
Solid-Phase Synthesis and Combinatorial Technologies
.pdfREFERENCES 43
130.Stones, D., Miller, D. J, Beaton, M. W., Rutherford, T. J. and Gani, Tetrahedron Lett. 39, 4875–4878 (1998).
131.Johnson, C. R. and Zhang, B., Tetrahedron Lett. 36, 9253–9256 (1995).
132.Swayze, E. E., Tetrahedron Lett. 38, 8643–8646 (1997).
133.Look, G. C., Holmes, C. P., Chinn, J. P. and Gallop, M. A., J. Org. Chem. 59, 7588–7590 (1994).
134.Holmes, C. P., J. Org. Chem. 62, 2370–2380 (1997).
135.Bam, D. R., Morphy, J. R. and Rees, D. C., Tetrahedron Lett. 37, 3213–3216 (1996).
136.Marchioro, C. and Missio A., personal communication, March 1998.
137.Muddiman, D. C., Bakhtiar, R., Hofstadler, S. A. and Smith, R. D., J. Chem. Educ. 74, 1288–1292 (1997).
138.Zambias, R. A., Boulton, D. A. and Griffin, P. R., Tetrahedron Lett. 35, 4283 (1994).
139.Egner, B. J., Langley, G. J. and Bradley, M., J Org. Chem. 60, 2652–2653 (1995).
140.Haskins, N. J., Hunter, D. J., Organ, A. J., Rahman, S. S. and Thom, C., Rapid Commun. Mass Spectrom. 9, 1437–1440 (1995).
141.Egner, B. J. and Bradley, M., Tetrahedron 41, 14021–10430 (1997).
142.Egner, B. J., Cardno, M. and Bradley, M., J. Chem. Soc., Chem. Commun. 2163–2164 (1995).
143.Fitzgerald, M. C., Harris, K., Shevlln, C. G. and Siuzdak, G., Bioorg. Med. Chem. Lett. 6, 979–982 (1996).
144.Benninghoven, A., Wemer, H. W. and Rudenauer, F. G.,Secondary Ion Mass Spectrometry, Basic Concepts, Instrumental, Aspects, Application and Trends . John Wiley, New York, 1986.
145.Enjabal, C., Tetrahedron Lett. 40, 6217–6220 (1999).
146.Geysen, H. M., Wagner, C. D., Bodnar, W. M., Markworth, C. J., Parke, G. J., Schoenen,
F.J., Wagner, D. S. and Kinder, D. S., Chem. Biol. 3, 679–688 (1996).
147.Carrasco, M. R., Fitzgerald, M. C., Oda, Y. and Kent, S. B. H., Tetrahedron Lett. 38, 6331–6334 (1997).
148.McKeown, S. C., Watson, S. P., Caff, R. A. E. and Marshall, P., Tetrahedron Lett. 40, 2407–2410 (1999).
149.Murray, P. J., Kay, C., Scicinski, J. J., McKeown, S. C., Watson, S. P. and Carr, R. A. E., Tetrahedron Lett. 40, 5609–5612 (1999).
150.Chen, C., Randall, L. A. A., Miller, R. B., Jones, A. D. and Kurth, M. J., J. Am. Chem. Soc. 116, 2661–2662 (1994).
151.Hauske, J. R. and Dorff, P., Tetrahedron Lett. 36, 1589–1592 (1995).
152.Yan, B., Kumaravel, G., Anjaria, H., Wu, A., Petter, R. C., Jewell, C. F. Jr., Wareing, J. R.,
J.Org. Chem. 60, 5736–5738 (1995).
153.Yan, B. and Kumaravel, G., Tetrahedron 52, 843–848 (1996).
154.Yan, B. and Li, W., J. Org. Chem. 62, 9354–9357 (1997).
155.Yan, B., Sun, Q., Wareing, J. R. and Jewell, C. F., J. Org. Chem. 61, 8765–8770 (1996).
156.Yan, B., Liu, L., Astor, C. A. and Tang, Q., Anal. Chem. 71, 4564–4571 (1999).
157.Pivonka, D. E., J. Comb. Chem. 1, 294–296 (1999).
158.Pivonka, D. E., Palmer, D. L. and Gero, T. W., Appl. Spectrosc. 53, 1027–1032 (1999).
44SOLID-PHASE SYNTHESIS: BASIC PRINCIPLES
159.Haap, W. J., Kaiser, D., Walk, T. B. and Jung, G., Tetrahedron 54, 3705–3724 (1998).
160.Huber, W., Bubendorf, A., Grieder, A. and Obrecht, D., Anal. Chim. Acta 393, 213–221 (1999).
161.Rosse’, G., Ouertani, F. and Schroder, H., J. Comb. Chem. 1, 397–401 (1999).
162.Gosselin, F., Di Renzo, M., Ellis, T. H. and Lubell, W. D., J. Org. Chem. 61, 7980–7981 (1996).
163.Chan, T. Y., Chen, R., Sofia, M. J., Smith, B. C. and Glennon, D., Tetrahedron Lett. 38, 2821–2824 (1997).
164.Deben, I., Goorden, J, Van Doomum, E., Ovaa, H. and Kellenbach, E.,Eur. J. Org. Chem., 697–700 (1998).
165.Hammond, J., Moffat, A. C., Jee, R. D. and Kellam, B., Anal. Commun. 36, 127–129 (1999).
166.Fischer, M. and Tran, C. D., Anal. Chem. 71, 2255–2261 (1999).
167.Yan, B., Gremlich, H.-U., Moss, S., Coppola, G. M., Sun, Q., and Liu, L.,J. Comb. Chem. 1, 46–54 (1999).
168.Rahman, S. S., Busby, D. J. and Lee, D. C., J. Org. Chem. 63, 6196–6199 (1998).
169.Yan, B. and Gremlich, H.-U., J. Chromatogr., B: Biomed. Sci. Applic. 725, 91–102(1999).
170.Hochlowski, J., Whittem, D., Pan, J. and Swenson, R.,Drugs Future 24, 539–554 (1999).
171.Luo, Y., Ouyang, X., Armstrong, R. W. and Murphy, M. M.,J. Org. Chem. 63, 8719–8722 (1998).
172.Andrew, E. R., Prog. Nucl. Magn. Reson. Spectrosc . 8, 1–39 (1971).
173.Fitch, W. L., Detre, G., Holmes, C. P., Shoolery, J. N. and Keifer, P. A.,J. Org. Chem. 59, 7955–7956 (1994).
174.Kempe, M., Keifer, P. A. and Barany, G., inProceedings from the Twenty-Fourth European Peptide Symposium, R. Rampage and R. Epton (Eds.). Edinburgh, Scotland, September 8th–13th, 1996. Mayflower Scientific Ltd., West Midland, 1998, pp. 533–534.
175.Keifer, P. A., J. Org. Chem. 61, 1558–1559 (1996).
176.Shapiro, M. J. and Wareing, J. R., Curr. Opin. Chem. Biol. 2, 372–375 (1998).
177.Lippens, G., Bourdonneau, M., Dhalluin, C., Waffass, R., Richert, T., Seetharaman, C., Boutillon, C. and Piotto, M., Curr. Org. Chem. 3, 147–169 (1999).
178.Riedl, R., Tappe, R. and Berkessel, A., J. Am. Chem. Soc. 120, 8994–9000 (1998).
179.Warrass, R., Wieruszeski, J.-M. and Lippens, G., J. Am. Chem. Soc. 121, 3787–3788 (1999).
180.Missio, A. and Marchioro, C., personal communication, March 1998.
181.Chin, J., Fell, B., Pochapsky, S., Shapiro, M. J. and Wareing, J. R., J. Org. Chem. 63, 1309–1311 (1998).
182.Sarkar, S. K., Garigipati, R. S., Adams, J. L. and Keifer, P. A., J. Am. Chem. Soc. 118, 2305–2306 (1996).
183.Pursch, M., Schlotterbeck, G., Tseng, L.-H., Albert, K. and Rapp, W.,Angew. Chem., Int. Ed. Engl. 35, 2867–2868 (1996).
Solid-Phase Synthesis and Combinatorial Technologies. Pierfausto Seneci Copyright © 2000 John Wiley & Sons, Inc.
ISBNs: 0-471-33195-3 (Hardback); 0-471-22039-6 (Electronic)
2Solid-Phase Synthesis: Oligomeric Molecules
Thefirst preparation of abiologically relevant oligopeptide on asolidsupportby Merrifield
(1) represented a landmark, not only for the field of SPS but also for organic chemistry as a whole. The use of this technique to prepare oligomeric molecules by repeated use of the same type of chemical reaction applied to similar building blocks rapidly gained popularity. The advantages of oligomeric SPS versus solution-phase synthesis of the same oligomers are self-evident: tedious and troublesome purifications after each step are avoided and the repetitive nature of the chemistry lends itself to automation of the process. Excellent yields are obtained by forcing the reactions to completion through the use of large excesses and/or high concentration of reagents in solution.
Peptides are the single most exploited oligomeric class of molecules in SPS, and the last 35 years have seen significant improvements in all aspects of SP peptide synthesis. The SPS of peptoids, peptidomimetics, peptide hybrids, and cyclic peptides has also gained in importance because of their improved properties when compared with natural peptides (e.g., stability and solubility). Oligonucleotides represent the other class of biologically important molecules in which considerable progress has been made in automated synthesis on SP The field of combinatorial chemistry started and, for quite some time, remained strictly related to the SPS of peptides and to some extent oligonucleotides. Oligosaccharides have also been considered as targets for synthesis in the SP; however, their complex structures makes the chemistry of this class much more challenging due to problems of regioand chemoselectivity as well as availability of suitable building blocks. Even today, this class remains underexploited.
In this chapter, a historical overview of the SPS of peptides, nucleotides, oligosaccharides, and related oligomeric molecules will be provided, summarizing the main efforts in this area. The strategy followed in designing and carrying out the SPS of an oligomeric biopolymer will be presented and discussed through the use of examples, and eventually, it will be compared with the strategy used for the SPS of small organic molecules (see Chapter 3).
2.1 PEPTIDES
2.1.1 Solid-Phase Synthesis of Peptides
The number of peptides prepared on SP since the pioneering work by Merrifield in the early 1960s is huge, and the relevance of peptide SPS is such that various books and
45
46 SOLID-PHASE SYNTHESIS: OLIGOMERIC MOLECULES
reviews extensively covered this topic. The interested reader is referred to a recent book (2) that covers the main aspects of peptide SPS to expand some of the basic concepts provided in this chapter.
The scheme of classical peptide SPS is shown in Fig. 2.1. The first step is the attachment of the first amino acid onto the resin, usually via the carboxylic group with both the amino and the side-chain functions of the α-amino acid protected (step 1). The inverse strategy, where the amino group would be linked to the resin and the carboxyl would be protected, has seldom been used because extensive racemization occurs during repeated resin-bound carboxylate activations (3); this limits the accessibility of C-terminal modified peptides that are biologically relevant. However, several recent reports (4–7) describe the SP modification of the peptide C-terminus using the more reliable C-to-N direction for peptide synthesis.
The synthetic scheme consists of repeated deprotections of the amino terminus followed by coupling of an N-protected amino acid (with side chains suitably protected) in solution until the target peptide is obtained. A single cycle (step 2) is detailed in Fig. 2.1, while the following cycles are identical. After the assembly of the fully protected peptide, all the side chains are deprotected and the peptide is released into solution via cleavage of the linker (Fig. 2.1). The repetitive nature of the deprotection/coupling steps where an amino-protecting group is removed and an amide bond is formed does not require any assessment of the chemistry since it is so well-known. This allows the rapid synthesis of long oligopeptides with excellent yields and no detectable resin-bound side products. The robustness of the peptide amidic bond significantly reduces the number and the importance of side reactions.
The synthesis of natural oligopeptides (of nonbacterial origin) of various lengths requires only 20 building blocks, the natural L-amino acids. They are commercially available with a large variety of orthogonal protecting groups on the amino function and on the functionalized side chains, which can be removed selectively without affecting other protecting groups present. This allows many possible protecting group strategies to be followed, depending on the linker and the chemistry to be used.
Two major protecting group strategies (see Fig. 2.1) are used for P1 in SP peptide synthesis. The Boc protocol (8) involves the use of N-Boc-protected amino acids and the iterative deprotections are performed with TFA; the Fmoc protocol (9) employs N-Fmoc-protected amino acids and the iterative deprotections are performed with 20% v/v piperidine in DMF.
The use of Boc as N-protecting group allows the anchoring of the first amino acid onto the Merrifield resin through a benzyl ester bond. Final cleavage with HF or hydrogenolysis gives the peptide acid or, alternatively, the C-terminal amide is obtained through ammonolysis. Groups in the side chains are protected with benzylbased protecting groups (e.g., 2-chloroCBz for Tyr, 4-methylbenzyl for Cys), which are removed during the hydrogenolytic cleavage. Amine linkers, such as the 4-methyl- benzhydrylamine (MBHA, benzyl amide bond), have also been used to release peptide primary amides.
The older Boc protocol has largely been superceded by the Fmoc protocol, which has several relevant advantages over it. The use of milder deprotection conditions for the Fmoc group and its complete orthogonality with a large number of side-chain
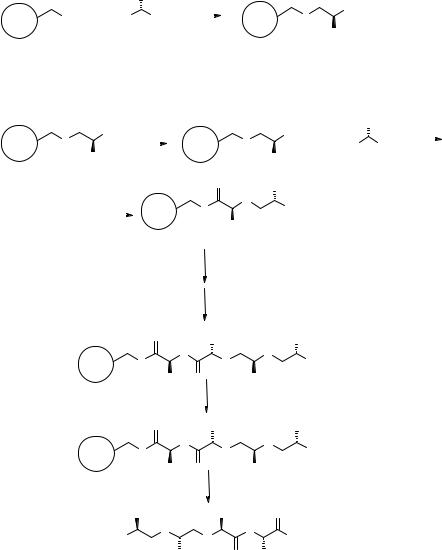
|
|
|
|
|
|
|
|
|
|
|
|
2.1 |
PEPTIDES 47 |
||||
|
|
|
|
|
|
R(P2) |
a |
|
|
O |
|
|
|
||||
|
|
|
|
|
|
|
NHP1 |
||||||||||
|
|
|
|
|
|
|
|
|
|
|
|
||||||
L + |
|
|
|
|
|
|
|
|
|
|
|
||||||
|
HOOC NHP1 |
|
|
|
|
L |
|
|
|
||||||||
|
|
|
|
|
|
|
|||||||||||
|
|
|
|
|
|
|
|
|
|
|
|
|
|
R(P2) |
|
|
|
|
|
|
|
|
|
|
|
step 1 |
|
|
|
|
|
|
|
|
|
O |
|
|
|
|
|
|
|
O |
|
|
|
R(P2) |
|||||
|
|
|
|
|
|
b |
|
|
|
|
|
|
|
||||
L |
|
NHP1 |
|
|
L |
NH2 + |
|
|
|
||||||||
|
|
|
|
|
|||||||||||||
|
|
|
|
|
|
|
|
|
|
|
|
|
|
HOOC |
NHP1 |
||
|
|
|
R(P2) |
|
|
|
|
|
|
||||||||
|
|
|
|
|
|
|
R(P2) |
|
|
|
|||||||
|
|
|
|
|
|
|
|
|
O |
H |
R(P2) |
|
|
|
|||
|
|
|
|
|
|
|
|
|
|
|
|
|
|
|
|
||
|
|
|
|
c |
L |
N |
NHP1 |
|
|
|
|||||||
|
|
|
|
|
|
|
|
|
|
|
|
|
|||||
|
|
|
|
|
|
|
|
step 2 |
R(P2) O |
|
|
|
|
|
|
||
|
|
|
|
|
|
|
|
|
|
|
|
|
|
|
|
||
L = linker |
|
|
|
|
|
|
|
|
|
|
|||||||
a) C-terminus coupling with the linker; |
step 3 |
|
|
|
|
|
|
b)P1 deprotection;
c)C-terminus amide coupling;
d) P2 deprotection; |
|
|
step n |
|
|
|
|
|||||||||
e) cleavage from the resin. |
|
|
|
|
|
|
|
|
|
|
|
|
||||
|
|
|
|
|
|
|
|
|
|
|
|
|
|
|
R(P2) |
|
|
O |
|
|
R(P2) |
|
O |
H |
|
||||||||
|
|
|
|
H |
|
|
|
|
|
|
|
|
|
|
||
|
|
|
|
N |
|
|
|
|
|
|
|
|
N |
|
NHP1 |
|
L |
|
|
|
N |
|
|
|
|
|
|
|
|||||
|
|
|
|
|
|
|||||||||||
|
|
|
|
R(P2) O |
H |
|
|
|
|
|
|
|
|
|||
|
|
|
|
|
|
|
|
|
R(P2) |
O |
||||||
|
|
|
|
|
|
|
b,d |
|
|
|
|
|
|
|
|
|
|
|
|
|
|
|
|
|
|
|
n-3 |
||||||
|
|
|
|
|
|
|
|
|
|
|
|
|
|
|
R |
|
|
O |
|
|
R |
|
|
O |
|
|
|
||||||
|
|
|
|
H |
|
|
|
|
|
|
|
|
H |
|
||
|
|
|
|
N |
|
|
|
|
|
|
|
|
N |
|
||
|
|
|
|
|
|
|
|
|||||||||
L |
|
|
|
N |
|
|
|
|
|
NH2 |
||||||
|
|
|
|
|
|
|||||||||||
|
|
|
|
R |
|
O |
H |
|
|
R |
|
|
||||
|
|
|
|
|
|
|
|
|
|
O |
||||||
|
|
|
|
|
|
|
|
|
|
|
|
|
||||
|
|
|
|
|
|
|
e |
|
|
n-3 |
||||||
|
|
|
|
|
|
|
|
|
||||||||
R |
|
|
O |
|
R |
|
O |
|||||||||
|
|
|
H |
|
|
|
|
|
|
|
|
H |
||||
H2N |
|
|
N |
|
|
|
|
|
|
|
|
N |
||||
|
|
|
|
|
|
|
|
|||||||||
|
|
|
|
|
|
N |
|
|
|
|
|
|
|
|
OH |
|
|
|
|
|
|
|
|
|
|
|
|
|
|
||||
|
|
|
|
R |
|
|
H |
O |
R |
|||||||
O |
|
|
|
|
||||||||||||
|
|
|
|
|
|
|
n-3 |
|
|
|
|
|||||
|
|
|
|
|
|
|
|
|
|
|
Figure 2.1 SPS of peptides.
protecting groups gives a better quality synthesis. Many different acid-sensitive SP linkers can be used, and the milder conditions allow the preparation of modified peptides containing acid-sensitive building blocks such as glycosylated or sulfated or phosphorylated peptides. Finally, the Fmoc reading of the cleavage solution as described in Section 1.4.2 allows for the easy quantitation of the coupling steps.
48 SOLID-PHASE SYNTHESIS: OLIGOMERIC MOLECULES
Many solid supports have been used in the SPS of peptides, and all of them are compatible with Fmoc-based chemistry. Cross-linked hydrophobic and hybrid PSbased resins are among the most used supports, but much SPS of peptides is performed by the so-called continuous-flow methodology (10) where sealed columns contain the resin and the peptide is assembled using flowing solutions of reagents for each step. This method is easily automated and is used by commercially available SP peptide synthesizers. The columns typically contain nonswelling resins such as the macroporous supports POLYHYPE and Kieselguhr described in Section 1.1.3 (11, 12). PSbased devices such as pins (13) have also been used as alternative supports together with paper (14), cotton (15), and sepharose (16).
Acid-labile protecting groups for side-chain functionalities allow the simultaneous final deprotection together with the cleavage of the acid-labile linker to produce the free peptide in solution in a single step. The most widely used protecting groups are t-butyl ethers (Ser, Thr, Tyr) or esters (Glu, Asp) and Boc carbamates (Lys). Acid-labile linkers with different acid stability are chosen depending on the stability of the target peptide and on the functional group to be released on the C-terminus (e.g., free acid, amide, hydrazide, or hydroxamate). The so-called Backbone Amide Linkage (BAL)
(5) linker has recently found a wide application in SPS (17–19); a specific example will be described in details in Section 2.1.3. Examples of cyclative cleavage approaches to provide cyclic peptides or depsipeptides have also been reported (20).
The coupling conditions have been thoroughly studied and optimized. A lot of effort has gone into studying the influence of solvents and temperatures, the extent of racemization, and the best coupling reagents or carboxyl activating agents to use. An excellent review (21) summarizes the state-of-the-art and clearly shows the superior quality of peptide SPS. The synthesis of peptide 40mers with diverse structures using coupling cycles of just a few minutes and average coupling yields in excess of 99% can be considered a routine task. Further reduction of cycle times and improvement of their efficiency will probably allow the peptide SPS of even longer oligopeptides with lower quantities of impurities.
The quality of the SPS of a peptide can be determined by the analytical methods presented in Sections 1.3 and 1.4. Classical amino acid analyses following acid degradation (22), capillary electrophoresis (23), and MS-related protocols (24, 25) are also commonly employed. Qualitative monitoring of couplings can be carried out using either the ninhydrin test or bromophenol blue, which is particular for the secondary amino group of prolines. The yield of each iterative cycle of coupling and deprotection is given by the Fmoc reading. On-bead spectroscopic and spectrometric methods such as MALDI–TOF, FTIR, and MAS–HR NMR are also routinely used.
The sequencing of a peptide (26) uses the well-known Edman degradation (27) of peptides, as shown in Fig. 2.2. The reaction cycle consists of reaction with phenylisothiocyanate followed by treatment with anhydrous TFA to promote cyclization of the intermediate thiourea. Rearrangement induced by treatment with aqueous TFA produces the phenylthiohydantoin (PTH) of the N-terminal amino acid 2.2 and the truncated peptide 2.3. The sequence is repeated through n cycles until the whole peptide is degraded. The PTHs produced are detected by HPLC-UV and their retention times are compared to those of 20 standard PTHs, one for each natural amino acid.
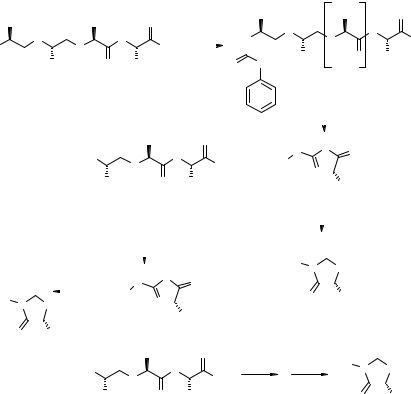
|
|
|
|
|
|
|
|
|
|
|
|
|
|
|
|
|
|
|
|
|
|
|
|
|
|
|
|
|
|
|
|
|
|
|
|
|
|
|
|
|
|
2.1 |
PEPTIDES 49 |
|||||||
|
|
|
|
|
|
|
|
|
|
|
|
|
|
|
|
|
|
|
|
|
|
|
|
|
|
|
|
|
|
|
|
|
|
|
Rn |
|
|
|
O |
|
|
|
R |
|
|
|
O |
|||
Rn |
|
|
|
|
O |
|
R |
|
|
|
|
|
|
|
|
|
O |
|
|
|
|
|
H |
|
|
|
||||||||||||||||||||||||
|
|
|
|
|
|
|
|
|
|
|
|
|
|
|
a |
|
|
|
|
|
|
|
|
|
|
|
H |
|
||||||||||||||||||||||
|
|
|
|
|
H |
|
|
|
|
|
|
|
|
|
H |
|
|
|
|
|
|
|
|
|
|
|
|
|
|
|
|
N |
|
|
|
|
|
|
|
N |
|
|||||||||
|
|
|
|
|
N |
|
|
|
|
|
|
|
|
|
N |
|
|
|
|
|
|
|
|
|
|
|
|
|
|
HN |
|
|
|
|
|
|
|
N |
|
|
|
|
|
OH |
||||||
H2N |
|
|
|
|
|
|
|
|
|
|
N |
|
|
|
|
|
|
|
|
|
|
OH |
|
|
|
|
|
|
|
|
|
|
|
|
H |
|
|
|
|
|
|
|||||||||
|
|
|
|
|
|
|
|
|
|
|
H |
|
|
|
|
|
|
|
|
|
|
|
|
|
|
|
|
|
|
|
|
|
O Rn-1 |
|
|
|
O |
|
|
R1 |
|
|||||||||
|
O |
|
|
|
Rn-1 |
|
R1 |
|
|
|
|
|
|
|
|
|
|
S |
|
|
|
|
|
|
||||||||||||||||||||||||||
|
|
|
|
|
O |
|
|
|
|
|
|
|
|
|
|
|
|
|
|
|
|
|
NH |
|
|
|
|
|
|
|
n-3 |
|
||||||||||||||||||
|
|
|
|
|
|
|
|
|
|
|
|
|
|
|
|
|
n-3 |
|
|
|
|
|
|
|
|
|
|
|
|
|
|
|
|
|
|
|
|
|
|
|
|
|||||||||
|
|
|
|
|
|
|
|
|
|
|
|
|
|
|
|
|
|
|
|
|
|
|
|
|
|
|
|
|
|
|
|
|
|
|
|
|
|
|
|
|
|
|
|
|
||||||
|
|
|
|
|
|
|
|
|
|
|
2.1 |
|
|
|
|
|
|
|
|
|
|
|
|
|
|
|
|
|
|
|
|
|
b |
cycle 1 |
|
|||||||||||||||
|
|
|
|
|
|
|
|
|
|
|
|
|
|
|
|
|
|
|
|
|
|
|
|
|
|
|
|
|
|
|
|
|
|
|
|
|
|
|
|
|
|
|||||||||
|
|
|
|
|
|
|
|
|
|
|
|
|
|
|
|
|
|
|
|
|
|
|
|
|
|
|
|
|
|
|
|
|
|
|
|
|
|
|
|
|
|
|
|
|||||||
|
|
|
|
|
|
|
|
|
|
|
|
|
|
|
|
|
|
|
|
|
|
|
|
|
|
|
|
|
|
|
|
|
|
|
|
|
|
|
|
|
|
|
|
|
|
|||||
|
|
|
|
|
|
|
|
|
|
|
|
|
|
|
|
|
|
|
|
|
|
|
|
|
|
|
|
|
|
|
|
|
|
|
|
|
|
|
|
|
|
|
|
|
|
|
|
|
|
|
|
|
|
|
|
|
|
|
|
|
|
|
|
|
|
|
|
|
|
|
|
|
|
|
|
|
|
|
|
|
|
|
|
|
|
|
|
|
|
|
|
|
|
|
|
|
|
|
|
||
|
|
|
|
|
|
|
|
|
|
|
|
|
|
|
O |
|
|
|
|
R |
H |
|
O |
|
|
|
|
H |
|
|
|
S |
O |
|
|
|
|
|
||||||||||||
|
|
|
|
|
|
|
|
|
|
|
H2N |
|
|
|
|
|
|
|
|
|
|
|
|
|
|
|
|
|
|
|
|
N |
|
|
|
|
|
|
|
|
||||||||||
|
|
|
|
|
|
|
|
|
|
|
|
|
|
|
N |
|
|
|
|
|
|
N |
|
OH |
|
+ Ph |
N |
|
|
|
|
|
|
|
|
|
||||||||||||||
|
|
|
|
|
|
|
|
|
|
|
|
|
|
|
|
|
|
|
|
|
|
|
|
|
|
|
|
|
|
|
|
|
|
|
|
|
|
|
||||||||||||
|
|
|
|
|
|
|
|
|
|
|
|
Rn-1 |
H |
|
O |
|
|
R1 |
|
|
|
|
|
|
|
|
R |
|
|
|
|
|
||||||||||||||||||
|
|
|
|
|
|
|
|
|
|
|
|
|
|
|
|
|
|
|
|
|
|
|
|
|
|
|
|
|||||||||||||||||||||||
|
|
|
|
|
|
|
|
|
|
|
|
|
|
|
|
|
|
|
|
|
|
|
|
|
|
|
|
|
|
|
|
|
|
|||||||||||||||||
|
|
|
|
|
|
|
|
|
|
|
|
|
|
|
|
|
|
|
|
|
|
|
|
|
|
|
|
|
n-3 |
|
|
|
|
|
|
|
|
|
|
|
n |
|
|
|
|
|
||||
|
|
|
|
|
|
|
|
|
|
|
|
|
|
|
|
|
|
|
|
|
|
|
|
|
|
|
|
|
|
|
|
|
|
|
|
|
|
|
|
|
|
|
|
|
|
|||||
|
|
|
|
|
|
|
|
|
|
|
|
cycle 2 |
|
|
|
|
a, b |
2.3 |
|
|
|
|
|
|
|
|
c |
|
|
|
|
|
|
|||||||||||||||||
|
|
|
|
|
|
|
|
|
|
|
|
|
|
|
|
|
|
|
|
|
|
|
|
|
|
|
|
|
|
|||||||||||||||||||||
|
|
|
|
|
|
|
|
|
|
|
|
|
|
|
|
|
|
|
|
|
|
|
|
|
|
|
S |
|
|
|
|
|
|
|||||||||||||||||
|
|
|
|
|
|
|
|
|
|
|
|
|
|
|
|
|
|
|
|
|
|
|
|
|
|
|
|
|
|
|
|
|
||||||||||||||||||
|
|
|
|
|
|
|
|
|
|
|
|
|
|
|
|
|
|
|
|
|
|
|
|
|
|
|
|
|
|
|
|
|
|
|
|
|
|
|
|
|
|
|
|
|
|
|
|
|||
|
|
|
|
|
|
|
|
|
|
|
|
|
|
|
|
|
|
|
|
|
H |
|
|
|
S |
|
|
|
|
|
|
|
Ph |
N |
|
|
NH |
|
|
|
|
|
||||||||
|
|
|
|
|
|
|
|
|
|
|
|
|
|
|
|
|
|
|
|
|
|
|
|
|
|
|
|
|
|
|
|
|
|
|
|
|
|
|||||||||||||
|
|
|
|
S |
|
|
|
|
|
|
|
|
|
|
|
|
|
|
|
|
|
|
|
|
|
|
|
|
|
|
|
|
|
|
|
|
|
|
|
|
|
|||||||||
|
|
|
|
|
|
|
|
c |
|
N |
|
|
|
|
O |
|
|
|
|
|
|
|
|
|
|
|
|
|
|
|
|
|
||||||||||||||||||
Ph |
|
|
|
|
|
|
|
|
|
|
|
|
|
|
Ph |
|
|
|
|
|
|
|
|
|
|
|
|
|
|
|
|
|
|
|
O |
|
|
|
Rn |
|
|
|
|
|
||||||
|
|
|
|
|
|
|
|
|
|
|
|
|
|
|
|
|
|
|
|
|
|
|
|
|
|
|
|
|
|
|
|
|
|
|
|
|
|
|
|
|
||||||||||
|
|
|
|
|
|
|
|
|
|
|
|
|
|
|
|
|
|
|
|
|
|
|
|
|
|
|
|
|
|
|
|
|
|
|
|
|
|
|
|
|
|
|
|
|
|
|||||
|
N |
|
|
|
NH |
|
|
|
|
|
|
|
|
|
|
|
|
|
N |
|
|
|
|
|
|
|
|
|
|
|
2.2 |
|
|
|
|
|
|
|||||||||||||
|
|
|
|
|
|
|
|
|
|
|
|
|
|
|
|
|
|
|
|
|
|
|
|
Rn-1 |
|
|
|
|
|
|
|
|
|
|
|
|
|
|
||||||||||||
|
|
|
|
|
|
|
|
|
|
|
|
|
|
|
|
|
|
|
|
|
|
|
+ |
|
|
|
|
|
|
|
|
|
|
|
|
|
|
|
|
|
|
|
|
|
|
|
||||
|
O |
|
|
|
Rn-1 |
|
|
|
|
|
|
|
|
|
|
|
|
|
|
|
|
|
|
|
|
|
|
|
|
|
|
|
|
|
|
|
|
|
|
|
||||||||||
|
|
|
|
|
|
|
|
|
|
|
|
|
|
|
|
|
|
|
|
|
|
|
|
|
|
|
|
|
|
|
|
|
|
|
|
|
|
|
|
|
|
|||||||||
|
|
|
|
|
|
|
|
|
|
|
|
|
|
|
|
|
|
|
|
|
|
|
|
|
|
|
|
|
|
|
|
|
|
|
|
|
|
|
S |
|
||||||||||
|
|
|
|
|
|
|
|
|
|
|
|
|
|
|
O |
|
|
|
|
R |
|
|
|
O |
|
cycle n |
|
|
|
|
|
|
Ph N |
|
|
|
|
|
||||||||||||
|
|
|
|
|
|
|
|
|
|
|
|
|
|
|
|
|
|
|
|
|
|
|
|
|
|
|
|
|
H |
|
|
|
|
|
|
|
|
|
|
|
|
|
|
|
|
|
NH |
|||
|
|
|
|
|
|
|
|
|
|
|
H2N |
|
N |
|
|
|
|
|
|
N |
|
OH |
|
|
|
|
|
|
|
|
|
|
|
|
|
|
||||||||||||||
|
|
|
|
|
|
|
|
|
|
|
|
|
|
|
|
|
|
|
|
|
|
|
|
|
|
|
|
|
|
|
|
|
|
|
|
|||||||||||||||
|
|
|
|
|
|
|
|
|
|
|
|
Rn-2 |
|
H |
|
O |
|
|
|
R1 |
|
|
|
|
|
|
|
|
|
|
|
O |
|
|
|
R |
1 |
|||||||||||||
|
|
|
|
|
|
|
|
|
|
|
|
|
|
|
|
|
|
|
|
|
|
|
|
|
|
|
|
|
|
|
||||||||||||||||||||
|
|
|
|
|
|
|
|
|
|
|
|
|
|
|
|
|
|
|
|
|
|
|
|
|
|
|
|
|
|
|
|
|
||||||||||||||||||
|
|
|
|
|
|
|
|
|
|
|
|
|
|
|
|
|
|
|
|
|
|
|
|
|
|
|
|
n-4 |
|
|
|
|
|
|
|
|
|
|
|
|
|
|
|
|
|
|||||
|
|
|
|
|
|
|
|
|
|
|
|
|
|
|
|
|
|
|
|
|
|
|
|
|
|
|
|
|
|
|
|
|
|
|
|
|
|
|
|
|
|
|
|
|
a)PhNCS, base, pH 9-10, 45°-50°C;
b)anhydrous TFA, 45°-55°C;
c)25% aq. TFA, 60°-65°C.
Figure 2.2 Edman sequencing of peptides: iterative cycles.
Commercially available Edman sequencers perform a fully automated sequencing and HPLC separation and provide the HPLC traces for each reaction cycle. The presence of deletion peptides is easily spotted qualitatively by the appearance of secondary PTH peaks in each reaction cycle. The method is limited to α-amino acids, but polypeptides containing exotic α-amino acids can also be sequenced provided the appropriate standard PTH is available. The method is very sensitive, and usually a single bead is enough to allow an accurate determination of the sequence of a peptide, but the quantitation of each amino acid is difficult because of their different stabilities and properties under the conditions of the cleavage cycle. Edman sequencing can be used in conjunction with other techniques such as MS.
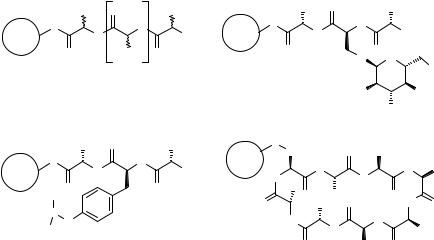
50 SOLID-PHASE SYNTHESIS: OLIGOMERIC MOLECULES
2.1.2 Solid-Phase Synthesis of Peptide-Related Oligomers
The wide use of peptides for biological applications has led to the SPS of many relevant sequences. The well-known drawbacks of natural peptides, such as their poor penetration through biological membranes and their poor stability in biological fluids, has stimulated the synthesis of modified peptides to overcome these limitations. There have been corresponding advances in SPS to accommodate altered structures, and some generic examples of peptide analogs prepared on SP are shown in Fig. 2.3.
The use of D-amino acids, or of exotic L- or D-amino acids such as 2.4, does not significantly change the SPS procedure; however, the availability of suitable N-Fmoc- protected exotic α-amino acids may be limited; thus a method called solid-phase unnatural peptide synthesis (SP-UPS) has been reported. This method, which entails the SPS of unnatural α-amino acids by alkylation of Fmoc-Gly under mild conditions, has been integrated with traditional SP peptide chemistry (28).
The synthesis of glycopeptides, such as 2.5, is compatible with the Fmoc strategy, and glycosylated Fmoc-protected amino acids such as Thr, Ser, Cys, and Tyr have been inserted into peptides prepared on SP (29). The sugar hydroxyls are normally protected with Fmoc-compatible acyl, benzyl, or silyl groups. Similarly, phosphopeptides 2.6 are prepared using Fmoc-protected phosphorylated Thr, Ser, and Tyr building blocks or by the so-called global phosphorylation method, which saturates the hydroxyl side chains of Thr, Ser, and Tyr in the resin-bound peptide (30). The phosphate residues may be protected as Fmoc-compatible t-butyl or benzyl esters.
R O |
R |
L |
H |
N |
|
N |
NH2 |
H |
R O |
O |
|
|
n |
R = natural AAs, L- or D-configuration, unnatural AAs, L- or D-configuration
2.4
R O |
R |
L |
H |
N |
|
N |
NH2 |
H |
O |
O |
|
OH |
|
O P
O
HO
phosphorylated Thr, Ser and Tyr
2.6
L = LINKER
R |
O |
H |
R |
|
L |
|
|
|
|
N |
N |
NH2 |
|
|
|
|
|
||
O |
H |
|
O |
|
|
O |
|
||
glycosylated |
|
O |
OH |
|
|
|
|
||
Thr, Ser, Cys and Tyr |
|
|
|
|
2.5 |
|
HO |
OH |
|
|
|
OH |
|
|
|
|
|
|
|
L |
|
O |
R |
|
R |
H |
|
||
|
|
H |
R |
|
N |
N |
N |
N |
|
|
|
|
||
R |
R |
H |
O |
|
O |
O |
O |
||
O |
R |
NH |
||
NH |
|
|
H |
|
N |
|
N |
|
|
|
|
R |
|
|
O |
H |
R |
O |
|
|
|
R = D- or L-natural and unnatural AAs
2.7
Figure 2.3 Structures of peptides containing non-natural amino acids (2.4), glycopeptides (2.5), phosphopeptides (2.6), and cyclic peptides (2.7).
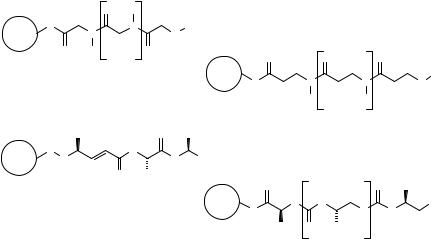
2.1 PEPTIDES 51
Many biologically active peptides are cyclic in nature, and the SPS of this class of peptides, exemplified by 2.7, has also received attention with several different strategies for the final cyclization. The phenomenon of pseudodilution on bead in which each resin site is essentially isolated from its neighbors favors the intramolecular cyclization reaction compared to the intermolecular dimerization, which occurs in solution even at high dilutions. The SPS of cyclic peptides has recently been covered in two excellent reviews (31, 32). The technique of cyclative cleavage via the N- or the C-terminus (see Section 1.2.7) has been used, as has anchoring through amino acid side chains with sequential cyclization and peptide release.
Solid-phase synthesis has also been used to make peptoids, some examples of which are shown in Fig. 2.4. Compounds of general structure 2.8, where the amino acid side chain is on the nitrogen, have been prepared either by the corresponding Fmocprotected N-alkylated glycines (33) or, in an improved method, via treatment of a resin-bound secondary amine with bromoacetic acid to produce the first peptoid building block, which is then elaborated via iteration of the procedure (34). Other modified β-peptoid structures such as 2.9 with repeating β-amino propionic units have been prepared by acylation of a resin-bound amine with acriloyl chloride followed by Michael addition of a primary amine. The cycle is repeated to build up the polymer (35).
Peptidomimetics, a class of compound in which the peptidic bond is replaced by a grouping of similar geometry, have also been prepared on SP. Two examples are shown in Fig. 2.4. The peptidomimetic 2.10, in which the N-terminal amide has been substituted by an olefin bond, was prepared by the Wittig reaction of a resin-bound α-amino aldehyde and sequential peptide SPS to give oligomers of various lengths (36). The amidic bond of peptides has also been replaced by a urea, as in the
O |
R |
|
|
|
|
L |
N |
R |
|
|
|
N |
N |
|
|
|
|
O R |
H |
|
|
|
|
O |
O |
|
O |
O |
|
|
n |
|
|||
|
|
L |
N |
|
R |
R: natural AAs' side chains |
N |
N |
|||
|
|
|
R |
R |
H |
2.8 |
|
|
|
||
|
|
|
|
n |
|
|
|
|
|
|
|
R |
O |
R: natural AAs' side chains |
|||
R |
|
2.9 |
|
||
L |
H |
|
|
|
|
N |
COOH |
|
|
|
|
N |
N |
|
|
|
|
H |
H |
|
|
|
|
O |
R |
|
|
|
|
2.10 |
|
O |
H |
H |
O R |
|
|
NH2 |
|||
|
|
L |
N |
N |
|
|
|
|
N |
N |
|
|
|
R |
O |
H |
H |
|
|
R |
|
||
L = LINKER |
|
|
|
|
n |
2.11
Figure 2.4 Structures of peptoids (2.8, 2.9), peptidomimetics (2.10), and oligoureas (2.11).
52 SOLID-PHASE SYNTHESIS: OLIGOMERIC MOLECULES
β-oligoureas 2.11 prepared from a resin-bound amino acid treated with a suitable N-phthaloyl amino isocyanate. Deprotection releases the terminal amine, which can be treated with another N-phthaloyl-protected amino isocyanate to repeat the cycle (37).
The importance of SPS for the rapid and reliable preparation of complex peptides, peptoids, and peptidomimetics cannot be underestimated. The exploitation of the SPS of this type of oligomer has allowed the birth of synthetic combinatorial chemistry and its subsequent exploitation in pharmaceutical research. Even now that the main accent of SPS and synthetic combinatorial chemistry has shifted toward the preparation of small nonoligomeric organic molecules, a steady flow of reports regarding the SPS of medium-long peptides or peptidelike molecules with significant biological properties is still appearing.
A brief survey of recently appeared papers in peptide SPS should highlight the trends related to this area. They include the synthesis of novel amino acidic building blocks such as sulfonylated aspartic and glutamic acids (38), ω-N-substituted (39) and thio (40) arginines, or phosphotyrosines (41); the introduction of novel orthogonal protecting groups such as the cyclohexyl ether for serine (42) or the 2-(4- nitrophenyl)sulfonylethoxycarbonyl (Nsc) carbamate for amines (43); the continuous improvements in experimental protocols, regarding the automated synthesis of small proteins (44), or the reduction of racemization (45, 46), or the introduction of the swelling volume coupling protocol (47); the introduction of novel analytical techniques to monitor and quantify peptide SPS (48); the introduction of novel resins (49) or linkers (50–52) for peptide SPs; the postcoupling modification of amino acid side chains on SP, as for the transformation of aspartyl or glutamyl carboxylates (53); the synthesis of biologically relevant peptide sequences (54, 55); the synthesis of novel cyclic peptides (56–58); and the synthesis of novel peptide analogues belonging to the classes of glycopeptides (59), of peptide and glycopeptide dendrimers (60, 61), of oligopyrrole–peptide conjugates (62), of (PNA)–peptide conjugates (63) of ureabased (64), trifluoromethyl ketone–containing (65) and constrained peptidomimetics (66). Clearly, everything related to peptide SPS is reliable and flexible, but improvements are always looked for in any specific section and will continue to be the topic of high-quality scientific research.
2.1.3 An Example: SPS of cyclo(Arg-D-Phe-Pro-Glu-Asp-Asn- Tyr-Glu-Ala-Ala)
The SPS of the cyclic homodetic peptide cyclo(Arg-D-Phe-Pro-Glu-Asp-Asn-Tyr- Glu-Ala-Ala) 2.12 was previously attempted by anchoring the starting amino acid to the resin through the side chain (via L1–4, Fig. 2.5) (67). The carboxylic groups of Glu6, Glu10, and Asp7 and the amide of Asn8 were anchored during several different attempts using the 4-hydroxymethylphenoxyacetic linker for the acids and the PAL linker for the amide bond. The best results were obtained using the Asn8 handle and the PAL linker, with a 71% yield of the cyclized decapeptide (HPLC, purity calculated with an authentic standard sample).