
pvt
.pdf
PVT Analysis
Figure 3: Schematic of Ethane Molecule
The common shorthand representation is CH3CH3 or C2H6: on PVT-reports it will be denoted C2.
A Methane molecule can lose two electrons to generate a CH2 radical. Now if the Ethane C-C bond is broken, the CH2 radical can be inserted between the 2 Methyl radicals and the Propane molecule is created which is represented by:
Figure 4: Schematic of Propane Molecule
The common shorthand representation is CH3 CH2CH3 or C3H8: on PVT-reports it will be denoted C3.
The process just described of inserting CH2 radicals can now be repeated ad-infinitum. The next few molecules in the series are Butane [C4H10 or C4], Pentane [C5H12 or C5]
and Hexane [C6H14 or C6]. The generic formula for this series is CNH2N+2 where N is number of Carbon atoms.
Organic molecules that have a similar structure and consequently graded physical properties are known as a homologous series. This series is variously referred to as the Alkanes or Paraffins. Some physical properties of the normal-Alkanes are shown in Table 2.1 and its corresponding Chart.
Note the melting points of Methane and Ethane does not fit the trend; otherwise, a remarkably smooth set of trends is evident. The first four Alkanes are gases at room conditions: Alkanes with 18 Carbons or more are solids at room conditions.
Whether hydrocarbon molecules are found in gas, liquid or solid states depend on the inter-molecular force called the van der Waals force. Fluctuations in the distribution of the electron clouds gives rise to an electric field, which is the basis for the force. The smallest molecules are highly symmetric and hence the generated fields are weak: characteristics of a gas. The larger molecules are less symmetric and have a stronger field: characteristic of a liquid or solid.
Roxar Oxford |
11 |
12/12/12 |

PVT Analysis
|
|
Boil.Point |
Melt.Point |
Spec.Grav. |
N |
Name |
oF |
oF |
60o/60o |
1 |
Methane |
-258.7 |
-269.4 |
|
2 |
Ethane |
-127.5 |
-297.0 |
|
3 |
Propane |
-43.7 |
-305.7 |
0.507 |
4 |
Butane |
31.1 |
-217.1 |
0.584 |
5 |
Pentane |
96.9 |
-201.5 |
0.631 |
6 |
Hexane |
155.7 |
-139.6 |
0.664 |
7 |
Heptane |
209.2 |
-131.1 |
0.688 |
8 |
Octane |
258.2 |
-70.2 |
0.707 |
9 |
Nonane |
303.5 |
-64.3 |
0.722 |
10 |
Decane |
345.5 |
-21.4 |
0.734 |
11 |
Undecane |
384.6 |
-15.0 |
0.740 |
12 |
Dodecane |
421.3 |
14.0 |
0.749 |
15 |
Pentadecane |
519.1 |
50.0 |
0.769 |
20 |
Eicosane |
648.9 |
99.0 |
|
30 |
Triacontane |
835.5 |
151.0 |
|
Table/Chart 2.1: Physical Properties of Normal-Alkanes
|
|
|
|
|
Variation of Physical Properties with Carbon Number |
|
|
|
|
|||||
|
500.0 |
|
|
|
|
|
|
|
|
|
|
0.900 |
|
|
|
400.0 |
|
|
|
|
|
|
|
|
|
|
0.850 |
|
|
|
|
|
|
|
|
|
|
|
|
|
|
|
|
|
|
300.0 |
|
|
|
|
|
|
|
|
|
|
0.800 |
|
|
|
|
|
|
|
|
|
|
|
|
|
|
|
|
|
|
200.0 |
|
|
|
|
|
|
|
|
|
|
0.750 |
|
|
Temperature/[degF] |
|
|
|
|
|
|
|
|
|
|
|
GravitySpecific |
|
|
|
|
|
|
|
|
|
|
|
|
|
0.700 |
|
||
|
|
|
|
|
|
|
|
|
|
|
|
|
|
|
|
100.0 |
|
|
|
|
|
|
|
|
|
|
|
|
Boil.Point |
|
|
|
|
|
|
|
|
|
|
|
|
|
|
|
|
|
|
|
|
|
|
|
|
|
|
|
0.650 |
|
Melt.Point |
|
0.0 |
|
|
|
|
|
|
|
|
|
|
|
|
Spec.Grav. |
|
|
|
|
|
|
|
|
|
|
|
|
|
|
|
|
|
|
|
|
|
|
|
|
|
|
|
0.600 |
|
|
|
-100.0 |
|
|
|
|
|
|
|
|
|
|
0.550 |
|
|
|
|
|
|
|
|
|
|
|
|
|
|
|
|
|
|
-200.0 |
|
|
|
|
|
|
|
|
|
|
0.500 |
|
|
|
|
|
|
|
|
|
|
|
|
|
|
|
|
|
|
-300.0 |
|
|
|
|
|
|
|
|
|
|
0.450 |
|
|
|
|
|
|
|
|
|
|
|
|
|
|
|
|
|
|
-400.0 |
|
|
|
|
|
|
|
|
|
|
0.400 |
|
|
|
1 |
2 |
3 |
4 |
5 |
6 |
7 |
8 |
9 |
10 |
11 |
12 |
|
|
|
|
|
|
|
|
Carbon Number |
|
|
|
|
|
|
|
Roxar Oxford |
12 |
12/12/12 |

PVT Analysis
2.2.1 Isomerism
The structure of the Alkanes from C4 and above can vary from that implied above. In Figure 3 showing Propane, one of the Hydrogen atoms bonded to the central Carbon atom can be removed and replaced by a Methyl radical: this is known as iso-Butane or iC4.
It has the same number of Carbon’s and Hydrogen’s as its straight-chained equivalent that is generally known as normal-Butane or nC4 [or just C4].
Figure 5: Schematic Representations of Butane Isomers, nC4 and iC4.
Three possible structures are possible for Pentane. The straight-chained molecule called normal-Pentane, nC5 [or just C5]. A Butane chain with a Methyl radical attached to the 2nd Carbon called iso-Pentane, iC5. Finally, a Propane chain with two Methyl radicals attached to the central Carbon: the last structure called neo-Pentane is rarely found in petroleum mixtures.
Figure 6: Schematic Representation of Pentane Isomers.
As the Carbon number rises, the number of Isomers increases rapidly.
Question:
How many isomers are there of Hexane [C6]? Estimate the number of isomers of Decane [C10] and Triacontane [C30].
Branch-chained Isomers do not exhibit the smooth variation in physical properties seen for the normal-Alkanes [see Table/Chart 2.1]. The physical properties of the Hexane Isomers are shown in Table 2.2, below.
Roxar Oxford |
13 |
12/12/12 |
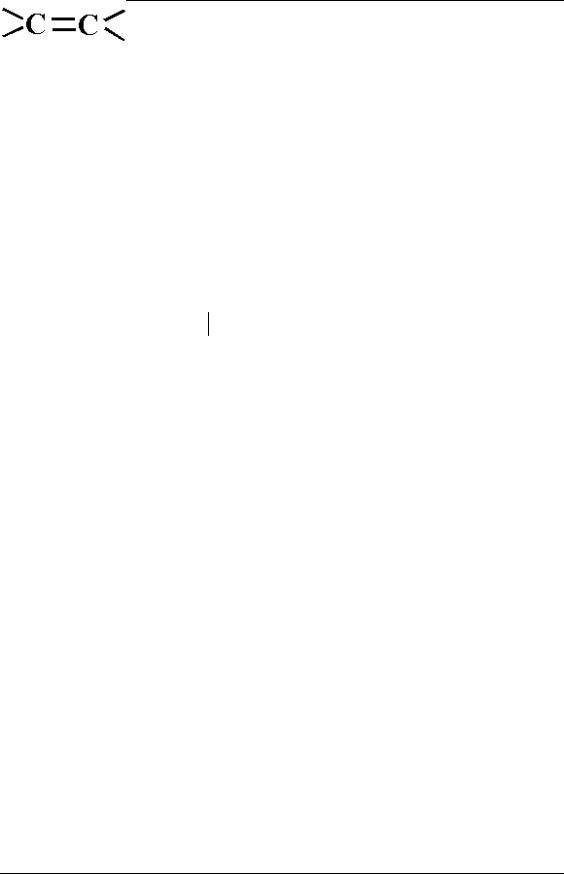
PVT Analysis
Isomer |
|
|
Structure |
Boil.Point |
Melt.Point |
Spec.Grav. |
||||||
|
|
|
|
|
|
|
|
|
|
oF |
oF |
60o/60o |
n-Hexane |
CH3CH2CH2CH2CH2CH3 |
155.7 |
-139.6 |
0.664 |
||||||||
|
|
|
|
CH3 |
|
|
|
|||||
3-MethylPentane |
CH3CH2 |
|
CHCH2CH3 |
145.9 |
-180.4 |
0.669 |
||||||
|
||||||||||||
|
CH3 |
|
|
|
||||||||
2-MethylPentane |
CH3 |
|
CHCH2CH2CH3 |
140.5 |
-244.6 |
0.658 |
||||||
|
||||||||||||
|
|
|
CH3 CH3 |
|
|
|
||||||
2,3-DiMethylButane |
|
|
|
|
|
|
|
|
|
|
|
|
CH3CH |
|
|
|
|
CHCH3 |
136.4 |
-199.4 |
0.666 |
||||
|
||||||||||||
|
|
|
CH3 |
|
|
|
||||||
2,2-DiMethylButane |
CH3CCH2CH3 |
121.5 |
-147.7 |
0.654 |
||||||||
|
|
|
|
|
|
|
|
|||||
|
|
|
CH3 |
|
|
|
Table 2.2: Physical Properties of Hexane Isomers
Generally, an increase in the degree of branching causes a decrease in the inter-molecular attraction with the consequent lowering in boiling point. The variation of melting point is harder to predict. The way the different shapes can be slotted together is the main factor affecting the formation of the solid lattice.
2.2.2 Alkenes and Alkynes
Chemically the Alkanes are unreactive: the name Paraffin means not enough affinity. This is not true of straight-chained and branched hydrocarbons with double bonds – the Alkenes: note each Carbon makes two conventional single bonds.
Figure 7: Schematic of the Alkene Double Bond.
Nor is it the case for triple bounds – the Alkynes: note each Carbon can make only one conventional single bond.
Roxar Oxford |
14 |
12/12/12 |

PVT Analysis
Figure 8: Schematic of the Alkyne Triple Bond.
These bonds are somewhat stretched compared with the equivalent single bond making them much more likely to break. Therefore, these compounds are rarely found in naturally occurring petroleum. That is not to ignore their importance in the Petrochemical industry. In particular, the polymerization6 of Ethene, C2H4, [or to give it its old name of Ethylene] gives rise to that most versatile of materials – polyethylene. Similarly, polymerizing Ethyl Chloride gives PVC – poly-vinyl-chloride.
The presence of a Carbon-Carbon double bond in the Alkenes eliminates the need for two Hydrogen atoms giving a generic formula of CNH2N. The corresponding Alkyne has the formula CNH2N-2.
2.3 Cycloalkanes
The Alkanes [Alkenes and Alkynes] are all straight or branched chains; with 3 or more Carbon atoms, other structures are possible. One of these alternatives is the homologous series called the Cycloalkanes. In the petroleum industry, the names Cycloparaffins or Napthenes are often used instead.
Although they have the same general formula as the Alkenes, CNH2N, because they have a ring structure, their physical properties are very different. The two most common Cycloalkanes are Cyclopentane, C5H10, and Cyclohexane, C6H12: see figures below.
The lighter Cycloparaffins of Cyclopropane, C3H6, and Cyclobutane, C4H8, are both possible. However, they rarely occur in natural petroleum. The Carbon-Carbon bond angles of 60o and 90o are both very sharp making these bonds much weaker than their equivalents in the 5- and 6-Carbon ring structures. Ring structures with 7 or more Carbon atoms are chemically stable but again occur rarely in natural petroleum. This is probably because the probability a straight-chained molecule of 7 or more Carbons would lose a Hydrogen atom from both ends simultaneously is very low.
6 In the case of polymerization of Ethene, one of the C=C double bonds is broken in each molecule. The second Carbon in the first ethane-radical then bonds with the first Carbon in the second ethane-radical, etc. to form the long-chain polyethylene.
The Polymerization Process of Ethene.
Roxar Oxford |
15 |
12/12/12 |

PVT Analysis
Figure 9: Schematic Representation of Cyclopentane and Cyclohexane.
As with the Paraffins, one or more Hydrogen atoms can be removed from any point on the ring to be replaced by Methyl, Ethyl, etc. radicals. Unlike the next series, the Aromatics, the central ring is referred to as saturated.
2.4 Aromatics
A third main homologous series are the Aromatics. The basis for the Aromatics is the Benzene molecule. Benzene contains six Carbons in a hexagonal ring with one Hydrogen atom attached to each Carbon. Initially it was thought that there were three single Carbon-Carbon bonds alternating with 3 double Carbon-Carbon bonds. However, the double bonds would be much weaker than their single bond equivalents making Benzene chemically reactive which is not the case. Clearly, the Carbon-Carbon bonds in Benzene are unlike anything considered to date.
Current thinking has it that the electrons are delocalized over all six Carbon atoms thus there are six hybrid bonds, or one-and-half bonds or benzene bonds.
The common symbols used to depict the Benzene molecule are shown below:
Figure 10: Alternate Schematic Representations for Benzene Molecule
In the figure above, it is assumed there is a single CH group at each vertex of the hexagon. As with the Cycloalkanes, each of the Hydrogen atoms can be replaced by a Methyl, Ethyl, etc. radical. Replacing one Hydrogen atom by a single Methyl radical produces Toluene, C6H5.CH3. Replacing two Hydrogen atoms by Methyl radicals produces Xylene, C6H4.CH3.CH3.
Roxar Oxford |
16 |
12/12/12 |

PVT Analysis
2.5 Polyaromatics
Most of the large molecules found in petroleum are linked multi-ring structures that are composed of Cycloalkanes and Aromatics, often with Sulphur, Oxygen and/or Nitrogen atoms replacing one or more Hydrogen atoms. They are often sub-divided into two classes called Resins and Asphaltenes.
Resins readily dissolve in petroleum and are either heavy liquids or sticky solids. Asphaltenes are solids that are only weakly soluble in petroleum. An oil with a high Asphaltene content is a production nightmare since when the near well bore pressure drops, these molecules will precipitate, causing pore blocking and leading to a loss of Productivity Index (PI).
2.6 Other Compounds
The four main non-hydrocarbon or inorganics components of naturally produced petroleum are:
|
Nitrogen |
N2 |
|
Carbon Dioxide |
CO2 |
|
Hydrogen Sulphide |
H2S |
|
Water |
H2O |
Of these, it is generally assumed that water is mutually insoluble in hydrocarbon phases: this may not be true at high temperatures or in the presence of large concentrations of CO2 and/or H2S. However, we will not consider the effect of water other than as a standalone component during this course.
N2, CO2 and H2S are important constituents of most petroleum mixtures and are routinely considered when laboratory analyses of reservoir fluids are undertaken. Chemically, N2 behaves most closely to Methane. CO2 is most similar to Ethane and H2S to Propane.
2.7 Single Carbon Number Groups
If the presence of Isomers were not bad enough, we now have to contend with the different homologous series. Laboratory analysts could spend between now and eternity trying to isolate every different molecular species. Clearly an impossible task!
For petroleum engineering purposes, standard practice is to check for and measure the concentrations of the inorganics, N2, CO2 and H2S and the first few members of the Alkane series, C1, C2, C3, iC4, nC4, iC5 and nC5. Thereafter, boiling point cuts called Single Carbon Number (SCN) groups are used.
Roxar Oxford |
17 |
12/12/12 |

PVT Analysis
SCN group N is taken to be all hydrocarbon molecules which boil at temperatures just above that of the normal paraffin CN-1 up to and including the normal paraffin CN. For example, consider some of the members of SCN group 7, denoted C7:
Name Tboil/Kelvin normal-Hexane 341.9 Benzene 353.2 Cyclohexane 353.8 2-Methylhexane 363.2 normal-Heptane 371.6
Table 2.3: Some Members of SCN group C7 – Shaded Area.
Clearly, the blend of Paraffins, Paraffin-Isomers, Cycloparaffins and Aromatics within any given SCN group will vary from fluid to fluid. As the blend varies from highly Paraffinic to highly aromatic, the average physical properties of the group can vary considerably. This can in turn, have a considerable effect on the ability of our models to predict fluid behaviour. We will reconsider this issue when we look at fluid characterization and regression. For now, we either take some average of a reasonably large set of test fluids or just use the paraffin properties.
2.7.1 Generalized SCN Physical Properties
The following table is re-produced from Whitson. Note the mole weights of the SCN components are less than the mole weights of the normal-Paraffins with the same number of Carbon atoms. This reflects the presence of Napthanic and Aromatic components within the blend.
Key: Tb |
Normal Boiling Point Temperature |
Specific Gravity (60/60)
Kw |
Watson Characterization Factor: see section 7.2 |
Mw |
Molecular Weight |
Tc |
Critical Temperature |
Pc |
Critical Pressure |
Acentric Factor
Roxar Oxford |
18 |
12/12/12 |
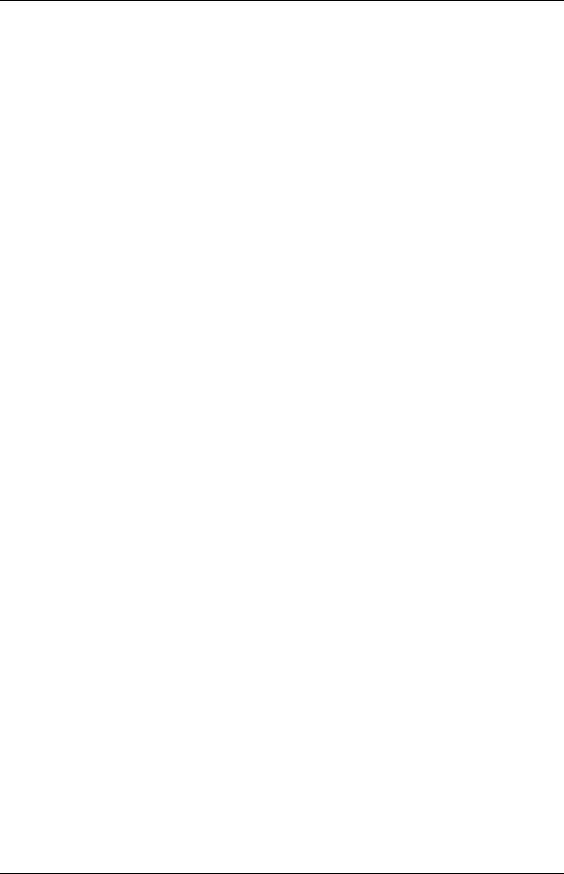
PVT Analysis
|
Tb |
|
|
Kw |
Mw |
Tc |
|
Pc |
|
|
|||
SCN |
|
|
|
|
|
|
|
|
|
|
|
|
|
K |
|
oR |
|
|
|
K |
|
oR |
kPa |
|
psia |
|
|
6 |
337 |
|
607 |
0.690 |
12.27 |
84 |
512 |
|
923 |
3340 |
|
483 |
0.250 |
7 |
366 |
|
658 |
0.727 |
11.96 |
96 |
548 |
|
985 |
3110 |
|
453 |
0.280 |
8 |
390 |
|
702 |
0.749 |
11.87 |
107 |
575 |
|
1036 |
2880 |
|
419 |
0.312 |
9 |
416 |
|
748 |
0.768 |
11.82 |
121 |
603 |
|
1085 |
2630 |
|
383 |
0.348 |
10 |
439 |
|
791 |
0.782 |
11.83 |
134 |
626 |
|
1128 |
2420 |
|
351 |
0.385 |
11 |
461 |
|
829 |
0.793 |
11.85 |
147 |
648 |
|
1166 |
2230 |
|
325 |
0.419 |
12 |
482 |
|
867 |
0.804 |
11.86 |
161 |
668 |
|
1203 |
2080 |
|
302 |
0.454 |
13 |
501 |
|
901 |
0.815 |
11.85 |
175 |
687 |
|
1236 |
1960 |
|
286 |
0.484 |
14 |
520 |
|
936 |
0.826 |
11.84 |
190 |
706 |
|
1270 |
1860 |
|
270 |
0.516 |
15 |
539 |
|
971 |
0.836 |
11.84 |
206 |
724 |
|
1304 |
1760 |
|
255 |
0.550 |
16 |
557 |
|
1002 |
0.843 |
11.87 |
222 |
740 |
|
1332 |
1660 |
|
241 |
0.582 |
17 |
573 |
|
1032 |
0.851 |
11.87 |
237 |
756 |
|
1360 |
1590 |
|
230 |
0.613 |
18 |
586 |
|
1055 |
0.856 |
11.89 |
251 |
767 |
|
1380 |
1530 |
|
222 |
0.638 |
19 |
598 |
|
1077 |
0.861 |
11.91 |
263 |
778 |
|
1400 |
1480 |
|
214 |
0.662 |
20 |
612 |
|
1101 |
0.866 |
11.92 |
275 |
790 |
|
1421 |
1420 |
|
207 |
0.690 |
21 |
634 |
|
1124 |
0.871 |
11.94 |
291 |
801 |
|
1442 |
1380 |
|
200 |
0.717 |
22 |
637 |
|
1146 |
0.876 |
11.95 |
300 |
812 |
|
1461 |
1330 |
|
193 |
0.743 |
23 |
648 |
|
1167 |
0.881 |
11.95 |
312 |
822 |
|
1480 |
1300 |
|
188 |
0.768 |
24 |
659 |
|
1187 |
0.885 |
11.96 |
324 |
832 |
|
1497 |
1260 |
|
182 |
0.793 |
25 |
671 |
|
1207 |
0.888 |
11.99 |
337 |
842 |
|
1515 |
1220 |
|
177 |
0.819 |
26 |
681 |
|
1226 |
0.892 |
12.00 |
349 |
850 |
|
1531 |
1190 |
|
173 |
0.844 |
27 |
691 |
|
1244 |
0.896 |
12.00 |
360 |
859 |
|
1547 |
1160 |
|
169 |
0.868 |
28 |
701 |
|
1262 |
0.899 |
12.02 |
372 |
867 |
|
1562 |
1130 |
|
165 |
0.894 |
29 |
709 |
|
1277 |
0.902 |
12.03 |
382 |
874 |
|
1574 |
1110 |
|
161 |
0.915 |
30 |
719 |
|
1294 |
0.905 |
12.04 |
394 |
882 |
|
1589 |
1090 |
|
158 |
0.941 |
|
|
|
|
|
|
|
|
|
|
|
|
|
|
2.8 The Plus Fraction
Depending on the nature of the fluid, there comes a point in the allocation of SCN groups where the law of diminishing returns takes over. That is, the error associated with measuring the concentration of SCN group N is bigger than that concentration. At some point before that, which usually depends on how much the owner of the fluid is prepared to pay the service laboratory, a cut-off in the analysis is made. The residual or plus
Roxar Oxford |
19 |
12/12/12 |

PVT Analysis
fraction is what is left. Usually, just the molecular weight7 and sometimes the specific gravity of this fraction are measured are reported. As laboratory techniques have improved, so the typical Carbon number of the plus fraction has increased. In the 1960’s, a plus fraction of C7+ was typical. During the 1970’s and 1980’s, C12+ was typical whereas C20+ would be the norm now. Some typical reservoir fluid analyses are shown below. Note the units are mole fractions. Moles and mole fractions will be discussed in some detail in section 5.1.
Comp |
NS GC |
|
Bah-GC |
|
NS-VO |
|
SWT-BO |
N2 |
0.60 |
|
11.71 |
|
0.58 |
|
0.00 |
CO2 |
3.34 |
|
6.50 |
|
3.27 |
|
0.00 |
H2S |
0.00 |
|
0.05 |
|
0.00 |
|
0.00 |
C1 |
74.16 |
|
79.06 |
|
53.89 |
|
52.00 |
C2 |
7.90 |
|
1.62 |
|
8.57 |
|
3.81 |
C3 |
4.15 |
|
0.35 |
|
6.05 |
|
2.37 |
iC4 |
0.71 |
|
0.08 |
|
1.05 |
|
0.76 |
nC4 |
1.44 |
|
0.10 |
|
2.44 |
|
0.96 |
iC5 |
0.53 |
|
0.04 |
|
0.88 |
|
0.69 |
nC5 |
0.66 |
|
0.04 |
|
1.17 |
|
0.51 |
C6 |
0.81 |
|
0.06 |
|
1.45 |
|
2.06 |
C7 |
1.20 |
|
0.06 |
|
2.38 |
|
2.63 |
C8 |
1.15 |
|
0.05 |
|
2.59 |
|
2.34 |
C9 |
0.63 |
|
0.04 |
|
1.75 |
|
2.35 |
C10 |
0.50 |
|
0.24 |
(+)195 |
1.50 |
|
29.52 (+)221 |
C11 |
0.29 |
|
|
|
1.55 |
|
|
C12 |
0.27 |
|
|
|
0.93 |
|
|
C13 |
0.28 |
|
|
|
1.13 |
|
|
C14 |
0.22 |
|
|
|
1.01 |
|
|
C15 |
0.17 |
|
|
|
0.80 |
|
|
C16 |
0.15 |
|
|
|
0.86 |
|
|
C17 |
0.14 |
|
|
|
0.60 |
|
|
C18 |
0.09 |
|
|
|
0.68 |
|
|
C19 |
0.13 |
(+)362 |
|
|
0.54 |
(+)411 |
|
C20 |
0.47 |
|
|
4.34 |
|
Table 2.4: Typical Fluid Analyses
Key: NS – North Sea |
SWT – South West Texas |
Bah – Bahrain |
GCGas Condensate |
VO – Volatile Oil |
BO – Black Oil |
7 Molecular weight will be defined shortly. Note its measurement can be subject to an error of 10 %.
Roxar Oxford |
20 |
12/12/12 |