
Solid Support Oligosaccharide Synthesis
.pdf98POLYMER-SUPPORTED SYNTHESIS OF OLIGOSACCHARIDES
17.Adinolfi, M., Barone, G., De Napoli, L., Guariniello, L., Iadonisi, A., and Piccialli, G.,
Tetrahedron Lett. 40, 2607–2610 (1999).
18.Paulsen, H., Schleyer, A., Mathieux, N., Meldal, M., and Bock, K.,J. Chem. Soc. Perkin Trans 1, 281–293 (1997).
Solid Support Oligosaccharide Synthesis and Combinatorial Carbohydrate Libraries. Edited by Peter H. Seeberger Copyright © 2001 John Wiley & Sons, Inc.
ISBNs: 0-471-37828-3 (Hardback); 0-471-22044-2 (Electronic)
5Synthesis of Oligosaccharides on Solid Support Using Thioglycosides and Pentenyl Glycosides
VALENTIN WITTMANN
Institut für Organische Chemie, Johann Wolfgang Goethe-Universität, Frankfurt am Main, Germany
5.1 INTRODUCTION
Today, a multitude of methods for formation of glycosidic linkages is available to the synthetic organic chemist. Covered here are solid-phase oligosaccharide syntheses relying on the use of thioglycosides and n-pentenyl glycosides as glycosyl donors. They differ from many other glycosyl donors because they are stable under a variety of reaction conditions commonly employed in oligosaccharide synthesis but at the same time can be selectively activated when required. Thus, they serve both as a means of anomeric protection and activation. Activation of thioglycosides and n-pentenyl glycosides proceeds on addition of a soft electrophile under mild conditions, which is helpful for the construction of sensitive target molecules. Their chemical stability—especially that of thioglycosides—has been exploited mainly in block syntheses of oligosaccharides in solution where they are activated late in the synthetic sequence. On solid support, the main advantage of the chemical stability of thioglycosides and n-pentenyl glycosides is that these glycosidic linkages can be used to anchor the growing oligosaccharide chain on the resin.
5.2 THIOGLYCOSIDES AS GLYCOSYL DONORS
Thioglycosides were the first example of an anomeric derivatization that serves the dual role of protection and activation. They were introduced in glycosylation reactions by Ferrier et al. in 1973, who used mercury(II) salts as activator.1 After the development of improved methods of their synthesis and activation, thioglycosides together with trichloroacetimidates are now the most commonly used glycosyl
99
100 SYNTHESIS OF OLIGOSACCHARIDES ON SOLID SUPPORT
donors.2–6 Thioglycosides are conveniently obtained by a number of substitution reactions of anomerically activated carbohydrates.2,4 Examples include thiolysis of glycosyl bromides and chlorides under basic conditions, thiolysis of glycosyl acetates and trichloroacetimidates under Lewis acid catalysis, tin-mediated thioalkylation of glycosyl acetates, and the Lewis acid–catalyzed reaction of glycosyl acetates with trimethylsilyl thioethers. The direct conversion of aldoses with thiols into thioglycosides under conditions normally used with alcohols for Fischer glycosidation is not possible. Under these conditions dithioacetals rather than thioglycosides are obtained.
5.2.1 Activation Procedures
A distinct advantage of thioglycosides is the fact that they are stable under a wide range of reaction conditions commonly employed in the synthesis of oligosaccharides whereas at the same time they can be employed for most of the coupling procedures currently in use. Thus, thioglycosides may be introduced at an early stage of a synthesis and later on be converted into a glycosyl donor, making them interesting intermediates in the block synthesis of oligosaccharides.
Thioglycosides readily react with bromine and chlorine to afford glycosyl bromides7 and glycosyl chlorides,8 respectively, which can be transformed into O-glycosides under promotion by Ag+, Hg2+, or tetraalkylammonium bromide.9 Alternatively, the halogenoses may be hydrolyzed to the lactols, which can be further derivatized to give trichloroacetimidates.10 The Hg2+-promoted conversion of thioglycosides into O-glycosides introduced by Ferrier1 was the first example of the direct use of thioglycosides in O-glycosylation reactions. Subsequently, Hanessian et al. demonstrated the use of the 2-pyridylthio group.11 The application of heavy-metal salts is, however, of limited utility since they suffer from low reactivity and inconvenience. Nicolaou et al.12 as well as Hanessian et al.11 used N-bromosuccinimide (NBS), but this method also has disadvantages, such as variable reactivity and somewhat poor selectivity.
Recently, a number of highly specific thiophilic activators have been introduced (Scheme 5.1a). Methyl triflate13 efficiently S-alkylates the thioglycoside function to give an anomeric sulfonium triflate. This species (I), or the derived glycosyl triflate, is then substituted by the alcohol. However, methyl triflate is toxic and in the presence of slow-reacting glycosyl donors can give rise to methyl ethers in addition to O-glycosides. This can be overcome by the use of the soft electrophile dimethyl(methylthio)sulfonium triflate (DMTST)14 which methylsulfenylates the thioglycoside and gives fast glycosylations at room temperature or below. A side reaction has been reported in which an acetamidosugar underwent N-methylsulfenylation by DMTST.15 Care must also be taken in the presence of the allyloxycarbonyl protecting group, which is not compatible with DMTST.16 Other examples of thiophilic electrophiles include phenylselenyl triflate (PhSeOTf),17,18 N-iodosuccinimide-triflic acid (NIS-TfOH),19,20 and iodonium di-sym-collidine perchlorate (IDCP).21 IDCP is not commercially available, but it is easily prepared by the procedure of Lemieux and Morgan.22
Treatment of thioglycosides with N-bromosuccinimide (NBS) in the presence of diethylaminosulfur trifluoride (DAST) or HF-pyridine complex furnishes glycosyl fluorides.23 These can be activated with AgClO4/SnCl2 in the presence of another

|
|
|
|
5.2 THIOGLYCOSIDES AS GLYCOSYL DONORS 101 |
||||
(a) |
|
|
|
|
X |
|
|
|
|
O |
SR |
“X+” |
O |
S R |
R’OH |
O |
OR’ |
|
|
|
|
|
|
– RSX |
|
|
|
|
|
|
I |
|
|
|
|
(b) |
O |
SR |
TBPA•+ |
O |
S R |
R’OH |
O |
OR’ |
|
||||||||
|
|
|
|
|
|
– RS• |
|
|
|
|
|
Br |
II |
|
|
|
|
|
TBPA•+ = |
|
|
SbCl6 |
|
|
|
|
|
|
N |
|
|
|
|
|
|
|
|
|
|
|
|
|
|
|
|
|
Br |
|
Br |
|
|
|
|
(c) |
|
|
|
|
O |
O |
O |
|
|
|
|
|
F3C S O S CF3 |
|
|||
|
O |
SPh m-CPBA |
O |
S Ph |
O OR |
|||
|
O |
O |
ROH
Scheme 5.1 Mechanism of thioglycoside activation (a) by thiophiles “X+” such as N-bromosuccinimide (NBS),11,12 methyl triflate,13 dimethyl(methylthio)sulfonium triflate (DMTST),14 phenylselenyl triflate (PhSeOTf),17,18 N-iodosuccinimide/triflic acid (NIS/TfOH),19,20 and iodonium di-sym-collidine perchlorate (IDCP)21; (b) by tris(4-bromophenyl)ammoniumyl hexachloroantimonate (TBPA•+)25; and (c) via anomeric sulfoxides.26 The stereochemical outcome of these glycosylations follows the same general trends as with many other glycosyl donor/promoter combinations (m-CPBA = meta-chloroperbenzoic acid).
thioglycoside, thus it is possible to use thioglycosidic acceptors, a feature that is exploited in the two-stage activation procedure.23,24
Tris(4-bromophenyl)ammoniumyl hexachloroantimonate (TBPA•+) is a rather novel activator for thioglycosides which has been developed by Sinaÿ et al. (Scheme 5.1b).25 This method involves a single-electron transfer step from sulfur to the radical cation TBPA•+, thus generating a glycosyl radical cation II that extruses a thiyl radical on addition of the hydroxylic glycosyl acceptor. Another new method of O-glycosylation using thioglycosides has been reported by Kahne et al. (Scheme
In this two-step procedure thioglycosides are first oxidized by meta-chloroperbenzoic acid (m-CPBA) to give anomeric sulfoxides, which are activated by triflic anhydride in a second step. Related to this method is an approach developed by Ley et al. involving oxidation of thioglycosides to the sulfone stage followed by displacement of the sulfonyl group by alcohols in the presence of magnesium bromide etherate and sodium bicarbonate.27,28
5.2.2 Applications to Solid-Phase Synthesis
Thioglycosides have been applied in solid-phase oligosaccharide synthesis by several research groups.29–35 Kahne et al. used them as precursors for the generation of

102 SYNTHESIS OF OLIGOSACCHARIDES ON SOLID SUPPORT
glycosyl sulfoxides, which are valuable glycosyl donors in solid-phase synthesis because of their high reactivity on activation by triflic anhydride even at low temperatures and their nonpolar character.36,37 Danishefsky et al. developed a method for the conversion of resin-bound glycals into 2-O-pivaloyl thioglycosides.31 These were subsequently coupled to several hindered glycosyl acceptors to furnish β-(1→2), β-(1→3), β-(1→4), and β-(1→6) linkages. Especially in the case of glucosyl donors, the use of thioglycosides is advantageous over the so far used 1,2-anhydrosugar derivatives. Subsequently, thioglycoside intermediates were also employed by the same group for the transformation of immobilized glycals into β-glycosides of glucosamine.32 Further examples of solid support syntheses with thioglycoside donors have been reported by the groups of Boons,33 Kanie and Wong,34 and Takahashi.35
Thioglycosides have been also used in glycosylation reactions with soluble polymers38,39 and for anchoring oligosaccharides on a solid support.36,37,40–43 Some of
this work is described elsewhere in this book in more detail and thus is not covered in this chapter.
In 1997 Nicolaou et al. published the assembly of a heptasaccharide phytoalexin elicitor (HPE, 16) on a solid support (Schemes 5.2–5.4).29 This oligosaccharide
(a) |
OTBDPS |
OTBDPS |
|
|
OAc |
|
|
|
|
||||
BzO |
O |
BnO |
O |
|
AcO |
O |
BzO |
SPh |
FmocO |
SPh |
|
AcO |
SMe |
|
OBz |
|
OBz |
|
|
OAc |
|
1 |
2 |
|
|
|
3 |
(b) |
|
|
|
|
|
|
|
1. n-BuLi |
|
|
|
|
|
|
2. O2 |
HO |
|
Polystyrene |
HO |
|
Polystyrene |
|
|||||
|
3. PPh3 |
|
|
|
|
|
4 |
|
|
5 |
|
|
|
O |
I |
|
|
|
O |
I |
|
1, DMTST |
|
OTBDPS |
|
5, Cs2CO3 |
|
|
BzO |
O |
|
|
||
HO |
|
|
|
|
||
95% |
BzO |
O |
|
>90% |
||
|
|
OBz |
|
|
||
NO2 |
|
|
NO2 |
|
||
|
7 |
|
|
|||
6 |
|
|
|
|
|
|
|
|
|
|
|
|
|
|
O |
O |
|
|
|
|
OR |
hν |
|
|
OTBDPS |
||
BzO |
O |
|
BzO |
O |
|
|
|
|
OH |
||||
BzO |
O |
95% |
BzO |
|||
|
OBz |
|
OBz |
|||
|
|
|
|
|||
|
NO2 |
|
|
|
|
|
HF•py |
8: R = TBDPS |
|
|
|
|
|
|
|
|
|
|
|
|
>98% |
9: R = H |
|
|
|
|
|
|
|
|
|
|
|
Scheme 5.2 (a) Monosaccharide building blocks 1–3 selected for solid phase synthesis of heptasaccharide 16; (b) attachment of the first carbohydrate onto phenolic polystyrene through a new photocleavable o-nitrobenzyl-type linker29 (Bn = benzyl, Bz = benzoyl, Fmoc = 9-fluoromethyloxycarbonyl, Ph = phenyl, py = pyridine, TBDPS = t-butyldiphenylsilyl).

5.2 THIOGLYCOSIDES AS GLYCOSYL DONORS 103
|
OH |
BzO |
O |
BzO |
O |
|
OBz |
|
9 |
O
NO2
AcO
AcO
AcO
AcO
AcO
AcO
AcO
AcO
|
|
OTBDPS |
|
|
|
O |
BnO |
O |
O |
O |
|
HO |
O |
||||
|
|
|
|||
1. 2, DMTST |
OBz |
|
|
||
|
O |
|
|||
2. Et3N, CH2Cl2 |
|
BzO |
|
||
|
|
BzO |
O |
|
|
|
|
|
OBz |
|
|
|
|
|
NO2 |
|
|
|
|
|
10 |
|
|
|
|
|
|
OH |
|
|
AcO |
BnO |
O |
|
|
|
AcO |
O |
O |
O |
|
|
AcO |
OAc |
OBz |
|
|
|
|
BzO |
||
|
|
|
|
|
BzO |
|
|
|
OH |
|
|
|
|
BzO |
|
O |
O |
|
|
BzO |
|
OBz |
|
|
|
|
|
|
|
|
|
AcO |
BnO |
O |
|
|
|
AcO |
O |
O |
O |
|
|
AcO |
OAc |
OBz |
|
|
|
|
BzO |
||
|
|
|
|
|
BzO |
|
|
OTBDPS |
|
|
|
BnO |
O |
O |
|
|
|
|
HO |
|
|
|
|
|
OBz |
|
|
|
|
|
|
|
|
|
|
|
|
BzO |
|
O |
O |
|
|
BzO |
|
OBz |
|
|
|
|
|
|
|
|
|
AcO |
BnO |
O |
|
|
|
AcO |
O |
O |
O |
|
|
AcO |
OAc |
OBz |
|
|
|
|
BzO |
||
|
|
|
|
|
BzO |
|
|
OH |
|
|
|
BnO |
O |
O |
|
|
|
O |
O |
OBz |
|
|
|
OAc |
|
|
|
||
BzO |
|
O |
O |
||
|
|
BzO |
|
OBz |
|
|
|
|
|
|
|
|
|
AcO |
BnO |
O |
|
|
|
AcO |
O |
O |
O |
|
|
AcO |
OAc |
OBz |
|
|
|
|
BzO |
||
|
OAc |
|
|
|
BzO |
|
|
|
|
|
|
|
O |
O |
|
|
|
|
|
|
|
|
|
|
OAc |
|
|
|
|
BnO |
O |
O |
|
|
|
O |
O |
OBz |
|
|
|
OAc |
|
|
|
||
BzO |
|
O |
O |
||
|
|
BzO |
|
OBz |
|
|
|
|
|
|
|
|
|
AcO |
BnO |
O |
|
|
|
AcO |
O |
O |
O |
|
|
AcO |
OAc |
OBz |
|
|
|
|
BzO |
||
|
|
|
|
|
BzO |
1.3, DMTST
2.HF•py
O O
O
O
OBz
11NO2
1.1, DMTST
2.HF•py
O O
O
O
OBz
NO2
12
1.2, DMTST
2.Et3N, CH2Cl2
O O
O
O
OBz
13NO2
1.3, DMTST
2.HF•py
O O
O
O
OBz
NO2
14
3, DMTST
O O
O
O
OBz
NO2
15
Scheme 5.3 Stepwise assembly of the solid-phase-bound heptasaccharide 15 using thioglycosides as glycosyl donors and DMTST as activator.29

104 SYNTHESIS OF OLIGOSACCHARIDES ON SOLID SUPPORT
HO
HO
HO
HO
HO
1.hν (20% from 8)
2.NaOMe, MeOH, THF
15
3.H2, Pd-C (95%, 2 steps)
OH |
|
|
|
|
O |
O |
|
|
|
|
|
|
|
|
OH |
|
|
|
|
HO |
O |
O |
|
|
O O |
OH |
|
|
|
OH |
|
|
|
|
HO |
O |
O |
|
|
|
HO |
OH |
|
|
|
|
|
|
|
|
HO |
HO |
O |
O |
|
HO |
O O |
OH |
|
|
HO |
OH |
O |
|
|
|
HO |
||
|
|
|
HO |
OH |
|
|
|
|
OH |
16 (Heptasaccharide Phytoalexin Elicitor)
Scheme 5.4 Photolytic cleavage of resin-bound heptasaccharide 15 and deprotection to yield the heptasaccharide phytoalexin elicitor 16.29
consists of seven β-(1→6) and β-(1→3) linked glucose units and has two branch points. Three monosaccharide building blocks were selected for linear chain elongation (1), establishing the branch points (2), and termination (3), respectively (Scheme 5.2). These thioglycosides were activated by DMTST throughout the whole synthesis. Acyl protecting groups at C2 assured stereoselective formation of β-glycosides through neighboring-group participation. For attaching the saccharide to the solid support, a new photolabile linker with improved cleavage properties compared to published linkers44–46 was constructed. Readily available o-nitrobenzyl alcohol 6 was glycosylated with thioglycoside 1 and then coupled to phenolic polystyrene 5, which had been prepared from polystyrene 4. Irradiation of 8 liberated the attached monosaccharide in high yield and demonstrated the efficiency of the photocleavage. The assembly of the target oligosaccharide proceeded in a stepwise manner as depicted in Scheme 5.3. HF-pyridine complex was used to remove the silyl protecting groups, whereas the Fmoc group at the 3 position of the branch point building block 2 was selectively cleaved with triethylamine in dichloromethane. The obtained support-bound heptasaccharide 15 was photolytically cleaved from the resin to furnish the protected saccharide with free reducing end in a 20% overall yield from 8 as mixture of α- and β-anomers (Scheme 5.4). The targeted heptasaccharide 16 was finally obtained after deacylation followed by hydrogenolysis.
A problem associated with solid-phase synthesis is the characterization of
resin-bound reaction products. Although spectroscopic solutions to this problem have been published,34,47–53 the commonly applied procedure is to cleave the product from
a small resin sample and subsequently analyze the product in solution. However, if saccharides with free reducing end are generated in this step, mixtures of anomers are obtained, aggravating the NMR spectroscopic analysis, as was the case for the HPE 16. Another drawback of the solid support synthesis of 16 is the fact that cleavage products first have to be activated unless they can themselves be coupled to the support-bound growing oligosaccharide chain. With respect to the composition of 16 of repeating trisaccharides, such an approach would be more efficient than the stepwise coupling of monosaccharide building blocks.

5.2 THIOGLYCOSIDES AS GLYCOSYL DONORS 105
With the solid-phase synthesis of a dodecasaccharide, Nicolaou et al. presented a chemical solution to both problems mentioned above.30 As shown in Scheme 5.5, they incorporated 4-hydroxybenzoic acid as spacer between the photolabile linker and the anomeric position of the first saccharide (17). If 17 (or an analogously immobilized larger oligosaccharide) is photolytically cleaved, stereochemically homogeneous β-product 18 (or the analogous oligosaccharide derivative) is obtained, which is ideally suited for analytical investigations. Cleavage from the solid support can also be effected with concomitant activation of the released saccharide under conditions originally described by Hanessian54 for the cleavage of O-glycosides as shown for the conversion 17→19. The thioglycosides thus obtained can readily be used in further (solid-phase) glycosylation reactions.
Scheme 5.6 demonstrates the application of this strategy to the solid-phase block synthesis of the phytoalexin elicitors related dodecasaccharide 28.30 Commencing with 17, two cycles of deprotection and DMTST promoted coupling of a thioglycoside led via 20 and 21 to the resin-bound trisaccharide 22. Photolysis of a resin sample gave stereochemically pure trisaccharide 23 suitable for NMR spectroscopic analysis. A part of 22 was desilylated with HF-pyridine complex. Another portion of 22 was treated with TMS-SPh, ZnI2, and n-Bu4NI to give trisaccharide thioglycoside 24, which was subsequently coupled to deprotected 22 under DMTST activation to furnish resin-bound hexasaccharide 25. Two more cycles consisting of desilylation and DMTST activated glycosylation with trisaccharide 24 gave, via 26, solid-phase bound dodecasaccharide 27. Photolytic cleavage finally yielded the fully protected dodecasaccharide 28 as a single stereoisomer (~10% yield from 17).
|
|
|
|
O |
|
|
|
|
OTBDPS |
O |
|
|
|
|
BzO |
O |
O |
NO2 |
|
|
|
BzO |
OBz |
|
|
||
|
|
O |
17 |
|
|
|
|
|
|
|
|
||
Cleavage |
|
|
|
|
Activation |
|
|
hν, THF |
95% |
|
92% |
TMS-SPh |
|
|
|
|
|
|
ZnI2, n-Bu4NI |
|
|
OTBDPS |
OH |
|
|
OTBDPS |
|
BzO |
O |
O |
|
BzO |
|
O |
BzO |
|
|
BzO |
SPh |
||
|
OBz |
O |
|
|
|
OBz |
|
|
|
19 (α : β = 1 : 1) |
|||
|
18 |
|
Scheme 5.5 Structure and properties of the photolabile linker 17 used for solid-phase synthesis of dodecasaccharide 28.30
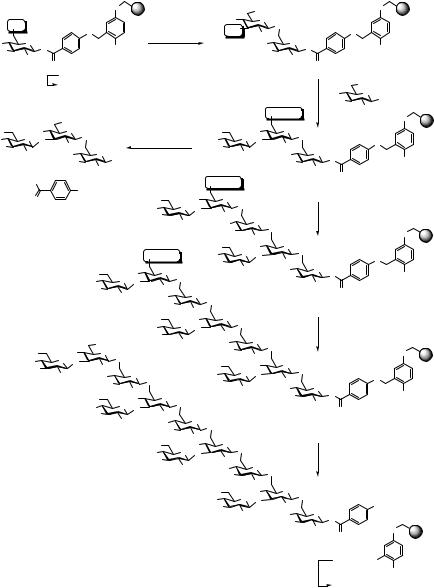
106 SYNTHESIS OF OLIGOSACCHARIDES ON SOLID SUPPORT
|
|
|
|
O |
|
|
|
|
|
|
OTBDPS |
|
|
|
O |
|
|
|
|
|
|
|
|
|
|
|
|
|
|
|
|
||
|
OR |
|
|
|
|
|
|
|
BnO |
|
O |
O |
|
|
|
|
|
|
O |
|
|
1. 2, DMTST |
HO |
|
|
O |
|
|
|||||
|
|
|
|
|
OBz |
|
|
|
|
|||||||
|
O |
|
|
|
2. Et3N, THF |
|
|
O |
|
|
|
|||||
BzO |
|
|
|
|
|
|
BzO |
|
|
|
|
|||||
|
|
NO2 |
|
|
|
|
|
|
|
|
|
NO2 |
||||
BzO |
O |
|
|
|
90% |
|
|
|
BzO |
O |
|
|
|
|||
|
OBz |
|
|
|
|
|
|
|
|
OBz |
|
|
|
|
||
|
O |
|
|
|
|
|
|
|
|
|
O |
|
|
|
||
|
|
|
|
|
|
|
|
|
|
|
|
21 |
|
|
||
|
|
17: R = TBDPS |
|
|
|
|
|
|
|
|
|
|
|
|
||
|
HF•py, THF |
|
|
|
|
|
|
|
|
|
|
|
OBn |
|
||
|
20: R = H |
|
|
|
|
|
|
|
|
|
|
|
|
|||
|
|
|
|
|
|
|
|
|
|
|
|
|
|
|||
|
|
|
|
|
|
|
|
|
|
|
|
78% |
|
BnO |
O |
SPh |
|
|
|
|
|
|
|
|
|
|
|
|
|
|
BnO |
|
|
|
|
|
|
|
|
|
|
|
|
|
OTBDPS |
|
|
OBz |
||
|
|
|
|
|
|
|
|
|
|
|
|
DMTST, TMU |
O |
|||
|
|
OTBDPS |
|
|
|
|
|
|
|
|
|
|
||||
|
|
|
|
|
|
|
|
|
|
|
|
|
|
|
||
BnO |
BnO |
O |
O |
|
22→23: |
|
|
BnO |
BnO |
O |
|
|
|
|
||
BnO |
O O |
|
|
hν, THF |
|
|
BnO |
O |
O |
O |
|
|
|
O |
||
OBz |
|
|
|
OBz |
|
|
|
|||||||||
BnO |
|
|
|
|
|
|
BnO |
|
|
|
|
|
||||
|
OBz |
BzO |
O |
R |
22→24: |
|
|
|
OBz |
BzO |
O |
O |
|
NO2 |
||
|
|
BzO |
|
TMS-SPh, ZnI2 |
|
|
|
|
BzO |
|
|
|||||
|
|
|
OBz |
|
|
|
|
|
|
OBz |
|
|
||||
23: R = O |
|
|
|
|
|
|
|
OTBDPS |
|
|
|
|
O |
|
|
|
|
OH |
|
|
|
|
|
|
|
|
22 |
|
|
|
|||
|
|
|
|
|
|
|
|
|
|
|
|
|
|
|
|
|
|
O |
|
|
|
|
BnO |
BnO |
O |
O |
|
|
|
|
|
|
|
|
(63% from 17) |
|
|
|
BnO |
O |
O |
OBz |
|
|
|
1. HF•py |
|
|
||
|
|
|
|
|
|
BnO |
|
|
|
|
61% |
|
|
|
||
24: R = SPh (76%, α/β-mixture) |
|
|
|
OBz |
BzO |
|
O |
|
2. 24, DMTST |
|
||||||
|
|
|
|
|
|
|
||||||||||
|
|
|
|
|
|
|
|
|
BzO |
|
O |
|
|
|
|
O |
|
|
|
|
|
|
|
|
|
|
|
OBz |
|
|
|
|
|
|
|
|
|
|
|
|
|
|
|
|
|
|
|
|
|
|
|
|
|
|
|
|
|
|
|
BnO |
BnO |
O |
|
|
|
|
|
|
|
|
|
|
|
OTBDPS |
|
|
BnO |
O |
O |
O |
|
|
|
O |
|
|
|
|
|
|
|
|
|
BnO |
OBz |
OBz |
O |
|
|
||
|
|
|
BnO |
BnO |
O |
|
|
|
BzO |
O |
|
NO2 |
||||
|
|
|
O |
|
|
|
|
BzO |
|
|
||||||
|
|
|
BnO |
O |
O |
|
|
|
|
|
|
OBz |
|
|
||
|
|
|
BnO |
|
|
OBz |
|
|
|
|
|
|
|
|
||
|
|
|
OBz |
|
O |
|
|
|
|
|
O |
|
|
|||
|
|
|
|
BzO |
|
|
|
|
|
|
|
|
||||
|
|
|
|
|
O |
|
|
|
25 |
|
|
|
||||
|
|
|
|
|
|
BzO |
|
|
|
|
|
|
|
|
||
|
|
|
|
|
|
|
|
OBz |
|
|
|
|
|
|
|
|
|
|
|
|
|
|
BnO |
BnO |
O |
O |
|
|
|
|
|
|
|
|
|
|
|
|
|
BnO |
O |
O |
|
|
|
|
1. HF•py |
|
|
|
|
|
|
|
|
|
OBz |
|
|
|
|
|
|
||||
|
|
|
|
|
|
BnO |
OBz |
|
O |
|
|
2. 24, DMTST |
|
|||
|
|
|
OTBDPS |
|
|
BzO |
|
|
|
|
||||||
|
|
|
|
|
|
|
|
|
|
|
||||||
|
BnO |
BnO |
O |
|
|
|
|
|
BzO |
|
O |
|
|
|
|
O |
|
O |
|
|
|
|
|
|
OBz |
|
|
|
|
||||
|
BnO |
O O |
|
|
|
|
|
|
|
|
|
|
|
|
||
|
|
|
|
|
|
BnO |
BnO |
O |
|
|
|
|
||||
|
BnO |
|
OBz |
|
|
|
|
|
|
|
|
|||||
|
|
OBz |
BzO |
|
O |
|
|
|
BnO |
O |
O |
O |
|
|
|
O |
|
|
|
O |
|
|
OBz |
|
|
|
|||||||
|
|
|
BzO |
|
|
|
|
BnO |
OBz |
O |
|
|
||||
|
|
|
|
|
OBz |
|
|
|
BzO |
O |
|
NO2 |
||||
|
|
|
BnO |
BnO |
O |
|
|
|
|
|
BzO |
|
|
|||
|
|
|
O |
|
|
|
|
|
OBz |
|
|
|||||
|
|
|
BnO |
O |
O |
|
|
|
|
|
|
|
|
|||
|
|
|
|
|
|
|
|
|
|
|
O |
|
|
|||
|
|
|
BnO |
|
|
OBz |
|
|
|
|
|
|
|
|
|
|
|
|
|
OBz |
|
O |
|
|
|
|
|
|
|
|
|||
|
|
|
|
BzO |
|
O |
|
|
|
26 |
|
|
|
|||
|
|
|
|
|
|
BzO |
|
|
|
|
|
|
|
|
|
|
|
|
|
|
|
|
|
|
OBz |
|
|
|
|
|
|
|
|
|
|
|
|
|
|
BnO |
BnO |
O |
O |
|
|
|
|
|
|
|
|
|
|
|
|
|
BnO |
O |
O |
OBz |
|
|
|
1. HF•py |
|
|
|
|
|
|
|
|
|
BnO |
OBz |
|
O |
|
|
2. 24, DMTST |
|
|||
|
|
|
|
|
|
|
BzO |
|
|
|
|
|||||
|
|
|
|
|
|
|
|
|
|
|
|
|
||||
|
|
|
|
|
|
|
|
|
BzO |
|
O |
|
|
|
|
|
|
|
|
|
|
|
|
|
|
|
|
OBz |
|
|
|
|
|
|
|
|
|
|
|
|
|
|
BnO |
BnO |
O |
|
|
|
|
|
|
|
|
|
|
|
|
|
|
BnO |
O |
O |
O |
|
|
|
OR |
|
|
|
|
|
|
|
|
|
BnO |
OBz |
OBz |
O |
|
|
||
|
|
|
|
|
|
|
|
|
|
BzO |
O |
|
|
|||
|
|
|
|
|
|
|
|
|
|
|
|
BzO |
|
|
|
|
|
|
|
|
|
|
|
|
|
|
|
|
|
OBz |
|
O |
|
|
|
|
|
|
|
|
|
|
|
|
|
|
|
O |
|
|
|
|
|
|
|
|
|
|
|
|
|
|
|
|
27: R = CH2 |
||
|
|
|
|
|
|
|
|
|
|
|
|
hν, THF |
|
|
|
NO2 |
|
|
|
|
|
|
|
|
|
|
|
|
|
|
28: R = H (10% from 17) |
Scheme 5.6 Solid-phase assembly of dodecasaccharide 2830 (TMU = tetramethylurea).

5.3 PENTENYL GLYCOSIDES AS GLYCOSYL DONORS 107
5.3 PENTENYL GLYCOSIDES AS GLYCOSYL DONORS
The use of n-pentenyl glycosides (NPGs) as glycosyl donors55,56 was introduced to carbohydrate chemistry in 1988 by Fraser-Reid et al.57 and was based on the observation of an unexpected side reaction during the synthesis of streptovaricin A. As with the thioglycosides, NPGs can serve both as protection and activation of the anomeric hydroxyl group. Their preparation55,56 can be carried out by standard procedures for making simple alkyl glycosides. Particularly, it is possible to obtain NPGs from aldoses with 4-pentenyl alcohol and camphorsulfonic acid under slightly modified Fischer glycosidation conditions.58 However, for some sugars, such as galactose and glucosamine, the Fischer glycosidation gives poor yields. In these instances, NPGs can be prepared from glycosyl bromides or chlorides under Koenigs–Knorr conditions or from glycosyl acetates under Lewis acid catalysis. Alternatively, the synthesis of NPGs via pentenyl 1,2-orthoesters has been described.59 The advantage of using orthoesters is that several base-promoted protecting group manipulations can be carried out before the acid-induced rearrangement to an NPG occurs, leaving an acyl protecting group at C2. Related to n-pentenyl glycosides are glycosyl 4-pentenoates, which have been described by Kunz et al.60
5.3.1 Activation Procedures
The activation of NPGs during a glycosylation reaction (Scheme 5.7a) depends on electrophilic addition to the olefin (→III), followed by intramolecular displacement by the anomeric oxygen to form the oxonium species IV. Trapping with a glycosyl
(a) |
|
|
E |
|
E |
|
|
|
|
|
|
O |
O |
E+ |
O O |
|
O O |
|
|
III |
|
|
IV |
|
|
|
O |
OR |
R–OH |
|
|
|
|
|
|
|
|
|
|
– |
O |
|
|
|
|
E |
|
(b) |
|
|
|
|
|
Et3Si |
OTf |
|
|
|
|
O |
|
O |
SiEt3 |
|
|
N |
I |
N |
+ |
I+ TfO– |
|
O |
|
O |
|
|
|
Scheme 5.7 (a) Mechanism of the n-pentenyl glycoside–based glycosylation method. Electrophiles commonly employed include iodonium di-sym-collidine perchlorate (IDCP) and N-iodosuccinimide/triethylsilyl triflate (NIS/Et3SiOTf); (b) Mechanism for the activation of NIS by Et3SiOTf.55