
- •CONTENTS
- •Preface
- •Contributors
- •1 Introduction to Toxicology
- •1.1 Definition and Scope, Relationship to Other Sciences, and History
- •1.1.2 Relationship to Other Sciences
- •1.1.3 A Brief History of Toxicology
- •1.3 Sources of Toxic Compounds
- •1.3.1 Exposure Classes
- •1.3.2 Use Classes
- •1.4 Movement of Toxicants in the Environment
- •Suggested Reading
- •2.1 Introduction
- •2.2 Cell Culture Techniques
- •2.2.1 Suspension Cell Culture
- •2.2.2 Monolayer Cell Culture
- •2.2.3 Indicators of Toxicity in Cultured Cells
- •2.3 Molecular Techniques
- •2.3.1 Molecular Cloning
- •2.3.2 cDNA and Genomic Libraries
- •2.3.3 Northern and Southern Blot Analyses
- •2.3.4 Polymerase Chain Reaction (PCR)
- •2.3.5 Evaluation of Gene Expression, Regulation, and Function
- •2.4 Immunochemical Techniques
- •Suggested Reading
- •3.1 Introduction
- •3.2 General Policies Related to Analytical Laboratories
- •3.2.1 Standard Operating Procedures (SOPs)
- •3.2.2 QA/QC Manuals
- •3.2.3 Procedural Manuals
- •3.2.4 Analytical Methods Files
- •3.2.5 Laboratory Information Management System (LIMS)
- •3.3 Analytical Measurement System
- •3.3.1 Analytical Instrument Calibration
- •3.3.2 Quantitation Approaches and Techniques
- •3.4 Quality Assurance (QA) Procedures
- •3.5 Quality Control (QC) Procedures
- •3.6 Summary
- •Suggested Reading
- •4 Exposure Classes, Toxicants in Air, Water, Soil, Domestic and Occupational Settings
- •4.1 Air Pollutants
- •4.1.1 History
- •4.1.2 Types of Air Pollutants
- •4.1.3 Sources of Air Pollutants
- •4.1.4 Examples of Air Pollutants
- •4.1.5 Environmental Effects
- •4.2 Water and Soil Pollutants
- •4.2.1 Sources of Water and Soil Pollutants
- •4.2.2 Examples of Pollutants
- •4.3 Occupational Toxicants
- •4.3.1 Regulation of Exposure Levels
- •4.3.2 Routes of Exposure
- •4.3.3 Examples of Industrial Toxicants
- •Suggested Reading
- •5 Classes of Toxicants: Use Classes
- •5.1 Introduction
- •5.2 Metals
- •5.2.1 History
- •5.2.2 Common Toxic Mechanisms and Sites of Action
- •5.2.3 Lead
- •5.2.4 Mercury
- •5.2.5 Cadmium
- •5.2.6 Chromium
- •5.2.7 Arsenic
- •5.2.8 Treatment of Metal Poisoning
- •5.3 Agricultural Chemicals (Pesticides)
- •5.3.1 Introduction
- •5.3.3 Organochlorine Insecticides
- •5.3.4 Organophosphorus Insecticides
- •5.3.5 Carbamate Insecticides
- •5.3.6 Botanical Insecticides
- •5.3.7 Pyrethroid Insecticides
- •5.3.8 New Insecticide Classes
- •5.3.9 Herbicides
- •5.3.10 Fungicides
- •5.3.11 Rodenticides
- •5.3.12 Fumigants
- •5.3.13 Conclusions
- •5.4 Food Additives and Contaminants
- •5.5 Toxins
- •5.5.1 History
- •5.5.2 Microbial Toxins
- •5.5.3 Mycotoxins
- •5.5.4 Algal Toxins
- •5.5.5 Plant Toxins
- •5.5.6 Animal Toxins
- •5.6 Solvents
- •5.7 Therapeutic Drugs
- •5.8 Drugs of Abuse
- •5.9 Combustion Products
- •5.10 Cosmetics
- •Suggested Reading
- •6 Absorption and Distribution of Toxicants
- •6.1 Introduction
- •6.2 Cell Membranes
- •6.3 Mechanisms of Transport
- •6.3.1 Passive Diffusion
- •6.4 Physicochemical Properties Relevant to Diffusion
- •6.4.1 Ionization
- •6.5 Routes of Absorption
- •6.5.1 Extent of Absorption
- •6.5.2 Gastrointestinal Absorption
- •6.5.3 Dermal Absorption
- •6.5.4 Respiratory Penetration
- •6.6 Toxicant Distribution
- •6.6.1 Physicochemical Properties and Protein Binding
- •6.7 Toxicokinetics
- •Suggested Reading
- •7 Metabolism of Toxicants
- •7.1 Introduction
- •7.2 Phase I Reactions
- •7.2.4 Nonmicrosomal Oxidations
- •7.2.5 Cooxidation by Cyclooxygenases
- •7.2.6 Reduction Reactions
- •7.2.7 Hydrolysis
- •7.2.8 Epoxide Hydration
- •7.2.9 DDT Dehydrochlorinase
- •7.3 Phase II Reactions
- •7.3.1 Glucuronide Conjugation
- •7.3.2 Glucoside Conjugation
- •7.3.3 Sulfate Conjugation
- •7.3.4 Methyltransferases
- •7.3.7 Acylation
- •7.3.8 Phosphate Conjugation
- •Suggested Reading
- •8 Reactive Metabolites
- •8.1 Introduction
- •8.2 Activation Enzymes
- •8.3 Nature and Stability of Reactive Metabolites
- •8.4 Fate of Reactive Metabolites
- •8.4.1 Binding to Cellular Macromolecules
- •8.4.2 Lipid Peroxidation
- •8.4.3 Trapping and Removal: Role of Glutathione
- •8.5 Factors Affecting Toxicity of Reactive Metabolites
- •8.5.1 Levels of Activating Enzymes
- •8.5.2 Levels of Conjugating Enzymes
- •8.5.3 Levels of Cofactors or Conjugating Chemicals
- •8.6 Examples of Activating Reactions
- •8.6.1 Parathion
- •8.6.2 Vinyl Chloride
- •8.6.3 Methanol
- •8.6.5 Carbon Tetrachloride
- •8.6.8 Acetaminophen
- •8.6.9 Cycasin
- •8.7 Future Developments
- •Suggested Reading
- •9.1 Introduction
- •9.2 Nutritional Effects
- •9.2.1 Protein
- •9.2.2 Carbohydrates
- •9.2.3 Lipids
- •9.2.4 Micronutrients
- •9.2.5 Starvation and Dehydration
- •9.2.6 Nutritional Requirements in Xenobiotic Metabolism
- •9.3 Physiological Effects
- •9.3.1 Development
- •9.3.2 Gender Differences
- •9.3.3 Hormones
- •9.3.4 Pregnancy
- •9.3.5 Disease
- •9.3.6 Diurnal Rhythms
- •9.4 Comparative and Genetic Effects
- •9.4.1 Variations Among Taxonomic Groups
- •9.4.2 Selectivity
- •9.4.3 Genetic Differences
- •9.5 Chemical Effects
- •9.5.1 Inhibition
- •9.5.2 Induction
- •9.5.3 Biphasic Effects: Inhibition and Induction
- •9.6 Environmental Effects
- •9.7 General Summary and Conclusions
- •Suggested Reading
- •10 Elimination of Toxicants
- •10.1 Introduction
- •10.2 Transport
- •10.3 Renal Elimination
- •10.4 Hepatic Elimination
- •10.4.2 Active Transporters of the Bile Canaliculus
- •10.5 Respiratory Elimination
- •10.6 Conclusion
- •Suggested Reading
- •11 Acute Toxicity
- •11.1 Introduction
- •11.2 Acute Exposure and Effect
- •11.3 Dose-response Relationships
- •11.4 Nonconventional Dose-response Relationships
- •11.5 Mechanisms of Acute Toxicity
- •11.5.1 Narcosis
- •11.5.2 Acetylcholinesterase Inhibition
- •11.5.3 Ion Channel Modulators
- •11.5.4 Inhibitors of Cellular Respiration
- •Suggested Reading
- •12 Chemical Carcinogenesis
- •12.1 General Aspects of Cancer
- •12.2 Human Cancer
- •12.2.1 Causes, Incidence, and Mortality Rates of Human Cancer
- •12.2.2 Known Human Carcinogens
- •12.3 Classes of Agents Associated with Carcinogenesis
- •12.3.2 Epigenetic Agents
- •12.4 General Aspects of Chemical Carcinogenesis
- •12.5 Initiation-Promotion Model for Chemical Carcinogenesis
- •12.6 Metabolic Activation of Chemical Carcinogens and DNA Adduct Formation
- •12.7 Oncogenes
- •12.8 Tumor Suppressor Genes
- •12.8.1 Inactivation of Tumor Suppressor Genes
- •12.8.2 p53 Tumor Suppressor Gene
- •12.9 General Aspects of Mutagenicity
- •12.10 Usefulness and Limitations of Mutagenicity Assays for the Identification of Carcinogens
- •Suggested Reading
- •13 Teratogenesis
- •13.1 Introduction
- •13.2 Principles of Teratology
- •13.3 Mammalian Embryology Overview
- •13.4 Critical Periods
- •13.5 Historical Teratogens
- •13.5.1 Thalidomide
- •13.5.2 Accutane (Isotetrinoin)
- •13.5.3 Diethylstilbestrol (DES)
- •13.5.4 Alcohol
- •13.6 Testing Protocols
- •13.6.1 FDA Guidelines for Reproduction Studies for Safety Evaluation of Drugs for Human Use
- •13.6.3 Alternative Test Methods
- •13.7 Conclusions
- •Suggested Reading
- •14 Hepatotoxicity
- •14.1 Introduction
- •14.1.1 Liver Structure
- •14.1.2 Liver Function
- •14.2 Susceptibility of the Liver
- •14.3 Types of Liver Injury
- •14.3.1 Fatty Liver
- •14.3.2 Necrosis
- •14.3.3 Apoptosis
- •14.3.4 Cholestasis
- •14.3.5 Cirrhosis
- •14.3.6 Hepatitis
- •14.3.7 Oxidative Stress
- •14.3.8 Carcinogenesis
- •14.4 Mechanisms of Hepatotoxicity
- •14.5 Examples of Hepatotoxicants
- •14.5.1 Carbon Tetrachloride
- •14.5.2 Ethanol
- •14.5.3 Bromobenzene
- •14.5.4 Acetaminophen
- •14.6 Metabolic Activation of Hepatotoxicants
- •Suggested Reading
- •15 Nephrotoxicity
- •15.1 Introduction
- •15.1.1 Structure of the Renal System
- •15.1.2 Function of the Renal System
- •15.2 Susceptibility of the Renal System
- •15.3 Examples of Nephrotoxicants
- •15.3.1 Metals
- •15.3.2 Aminoglycosides
- •15.3.3 Amphotericin B
- •15.3.4 Chloroform
- •15.3.5 Hexachlorobutadiene
- •Suggested Reading
- •16 Toxicology of the Nervous System
- •16.1 Introduction
- •16.2 The Nervous system
- •16.2.1 The Neuron
- •16.2.2 Neurotransmitters and their Receptors
- •16.2.3 Glial Cells
- •16.3 Toxicant Effects on the Nervous System
- •16.3.1 Structural Effects of Toxicants on Neurons
- •16.3.2 Effects of Toxicants on Other Cells
- •16.4 Neurotoxicity Testing
- •16.4.1 In vivo Tests of Human Exposure
- •16.4.2 In vivo Tests of Animal Exposure
- •16.4.3 In vitro Neurochemical and Histopathological End Points
- •16.5 Summary
- •Suggested Reading
- •17 Endocrine System
- •17.1 Introduction
- •17.2 Endocrine System
- •17.2.1 Nuclear Receptors
- •17.3 Endocrine Disruption
- •17.3.1 Hormone Receptor Agonists
- •17.3.2 Hormone Receptor Antagonists
- •17.3.3 Organizational versus Activational Effects of Endocrine Toxicants
- •17.3.4 Inhibitors of Hormone Synthesis
- •17.3.5 Inducers of Hormone Clearance
- •17.3.6 Hormone Displacement from Binding Proteins
- •17.4 Incidents of Endocrine Toxicity
- •17.4.1 Organizational Toxicity
- •17.4.2 Activational Toxicity
- •17.4.3 Hypothyroidism
- •17.5 Conclusion
- •Suggested Reading
- •18 Respiratory Toxicity
- •18.1 Introduction
- •18.1.1 Anatomy
- •18.1.2 Cell Types
- •18.1.3 Function
- •18.2 Susceptibility of the Respiratory System
- •18.2.1 Nasal
- •18.2.2 Lung
- •18.3 Types of Toxic Response
- •18.3.1 Irritation
- •18.3.2 Cell Necrosis
- •18.3.3 Fibrosis
- •18.3.4 Emphysema
- •18.3.5 Allergic Responses
- •18.3.6 Cancer
- •18.3.7 Mediators of Toxic Responses
- •18.4 Examples of Lung Toxicants Requiring Activation
- •18.4.1 Introduction
- •18.4.2 Monocrotaline
- •18.4.3 Ipomeanol
- •18.4.4 Paraquat
- •18.5 Defense Mechanisms
- •Suggested Reading
- •19 Immunotoxicity
- •19.1 Introduction
- •19.2 The Immune System
- •19.3 Immune Suppression
- •19.4 Classification of Immune-Mediated Injury (Hypersensitivity)
- •19.5 Effects of Chemicals on Allergic Disease
- •19.5.1 Allergic Contact Dermatitis
- •19.5.2 Respiratory Allergens
- •19.5.3 Adjuvants
- •19.6 Emerging Issues: Food Allergies, Autoimmunity, and the Developing Immune System
- •Suggested Reading
- •20 Reproductive System
- •20.1 Introduction
- •20.2 Male Reproductive Physiology
- •20.3 Mechanisms and Targets of Male Reproductive Toxicants
- •20.3.1 General Mechanisms
- •20.3.2 Effects on Germ Cells
- •20.3.3 Effects on Spermatogenesis and Sperm Quality
- •20.3.4 Effects on Sexual Behavior
- •20.3.5 Effects on Endocrine Function
- •20.4 Female Reproductive Physiology
- •20.5 Mechanisms and Targets of Female Reproductive Toxicants
- •20.5.1 Tranquilizers, Narcotics, and Social Drugs
- •20.5.2 Endocrine Disruptors (EDs)
- •20.5.3 Effects on Germ Cells
- •20.5.4 Effects on the Ovaries and Uterus
- •20.5.5 Effects on Sexual Behavior
- •Suggested Reading
- •21 Toxicity Testing
- •21.1 Introduction
- •21.2 Experimental Administration of Toxicants
- •21.2.1 Introduction
- •21.2.2 Routes of Administration
- •21.3 Chemical and Physical Properties
- •21.4 Exposure and Environmental Fate
- •21.5 In vivo Tests
- •21.5.1 Acute and Subchronic Toxicity Tests
- •21.5.2 Chronic Tests
- •21.5.3 Reproductive Toxicity and Teratogenicity
- •21.5.4 Special Tests
- •21.6 In vitro and Other Short-Term Tests
- •21.6.1 Introduction
- •21.6.2 Prokaryote Mutagenicity
- •21.6.3 Eukaryote Mutagenicity
- •21.6.4 DNA Damage and Repair
- •21.6.5 Chromosome Aberrations
- •21.6.6 Mammalian Cell Transformation
- •21.6.7 General Considerations and Testing Sequences
- •21.7 Ecological Effects
- •21.7.1 Laboratory Tests
- •21.7.2 Simulated Field Tests
- •21.7.3 Field Tests
- •21.8 Risk Analysis
- •21.9 The Future of Toxicity Testing
- •Suggested Reading
- •22 Forensic and Clinical Toxicology
- •22.1 Introduction
- •22.2 Foundations of Forensic Toxicology
- •22.3 Courtroom Testimony
- •22.4.1 Documentation Practices
- •22.4.2 Considerations for Forensic Toxicological Analysis
- •22.4.3 Drug Concentrations and Distribution
- •22.5 Laboratory Analyses
- •22.5.1 Colorimetric Screening Tests
- •22.5.2 Thermal Desorption
- •22.5.6 Enzymatic Immunoassay
- •22.6 Analytical Schemes for Toxicant Detection
- •22.7 Clinical Toxicology
- •22.7.1 History Taking
- •22.7.2 Basic Operating Rules in the Treatment of Toxicosis
- •22.7.3 Approaches to Selected Toxicoses
- •Suggested Reading
- •23 Prevention of Toxicity
- •23.1 Introduction
- •23.2 Legislation and Regulation
- •23.2.1 Federal Government
- •23.2.2 State Governments
- •23.2.3 Legislation and Regulation in Other Countries
- •23.3 Prevention in Different Environments
- •23.3.1 Home
- •23.3.2 Workplace
- •23.3.3 Pollution of Air, Water, and Land
- •23.4 Education
- •Suggested Reading
- •24 Human Health Risk Assessment
- •24.1 Introduction
- •24.2 Risk Assessment Methods
- •24.2.2 Exposure Assessment
- •24.2.3 Dose Response and Risk Characterization
- •24.3 Noncancer Risk Assessment
- •24.3.1 Default Uncertainty and Modifying Factors
- •24.3.2 Derivation of Developmental Toxicant RfD
- •24.3.3 Determination of RfD and RfC of Naphthalene with the NOAEL Approach
- •24.3.4 Benchmark Dose Approach
- •24.3.5 Determination of BMD and BMDL for ETU
- •24.3.6 Quantifying Risk for Noncarcinogenic Effects: Hazard Quotient
- •24.3.7 Chemical Mixtures
- •24.4 Cancer Risk Assessment
- •24.5 PBPK Modeling
- •Suggested Reading
- •25 Analytical Methods in Toxicology
- •25.1 Introduction
- •25.2 Chemical and Physical Methods
- •25.2.1 Sampling
- •25.2.2 Experimental Studies
- •25.2.3 Forensic Studies
- •25.2.4 Sample Preparation
- •25.2.6 Spectroscopy
- •25.2.7 Other Analytical Methods
- •Suggested Reading
- •26 Basics of Environmental Toxicology
- •26.1 Introduction
- •26.2 Environmental Persistence
- •26.2.1 Abiotic Degradation
- •26.2.2 Biotic Degradation
- •26.2.3 Nondegradative Elimination Processes
- •26.3 Bioaccumulation
- •26.4 Toxicity
- •26.4.1 Acute Toxicity
- •26.4.2 Mechanisms of Acute Toxicity
- •26.4.3 Chronic Toxicity
- •26.4.5 Abiotic and Biotic Interactions
- •26.5 Conclusion
- •Suggested Reading
- •27.1 Introduction
- •27.2 Sources of Toxicants to the Environment
- •27.3 Transport Processes
- •27.3.1 Advection
- •27.3.2 Diffusion
- •27.4 Equilibrium Partitioning
- •27.5 Transformation Processes
- •27.5.1 Reversible Reactions
- •27.5.2 Irreversible Reactions
- •27.6 Environmental Fate Models
- •Suggested Reading
- •28 Environmental Risk Assessment
- •28.1 Introduction
- •28.2 Formulating the Problem
- •28.2.1 Selecting Assessment End Points
- •28.2.2 Developing Conceptual Models
- •28.2.3 Selecting Measures
- •28.3 Analyzing Exposure and Effects Information
- •28.3.1 Characterizing Exposure
- •28.3.2 Characterizing Ecological Effects
- •28.4 Characterizing Risk
- •28.4.1 Estimating Risk
- •28.4.2 Describing Risk
- •28.5 Managing Risk
- •Suggested Reading
- •29 Future Considerations for Environmental and Human Health
- •29.1 Introduction
- •29.2 Risk Management
- •29.3 Risk Assessment
- •29.4 Hazard and Exposure Assessment
- •29.5 In vivo Toxicity
- •29.6 In vitro Toxicity
- •29.7 Biochemical and Molecular Toxicology
- •29.8 Development of Selective Toxicants
- •Glossary
- •Index

|
PHASE II REACTIONS |
137 |
|
OH |
|
O |
OH |
|
+ |
H2O |
|
Styrene 7,8-oxide |
Styrene 7,8-glycol |
|
|
HO H |
|
O |
OH |
|
+ |
H |
|
H2O |
|
|
Naphthalene 1,2-oxide |
Naphthalene dihydrodiol |
|
Figure 7.14 Examples of epoxide hydrolase reactions.
|
|
H |
|
|
|
|
|
|
|
|
|
|
|
|
|
|
|
|
|
|
|
|
|
|
|
|
|
|
|
|
|
|
|
|
|
|
|
|
Cl |
|
C |
|
Cl |
|
Cl |
|
C |
|
Cl |
||
|
|
|
||||||||||
|
|
|
|
|
|
|
|
|
|
|
|
|
|
|
CCl3 |
|
|
|
|
|
CCl2 |
|
|
||
|
|
DDT |
|
|
|
|
|
DDE |
|
|
Figure 7.15 DDT-dehydrochlorinase.
because it does not appear to be consumed during the reaction. The Km for DDT is 5 × 10−7 mol/L with optimum activity at pH 7.4. The monomeric form of the enzyme has a molecular mass of about 36,000 daltons, but the enzyme normally exists as a tetramer. In addition to catalyzing the dehydrochlorination of DDT to DDE and DDD (2,2-bis(p-chlorophenyl)-1,1-dichloroethane) to TDE (2,2-bis(p-chlorophenyl)- 1-chlorothylen), DDT dehydrochlorinase also catalyzes the dehydrohalogenation of a number of other DDT analogues. In all cases the p,p configuration is required, o,p, and other analogues are not utilized as substrates. The reaction is illustrated in Figure 7.15.
7.3PHASE II REACTIONS
Products of phase I metabolism and other xenobiotics containing functional groups such as hydroxyl, amino, carboxyl, epoxide, or halogen can undergo conjugation reactions with endogenous metabolites, these conjugations being collectively termed phase II reactions. The endogenous metabolites in question include sugars, amino acids, glutathione, sulfate, and so on. Conjugation products, with only rare exceptions, are more polar, less toxic, and more readily excreted than are their parent compounds.
Conjugation reactions usually involve metabolite activation by some high-energy intermediate and have been classified into two general types: type I, in which an activated conjugating agent combines with the substrate to yield the conjugated product, and type II, in which the substrate is activated and then combines with an amino acid

138 METABOLISM OF TOXICANTS
to yield a conjugated product. The formation of sulfates and glycosides are examples of type I, whereas type II consists primarily of amino acid conjugation.
7.3.1Glucuronide Conjugation
The glucuronidation reaction is one of the major pathways for elimination of many lipophilic xenobiotics and endobiotics from the body. The mechanism for this conjugation involves the reaction of one of many possible functional groups (R–OH, Ar–OH, R–NH2, Ar–NH2, R–COOH, Ar–COOH) with the sugar derivative, uridine 5 -diphosphoglucuronic acid (UDPGA). Homogeneous glucuronosyl transferase has been isolated as a single polypeptide chain of about 59,000 daltons, apparently containing carbohydrate, whose activity appears to be dependent on reconstitution with microsomal lipid. There appears to be an absolute requirement for UDPGA: related UDP-sugars will not suffice. This enzyme, as it exists in the microsomal membrane, does not exhibit its maximal capacity for conjugation; activation by some means (e.g., detergents), is required. The reaction involves a nucleophilic displacement (SN2 reaction) of the functional group of the substrate with Walden inversion. UDPGA is in the α-configuration whereas, due to the inversion, the glucuronide formed is in the β-configuration. The enzyme involved, the UDP glucuronosyl transferase (UGT), is found in the microsomal fraction of liver, kidney, intestine, and other tissues. Examples of various types of glucuronides are shown in Figure 7.16.
HO
|
|
NH |
|
|
|
|
|
|
COOH |
|
|
|
|
|
|
|
|
|
O |
||
|
|
|
|
|
|
|
COOH |
|
||
OH |
|
|
OH |
|
|
|
|
HO |
||
|
N |
|
|
|
O |
|
|
|||
|
|
|
|
|
|
|
OH |
|||
|
|
|
|
|
|
HO |
|
O |
||
|
|
|
|
|
|
|
|
|||
|
|
|
O |
O |
O |
|
|
OH |
||
+ |
HO |
|
P O |
OH |
OH |
UGT |
+ UDP |
|||
|
O |
|
|
|
||||||
|
|
P |
O |
|
||||||
|
|
HO |
OH |
|
|
|
||||
|
|
HO |
|
|
|
|
|
|
||
1-Naphthol |
|
|
UDPGA |
|
|
|
|
|
Naphthol glucuronide |
|
|
|
|
|
(a) Reaction Sequence |
|
|
||||
|
O |
|
|
|
|
|
|
NH2 |
|
SH |
Coumarin |
|
|
|
|
2-Naphthylamine |
Thiophenol |
||||
|
|
|
|
|
|
HO |
O |
|
|
|
|
OH |
|
|
|
|
|
|
|
H3C |
|
O |
|
|
|
|
|
N |
NH |
|
||
NH |
|
|
|
|
N |
N |
||||
|
|
|
|
|
|
|
|
|||
|
|
|
|
|
|
|
|
|
|
|
|
|
CH3 |
|
|
|
|
|
H3C |
|
|
|
H3C |
|
|
|
|
|
|
|
Cl |
|
Propanolol |
|
|
|
|
Oxazapam |
Imipramine |
||||
|
|
|
|
(b) Substrate Examples |
|
|
Figure 7.16 Reaction sequences of uridine diphospho glucuronosyl transferase and chemical structures of compounds that form glucuronides. Arrows indicate the position on each molecule where glucuronidation occurs.
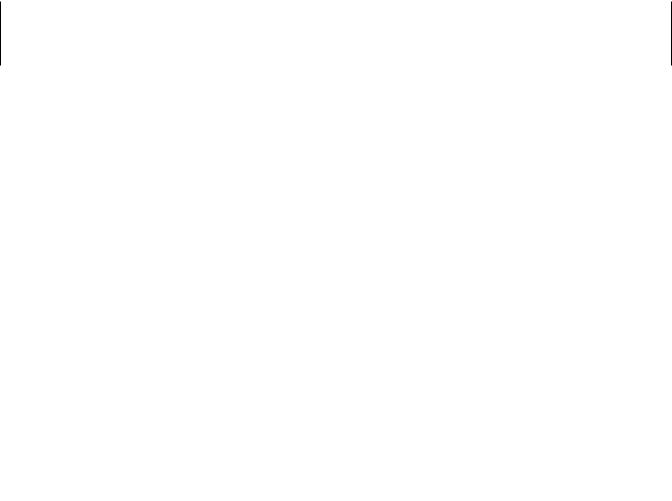
PHASE II REACTIONS |
139 |
Glucuronide conjugation generally results in the formation of products that are less biologically and chemically reactive. This, combined with their greater polarity and greater susceptibility to excretion, contributes greatly to the detoxication of most xenobiotics. However, there are now many examples where glucuronide conjugation results in greater toxicity. Perhaps the best-known example involves the bioactivation of N -hydroxy-2-acetylaminofluorine. This substrate, unlike 2-acetylaminofluorine, is unable to bind to DNA in the absence of metabolism. However, following glucuronide conjugation by linkage of the oxygen through the N-hydroxy group, this substrate becomes equipotent as a hepatocarcinogen with 2-acetylaminofluorine based on its ability to bind to DNA. Another relatively large class of xenobiotics that are often activated by glucuronide conjugation are the acyl glucuronides of carboxylic acids. Useful therapeutic drugs within this class include nonsteroidal anti-inflammatory drugs (NSAIDS), hypolipidemic drugs (clofibrate), and anticonvulsants (valproic acid). The various syndromes associated with the clinical use of some of these drugs (including cytotoxic, carcinogenic, and various immunologic effects) are thought to be the result of the ability of the glucuronide conjugates to react with nucleophilic macromolecules (protein and DNA).
A wide variety of reactions are mediated by glucuronosyltransferases, O-glucuro- nides, N-glucuronides, and S-glucuronides have all been identified. At this time over 35 different UGT gene products have been described from several different species. These are responsible for the biotransformation of greater than 350 different substrates. Evidence from molecular cloning suggests that the UGTs belong to one of two large superfamilies, sharing less than 50% amino acid identity. Nomenclature of these genes is similar to that of the CYP superfamily. The UGT1 gene family consists of a number of UGTs that arise from alternate splicing of multiple first exons and share common exons 2–5. Members of the UGT2 family catalyze the glucuronidation of a wide variety of substrates including steroids, bile acids, and opioids.
There are nine known human isozymes within the UGT1 family and six within the UGT2 family. Polymorphic forms of some of these enzymes are associated with diseases and significant adverse effects to some drugs.
Jaundice, a condition resulting from the failure of either transport or conjugation of bilirubin, becomes clinically evident when serum bilirubin levels exceed 35 µM. Although the human UGT1A locus encompasses nine functional transferase genes, only one isoform, UGT1A1, is involved in inherited diseases of bilirubin metabolism. All three inheritable hyperbilirubineamias are the result of either mutant UGT1A1 alleles or UGT1A1 promoter polymorphisms. To date, 33 mutant UGT1A1 alleles have been identified. For the disease to be clinically manifest, one must either be homozygous for the mutant allele or have multiple heterozygous mutant alleles.
7.3.2Glucoside Conjugation
Although rare in vertebrates, glucosides formed from xenobiotics are common in insects and plants. Formed from UDP-glucose, they appear to fall into the same classes as the glucuronides.
7.3.3Sulfate Conjugation
Sulfation and sulfate conjugate hydrolysis, catalyzed by various members of the sulfotransferases (SULT) and sulfatase enzyme superfamilies, play important roles in the

140 METABOLISM OF TOXICANTS
metabolism and disposition of many xenobiotics and endogenous substrates. Reactions of the sulfotransferase enzyme with various xenobiotics, including alcohols, arylamines, and phenols, result in the production of water soluble sulfate esters that often are readily eliminated from the organism. Although generally these reactions are important in detoxication, they have also been shown to be involved in carcinogen activation, prodrug processing, cellular signaling pathways, and the regulation of several potent endogenous chemicals including thyroid hormones, steroids, and catechols. The overall sulfation pathway shown in Figure 7.17, consists of two enzyme systems: the SULTs, which catalyze the sulfation reaction, and the sulfatases, which catalyze the hydrolysis of sulfate esters formed by the action of the SULTs.
Sulfation is expensive in energy terms for the cell, since two molecules of ATP are necessary for the synthesis of one molecule of 3 -phosphoadenosine 5 -phosphosulfate (PAPS). Both enzymes involved in the synthesis of PAPS, ATP sulfurylase, and APS kinase, reside within a single bifunctional cytosolic protein of approximately 56 kDa, where substrate channeling of APS from ATP sulfurylase to APS kinase occurs. Several group VI anions other than sulfate can also serve as substrates, although the resultant anhydrides are unstable. Because this instability would lead to the overall consumption of ATP, these other anions can exert a toxic effect by depleting the cell of ATP.
In humans, there are five well-characterized SULT genes, each possessing widely different amino acid sequences and with widely different substrate specificities. Based
|
|
|
|
|
OH |
ATP |
PPi |
ATP |
ADP |
|
4-nitrophenol |
|
|
|
|
|
|
SO42− |
APS |
|
PAPS |
|
|
|
|
|
|
|
NO2 |
|
|
|
Sulfotransferase |
Sulfatase |
|
|
|
|
|
|
OSO3− |
|
|
|
PAP |
|
4-nitrophenol |
|
|
|
|
|
|
|
|
|
|
|
sulfate |
|
|
|
|
|
NO2 |
|
|
|
(a) Reaction Sequence |
|
|
|
NH2 |
|
|
|
|
|
|
|
OH3C |
|
OH |
|
|
|
|
|
|
Naphthalene |
|
|
Estrone |
|
|
|
|
|
|
|
|
|
|
|
|
|
O |
OH |
|
|
NH |
2 |
CH3 |
|
HO |
|
NH |
||
|
|
|
HO |
CH3
H3C
HO
2-Butanol Dopamine Acetaminophen
(b) Substrate Examples
Figure 7.17 Reaction sequence of sulfotransferases and chemical structures of compounds that form sulfates. Arrows indicate positions on each molecule where sulfotransferases may attack.
- #15.08.20134.04 Mб15Hastie T., Tibshirani R., Friedman J. - The Elements of Statistical Learning Data Mining, Inference and Prediction (2002)(en).djvu
- #
- #
- #
- #
- #
- #
- #
- #15.08.201315.44 Mб23Hudlicky M, Pavlath A.E. (eds.) - Chemistry of Organic Fluorine Compounds 2[c] A critical Review (1995)(en).djvu
- #
- #