.-M. Peng, Chem. Commun. 1999, 1667.
18.F. A. Cotton, L. M. Daniels, C. A. Murillo and I. Pascual, Inorg. Chem. Commun. 1998, 1, 1.
19.R. Clérac, F. A. Cotton, S. P. Jeffery, C. A. Murillo and X. Wang, Dalton Trans. 2003, 3022.
20.R. Clérac, F. A. Cotton, L. M. Daniels, K. R. Dunbar, C. A. Murillo and H.-C. Zhou, Inorg. Chem. 2000, 39, 3414.
21.F. A. Cotton, L. M. Daniels, P. Lei, C. A. Murillo and X. Wang, Inorg. Chem. 2001, 40, 2778.
22.F. A. Cotton, L. M. Daniels, C. A. Murillo and X. Wang, Chem. Commun. 1998, 39.
23.F. A. Cotton, P. Lei, C. A. Murillo and L.-S. Wang, Inorg. Chim. Acta 2003, 349, 165.
24.R. Clérac, F. A. Cotton, L. M. Daniels, K. R. Dunbar, C. A. Murillo and I. Pascual, Inorg. Chem. 2000, 39, 752.
25.J. F. Berry, F. A. Cotton, C. A. Murillo and B. K. Roberts, Inorg. Chem. 2004, 43, 2277.
26.J. F. Berry, F. A. Cotton, T. Lu, C. A. Murillo, B. K. Roberts and X. Wang, J. Am. Chem. Soc. 2004, 126, 7082.
27.F. A. Cotton, P. Lei and C. A. Murillo, Inorg. Chim. Acta 2003, 349, 173.
28.M.-M. Rohmer and M. Bénard, J. Cluster Sci. 2002, 13, 333.
29.H.-C. Chang, J.-T. Li, C.-C. Wang, T.-W. Lin, H.-C. Lee, G.-H. Lee and S.-M. Peng, Eur. J. Inorg. Chem. 1999, 1243.
30.F. A. Cotton, L. M. Daniels, C. A. Murillo and X. Wang, Chem. Commun. 1999, 2461.
31.F. A. Cotton, L. M. Daniels and G. T. Jordan, IV, Chem. Commun. 1997, 421.
32.F. A. Cotton, L. M. Daniels, G. T. Jordan, IV and C. A. Murillo, J. Am. Chem. Soc. 1997, 119, 10377.
33.R. Clérac, F. A. Cotton, K. R. Dunbar, T. Lu, C. A. Murillo and X. Wang, Inorg. Chem. 2000, 39, 3065.

Extended Metal Atom Chains 705
Berry
34.R. Clérac, F. A. Cotton, L. M. Daniels, K. R. Dunbar, C. A. Murillo and X. Wang, J. Chem. Soc., Dalton Trans. 2001, 386.
35.R. Clérac, F. A. Cotton, S. P. Jeffery, C. A. Murillo and X. Wang, Inorg. Chem. 2001, 40, 1265.
36.J. F. Berry, F. A. Cotton, T. Lu and C. A. Murillo, Inorg. Chem. 2003, 42, 4425.
37.R. Clérac, F. A. Cotton, K. R. Dunbar, T. Lu, C. A. Murillo and X. Wang, J. Am. Chem. Soc. 2000, 122, 2272.
38.T. Sheng, R. Appelt, V. Comte and H. Vahrenkamp, Eur. J. Inorg. Chem. 2003, 3731.
39.F. A. Cotton, C. A. Murillo and X. Wang, J. Chem. Soc., Dalton Trans. 1999, 3327.
40.R. Clérac, F. A. Cotton, L. M. Daniels, K. R. Dunbar, C. A. Murillo and X. Wang, Inorg. Chem. 2001, 40, 1256.
41.M.-M. Rohmer and M. Bénard, Chem. Soc. Rev. 2001, 30, 340.
42.F. A. Cotton, C. A. Murillo and X. Wang, Inorg. Chem. 1999, 38, 6294.
43.C.-Y. Yeh, C.-H. Chou, K.-C. Pan, C.-C. Wang, G.-H. Lee, Y.-O. Su and S.-M. Peng, J. Chem. Soc., Dalton Trans. 2002, 2670.
44.J. F. Berry, F. A. Cotton, L. M. Daniels and C. A. Murillo, J. Am. Chem. Soc. 2002, 124, 3212.
45.L.-P. Wu, P. Field, T. Morrissey, C. Murphy, P. Nagle, B. Hathaway, C. Simmons and P. Thornton,
J.Chem. Soc., Dalton Trans. 1990, 3853.
46.G. J. Pyrka, M. El-Mekki and A. A. Pinkerton, J. Chem. Soc., Chem. Commun. 1991, 84.
47.R. Clérac, F. A. Cotton, K. R. Dunbar, C. A. Murillo, I. Pascual and X. Wang, Inorg. Chem. 1999, 38, 2655.
48.T.-B. Tsao, G.-H. Lee, C.-Y. Yeh and S.-M. Peng, Dalton Trans. 2003, 1465.
49.J. F. Berry, F. A. Cotton, P. Lei and C. A. Murillo, Inorg. Chem. 2003, 42, 377.
50.J. F. Berry, F. A. Cotton, T. Lu, C. A. Murillo and X. Wang, Inorg. Chem. 2003, 42, 3595.
51.F. A. Cotton, P. Lei and C. A. Murillo, Inorg. Chim. Acta 2003, 351, 183.
52.L.-G. Zhu, S.-M. Peng and G.-H. Lee, Anal. Sci. 2002, 18, 1067.
53.C.-H. Peng, C.-C. Wang, H.-C. Lee, W.-C. Lo, G.-H. Lee and S.-M. Peng, J. Chin. Chem. Soc. (Taipei) 2001, 48, 987.
54.J. F. Berry, F. A. Cotton and C. A. Murillo, Dalton Trans. 2003, 3015.
55.L.-G. Zhu, S.-M. Peng and G.-H. Lee, Chem. Lett. 2002, 1210.
56.H. Li, G.-H. Lee and S.-M. Peng, Inorg. Chem. Commun. 2003, 6, 1.
57.O. Kahn, Molecular Magnetism, Wiley-VCH, Inc.: New York, 1993.
58.(a) M. Gerloch and J. H. Harding, Proc. R. Soc. London 1978, A 360, 211. (b) M. Kato and Y. Muto,
Coord. Chem. Rev. 1988, 92, 45.
59.G. A. van Albada, I. Mutikainen, U. Turpeinen and J. Reedjik, Eur. J. Inorg. Chem. 1998, 547.
60.G. A. van Albada, P. J. van Koningsbruggen, I. Mutikainen, U. Turpeinen and J. Reedjik, Eur.
J.Inorg. Chem. 1999, 2269.
61.F. A. Cotton, C. A. Murillo and X. Wang, Inorg. Chem. Commun. 1998, 1, 281.
62.(a) J. Beck and J. Strähle, Angew. Chem., Int. Ed. Engl. 1985, 24, 409. (b) R. Schmid and J. Strähle,
Z.Naturforsch. 1989, 446, 105.
63.M.-S. Tsai and S.-M. Peng, J. Chem. Soc., Chem. Commun. 1991, 514.
64.R. Clérac, F. A. Cotton, L. M. Daniels, J. Gu, C. A. Murillo and H.-C. Zhou, Inorg. Chem. 2000, 39, 4488.
65.J. F. Berry, F. A. Cotton, P. Lei, T. Lu and C. A. Murillo, Inorg. Chem. 2003, 42, 3534.
66.C.-Y. Yeh, Y.-L. Chiang, G.-H. Lee and S.-M. Peng, Inorg. Chem. 2002, 41, 4096.
67.S.-Y. Lin, I.-W. P. Chen, C.-h. Chen, M.-H. Hsieh, C.-Y. Yeh, T.-W. Lin, Y.-H. Chen and S.-M. Peng, J. Phys. Chem. B 2004, 108, 959.
68.C.-C. Wang, W.-C. Lo, C.-C. Chou, G.-H. Lee, J.-M. Chen and S.-M. Peng, Inorg. Chem. 1998, 37, 4059.
69.S.-Y. Lai, C.-C. Wang, Y.-H. Chen, C.-C. Lee, Y.-H. Liu and S.-M. Peng, J. Chin. Chem. Soc. (Taipei) 1999, 46, 477.
70.K. A. Hofmann and G. Bugge, Ber. Dtch. Chem. Ges. 1908, 41, 312.

706Multiple Bonds Between Metal Atoms Chapter 15
71.(a) K. Krogmann and P. Dodel, Chem. Ber. 1966, 99, 3402. (b) K. Krogmann and P. Dodel, Chem. Ber. 1966, 99, 3408. (c) K. Krogmann, Z. anorg. allg. Chem. 1968, 358, 97. (d) K. Krogmann,
Angew. Chem., Int. Ed. Engl. 1969, 8, 35.
72.(a) Extended Linear Chain Compounds Miller, J. S., Ed. Vol. 1, Plenum Press: New York, 1982. (b) Extended Linear Chain Compounds Miller, J. S., Ed. Vol. 2, Plenum Press: New York, 1982. (c) Extended Linear Chain Compounds Miller, J. S., Ed. Vol. 3, Plenum Press: New York, 1983.
73.(a) J. C. Fettinger, S. P. Mattamana, C. J. O’Connor, R. Poli and G. Salem, J. Chem. Soc., Chem. Commun. 1995, 1265. (b) F. A. Cotton, M. Matusz and R. C. Torralba, Inorg. Chem. 1989, 28, 1516. (c)
F.A. Cotton and R. C. Torralba, Inorg. Chem. 1991, 30, 2196. (d) F. A. Cotton and R. C. Torralba, Inorg. Chem. 1991, 30, 4386. (e) F. A. Cotton and R. C. Torralba, Inorg. Chem. 1991, 30, 4386.
74.(a) T. Murahashi, E. Mochizuki, Y. Kai and H. Kurosawa, J. Am. Chem. Soc. 1999, 121, 10660.
(b)T. Murahashi, Y. Higuchi, T. Katoh and H. Kurosawa, J. Am. Chem. Soc. 2002, 124, 14288.
(c)T. Murahashi and H. Kurosawa, Coord. Chem. Rev. 2002, 231, 207. (d) T. Murahashi, T. Uemura and H. Kurosawa, J. Am. Chem. Soc. 2003, 125, 8536. (e) M.-D. Su, H.-Y. Liao, S.-Y. Chu, Y. Chi, C.-S. Liu, F.-J. Lee, S.-M. Peng and G.-H. Lee, Organometallics 2000, 19, 5400. (f) F. A. Cotton,
E.V. Dikarev and M. A. Petrukhina, J. Organomet. Chem. 2000, 596, 130. (g) F. A. Cotton,
E.V. Dikarev and M. A. Petrukhina, J. Chem. Soc., Dalton Trans. 2000, 4241. (h) T. Murahashi,
S.Ogashi and H. Kurosawa, Chem. Record 2003, 3, 101.
75.C. Tejel, M. A. Ciriano and L. A. Oro, Chem. Eur. J. 1999, 5, 1131.
76.(a) G. M. Finniss, E. Canadell, C. Campana and K. R. Dunbar, Angew. Chem., Int. Ed. Engl. 1996, 35, 2772. (b) F. P. Pruchnik, P. Jakimowicz, Z. Ciunik, K. Stanislawek, L. A. Oro, C. Tejel and
M.A. Ciriano, Inorg. Chem. Commun. 2001, 4, 19. (c) K. R. Mann, M. J. DiPierro and T. P. Gill, J. Am. Chem. Soc. 1980, 102, 3965.
77.P. Pyykkö, Chem. Rev. 1997, 97, 597.
78.(a) V. M. Miskowski and V. H. Houlding, Inorg. Chem. 1991, 30, 4446. (b) J. W. Brill,
M.Mégnamisi-Bélombé and M. Novotny, J. Chem. Phys. 1978, 68, 585. (c) A. Lechner and
G.Gliemann, J. Am. Chem. Soc. 1989, 111, 7469.
79.S. T. Carr and A. M. Tsvelik, Phys. Rev. B 2002, 65, 195121/1.
80.(a) I. Boz˘ovi´c and J. Delhalle, Phys. Rev. B 1984, 29, 4733. (b) J. S. Miller and A. J. Epstein, Prog. Inorg. Chem. 1976, 20, 1.
81.(a) A. J. Heeger, Rev. Mod. Phys. 2001, 73, 681. (b) A. G. MacDiarmid, Rev. Mod. Phys. 2001, 73, 701. (c) H. Shirakawa, Rev. Mod. Phys. 2001, 73, 713.
82.R. E. Peierls, Quantum Theory of Solids, Oxford University Press: Oxford, 1955, p. 108.
83.S. J. Lippard, Science 1982, 218, 1075.
84.B. Osuki and W. S. Sheldrick, Eur. J. Inorg. Chem. 1999, 1325.
85.(a) H. H. Murray, D. A. Briggs, G. Garzón, R. G. Raptis, L. C. Porter and J. P. Fackler, Jr., Organometallics 1987, 6, 1992. (b) J. P. Fackler, Jr. Inorg. Chem. 2002, 41, 6959.
86.S. Wang, G. Garzón, C. King, J.-C. Wang and J. P. Fackler, Jr., Inorg. Chem. 1989, 28, 4623.