
Scheer Solar Economy Renewable Energy for a Sustainable Global Future (Earthscan, 2005)
.pdf192 THROWING OFF THE FOSSIL SUPPLY CHAINS
Prevs post-conversion storage
Table 6.2 summarizes the energy storage media just discussed, and offers a crude evaluation of each with respect to the state of technological development, efficiency, energy density (a proxy measure of the space required), maximum number of charge/discharge cycles and the resultant overall performance and environmental impact. The ability to store electricity makes independence of the grid and its controlling influence on electricity supplies a real possibility. It also permits rapid expansion in the amount of conventional energy replaced by PV, wind and solar heating.
No energy supply system can do without energy storage. In the case of fossil fuels, the energy stores are stockpiles of coal and oil and gas tanks. Biomass being just as easy to store as fossil primary energy, storage would not be a particular issue for renewable energy if in future it were all supplied from biomass. However, as Chapter 2 concluded, though this would be possible in theory, it would be completely unnecessary in practice, and there are numerous reasons why it cannot be recommended: the role of biomass as an industrial raw material is crucial to achieving a sustainable economy.
Switching to biomass fuel would prevent further accumulation of greenhouse gases in the atmosphere. But fossil fuel pyromania has so overloaded the atmosphere with CO2 that more is needed than simply stabilizing atmospheric CO2 at current levels: it must be actively reduced. Plants absorb CO2 as they grow, sequestering the carbon and supplying the environment with oxygen. Only with their aid will it be possible to start taking CO2 back out of the atmosphere. This means that existing plant cover must be increased, primarily through afforestation, such that plants sequester more carbon and release more oxygen than additional CO2 is emitted into the atmosphere. Burning and regrowing equal amounts of biomass has a net zero effect on the atmosphere. Yet while – unlike fossil fuel consumption – this does not makes things worse, neither does it make them any better.
For any given practical application of renewable energy, it makes sense to choose the form of energy that is most conve-
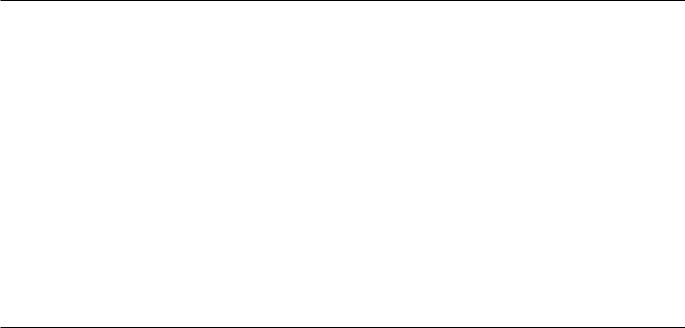
Table 6.2 Energy storage technologies
Storage system |
Level of |
Efficiency |
Cost |
Energy density Charge/discharge Environmental |
Note |
||
|
development |
|
|
(footprint) |
cycles |
impact |
|
|
|
|
|
|
|
|
|
Electrochemical |
|
|
|
|
|
|
most widespread |
Lead-acid batteries |
|
|
|
|
|
|
|
flooded |
3 |
3 |
3 |
1 |
1000 |
1 |
|
gas-tight |
3 |
3 |
2.5 |
1 |
1500 |
1 |
|
NiCd |
3 |
2.5 |
3 |
2 |
2000 |
1 |
|
NiMH |
2 |
2 |
2 |
2 |
1500 |
3 |
in common use |
Li ion |
2 |
3 |
1 |
3 |
5000 |
3 |
in the pipeline/promising |
Electrostatic |
|
|
|
|
|
|
|
Supercapacitors |
1 |
4 |
2 |
3 |
unlimited |
3 |
promising |
Electromechanical |
|
|
|
|
|
|
|
Fly-wheels |
2 |
3 |
3 |
2.5 |
>10,000 |
3 |
promising |
Compressed air |
3 |
3 |
4 |
2 |
unlimited |
3 |
very promising |
Electrodynamic |
|
|
|
|
|
|
|
Superconducting magnets |
1 |
3 |
? |
1 |
unlimited |
3 |
application questionable |
Chemical |
|
|
|
|
|
|
|
Hydrogen |
2 |
2 |
2 |
4 |
limited by fuel cell lifetime |
3 |
multiple applications |
Thermal/thermochemical |
|
|
|
|
|
|
|
Hot-water storage tanks |
3 |
3 |
2 |
2 |
unlimited |
3 |
most widespread |
Magnesium hybrid |
3 |
3 |
2 |
3 |
unlimited |
3 |
promising |
RIDG THE EYONDB NERGYE
Scale: 1 = poor; 2 = fairly good; 3 = good; 4 = exceptionally good. Source: Hermann Scheer, Heinz Ossenbrink
193
194 THROWING OFF THE FOSSIL SUPPLY CHAINS
nient to store, as far as storage is necessary. To this end, it is useful to know whether the energy is stored before or after the conversion process. The former is the typical pattern for energy derived from fossil fuel combustion. Of the renewable energy sources, only biomass can be stored in its original state. Postconversion storage is the typical pattern for non-combustive renewable energy generation: direct conversion of sunlight into electricity, wind power, non-reservoir water power or direct solar heating.
Biomass is thus best suited to those applications which require pre-conversion storage, primarily machinery that works on the combustion principle, motor vehicles in particular. Another candidate would be solar hydrogen. The advantages of biomass over solar hydrogen, however, cannot be overlooked. Hydrogen fuel can only be supplied in quantities large enough for the mass transportation market if produced on an industrial scale. The focus of attention in this respect has been on the fuel cell.13 Fuel cells are also the subject of keen interest from the car manufacturers, who are finally beginning to prepare for a post-oil world. The difference between the solarelectric car as discussed earlier and a car powered by fuel cells is that in the latter case, the electricity is generated on board, rather than taken from a battery.
Fuel cells are power plants in which a form of cold combustion process converts hydrogen or another gaseous fuel into electricity – effectively reverse electrolysis. In a car, this process takes place in the vehicle itself, and the electricity produced is used to power an electric motor. Fuel cell cars should enter massmarket production in the first decade of the 21st century. They will be almost completely silent, and emit nothing more harmful than water vapour. Until hydrogen starts being produced in sufficient quantities, the idea is to run them on natural gas.
Will the need for large quantities of fuel mean that fuel cell cars remain dependent on international energy supply chains – not necessarily on the grid, but on global suppliers of solar hydrogen? Will the motors whose thirst for fuel sparked the globalization of the energy industry in the 20th century be the factor that ensures the continued need for a global energy industry, in the form of the fuel cell and its need for hydrogen produced using electricity from large-scale power plants?
ENERGY BEYOND THE GRID 195
The range of renewable energy options is wider than this, even in the case of road fuel, and irrespective of the scope for fitting cars with more effective and lighter systems for direct electricity storage. Hydrogen can also be produced from biomass on a regional basis,14 and fuel cells do not necessarily have to run on hydrogen. Other options are:
•Biomass-derived alcohol (ethanol). This does not have to come from distant tropical sugar cane plantations; alcohol can also be produced from sorghum, or from the lignin component in wood. It has been calculated that 1 tonne of dry wood mass can yield 387 litres of ethanol.15
•Gasified biomass. Gasification plants can cope with all forms of biomass, so there would even be no need to separate or otherwise prepare the material beforehand, making it possible to utilize the entire plant.16
•Methanol, which can be synthesized from plant-derived carbon and hydrogen.17
•Biological petrol subsitutes, which can be synthesized from gasified biomass and hydrogen, which in turn can be produced using local wind power plants.18
•Biogas, which results from anaerobic fermentation of organic waste.
Clearly, there is no particular need to put all our eggs in the fuel cell basket; internal combustion engines can be made to run on vegetable oil instead of diesel, gasified biomass rather than natural gas, or on bioethanol or biomethanol. An extensive discussion of all these, and other, various options is not possible here, but there is a wealth of literature and calculations available, all of which can already draw on practical examples.19
Synergistic applications, cross-substitution and all-load micro-power plants
The importance of power storage lies in the opportunity for complete, efficient and cost-effective self-sufficiency in electricity, heating and even fuel. Generators for domestic

196 THROWING OFF THE FOSSIL SUPPLY CHAINS
power production have been around for as long as there have been motors. Up till now, electricity production from such generators has only been a viable proposition in comparison to mains electricity if combined with heat production. However, the mutual interdependency of heat and electricity production in a cogeneration plant and the consequent problem of overor underproduction has still made a grid connection necessary, so that surplus electricity could be sold to the grid (usually below cost) and additional electricity purchased from the grid to make up any shortfall. The ability to store electricity solves the problem of overor underproduction for operators of CHP plants, and consequently their dependency on the grid. Any surplus can be stored, be it heat or electricity, because heat can also be converted into electricity. All stored electricity can be used, whether to meet the electricity needs of the building, to run a car or to supply heat.
Decentralized energy storage is the link that enables electricity generation from renewable sources to be combined with motor technology in an optimal manner. The motors and drives industry* is potentially a staunch ally of renewable energy
– it need only refocus on the production of motors that can be run directly from renewable sources or from stored energy. The outcome would not be one single type of motor, but a wide variety of types, depending on the particular energy source and energy generation scheme.
If autonomous energy supplies are to achieve broad appeal and develop a momentum of their own – to become a ‘solar steam-engine’ – then the number of interlocking technologies must be kept small. With a domestic-level cogeneration plant in combination with a power storage system, residents could become independent of external electricity supplies and produce their own motor fuel. In this case, all they would need would be a supply of fuel for the cogeneration plant, the lynchpin in this scheme.
If they have a fuel cell car, the on-board fuel cell could be combined with a power storage system to generate electricity while the car stands idle in the garage, with sufficient reserves
* Manufacturers of electric motors and drives for industry (a drive being essentially an inverter-based regulator for an electric motor).
ENERGY BEYOND THE GRID 197
of power being laid in to cover times when the car is in use. The power generation plant then comes with the car. If a PV and electrolysis plant is added in, all or some of the fuel for the car can be domestically produced.
These are only a few examples among many of what could be achieved in future. Buildings whose heating, electricity and fuel needs are met solely from the sun, independently of the grid, are also conceivable.
Buildings as energy collectors and generators
Buildings do not just consume energy: in future they must also be seen as systems for collecting and harnessing solar energy. Among other things, conservationally minded architects or planners must give thought to:
•optimal solar gain, by allowing as much daylight as possible into the house. Besides windows, this might mean novel light storage systems;
•exploitation of ambient heat as a secondary heat source; siting the building so as to make maximum use of the sunlight and avoid shading; windows to open up the sunny side of the building and insulation on the other sides; optimal locations for solar collectors and PV arrays;
•natural ventilation from prevailing winds on the site;
•selecting building materials according to their insulating properties and the energy cost of their manufacture – ie, determining where concrete and aluminium can be replaced by wood, rammed earth or steel; and
•internal air circulation, which can also be used for seasonal cooling or heating.
In the end it comes down to what the building engineer Klaus Daniels calls ‘building to the climate’: ‘What distinguishes intelligently planned and operated buildings above all is their ability meet their occupants’ needs directly from the environment without hi-tech equipment, through natural lighting, natural ventilation, means of controlling the total amount of energy or daylight entering the building, and so on.’20

198 THROWING OFF THE FOSSIL SUPPLY CHAINS
Table 6.3 Energy regulation strategies in biological systems, compared with existing and potential architectural applications (selected examples)
Biological energy regulation |
Existing and potential architectural |
strategies |
applications |
|
|
Control of heat loss through capillary |
Adjustable exterior surfaces |
dilation/contraction |
|
Contraflow and heat-exchange
(eg whale flippers, seabird legs, gills)
Insulation through zoning and restricted blood circulation in cold conditions
(eg in arctic animals and insect nests)
Pigment alterations to make best use of solar heat
Expansion and contraction of insulating layers (by ruffling up feathers, winter and summer coats)
Light absorption for heat gain (translucent skin or hair, eg alpine plants, polar bear)
Heat exchangers in ventilation shafts and wastewater pipes
Frequently used in traditional architecture (eg farmhouses in Lower Saxony and South Germany, conservatories)
Regulation of light absorption and reflection using blinds or glass roofing in greenhouses
Adjustable insulation, summer and winter dwellings in traditional architecture, winter windows etc
Modern transparent insulating materials, glass façades
Maximizing heat collection through |
Adjustable façades, porches |
expansion of the collecting surfaces |
|
(by opening wings or exposing flanks |
|
to the sun) |
|
Evaporative cooling (sweat, panting, transpiration in plants)
Shading to promote convection (eg cactus ribbing)
Light filtering and distribution (eg South African window plants)
Piped-in light (light channelling in plant shoots)
Cladding which conducts or radiates away excess heat
Suitable shade-producing exterior structures
Glazing with different colouring and material properties, grills, ribbing
Daylighting systems, light pipes
Ventilation (termite mounds, prairie |
Chimneys, wind towers (Arabia, Iran) |
dog warrens) |
|
Centrally regulated heat exchange |
Computer-controlled solar buildings with |
(skin, behaviour) |
an optimal combination of |
|
energy-management measures |
|
|
Source: Helmut Tributsch, Wohnen mit der Sonne (Living with the Sun)
The European Charter for Solar Energy in Architecture and Urban Planning, drawn up under the overall control of the Munich-based architect Thomas Herzog in 1996, lists all these design options in detail.21 Helmut Tributsch of the HahnMeißner Institute in Berlin has attempted to illustrate the
ENERGY BEYOND THE GRID 199
energy-saving potential of intelligent materials using examples of energy regulation in biological systems.22 He calls upon architects to learn from the self-regulating energy systems of the natural world, and to copy them in their choice of material and form. This suggests scope for countless new multifunctional materials (see Table 6.3). The vast range and variety of possibilities that energy-autonomous buildings offer their occupants is clear. It is also clear that we are only just beginning to realize them.
It is not simply a case of bolting on technology, but rather a new form of architecture. In the words of Sir Norman Foster, describing prominent examples: ‘There is an extraordinary elegance that emerges from a true response to climate and place.’23 Building components acquire multiple roles: not just roofs, walls, windows, they also collect, store and exchange energy. The result is a renewed diversity of architecture. As the greatest part of fossil energy consumption takes place in buildings, solar construction techniques become part of the responsibility of society at large to maintain the environment, and part of the responsibility of business at large to reduce the need for imported energy. If buildings increasingly can become autonomous in energy, and the direct need for external supplies of electricity and heat can be reduced towards zero without compromising on lighting and heating, then the built-in solar plant begins to take on the role of power stations, able to sell surplus energy. Energy costs become energy gains.
The technical know-how to revolutionize energy supplies
The technological options described here are the kindling for the energy supply revolution to come.
1The various available sources of energy and autonomous generation and storage technologies make it possible to deploy energy and technology for multiple applications. In particular:
•energy sources can be mutually interchangeable, ie electricity can be replaced with heat or fuel, heat with
200 THROWING OFF THE FOSSIL SUPPLY CHAINS
electricity and fuel, fuel with electricity and heat, thus allowing energy to be used more flexibly and more efficiently;
•individual technologies – heating, cogeneration and storage plant, PV and collector arrays, equipment and vehicles – can be applied to a variety of functions, thus allowing more productive deployment of technology.
2Despite rising electricity consumption, the demand for grid electricity will fall if ever more devices produce and store their own electricity. The same applies to distributed heat, due to the scope for solar-optimized construction techniques.
3Combining electricity storage with motor-driven generators makes it possible to construct local micropower plants capable of serving all loads, rather like a car with an automatic gear-box which shifts gear from neutral up to fifth as required. This renders the load management regimes of the grid-based electricity industry – whereby electricity is supplied from multiple power stations with varying output profiles – superfluous. The costs of maintaining the grid are replaced by the costs of electricity storage and reserve capacity, which will deliver true cost transparency for the first time ever in the energy industry. Once the advantages begin to be realized, the existing electricity industry’s days are numbered. Pylons will cease to ‘march’ across the landscape.
4The familiar pattern of centrally managed supplies of electricity, fuel, heat and process energy will be replaced by a comprehensive decentralized supply.
5The essential prerequisite for all this is the deployment of renewable energy, because only from renewable sources can energy be supplied overwhelmingly without long supply chains. Only then can decentralized technologies lead to a truly decentralized energy system. The quickest and easiest way to meet electricity and heating needs will be through renewables. Fuel can also be supplied from local sources – that is, if the choice of vehicle motor technology does not lead to fuels being replaced by electricity.
ENERGY BEYOND THE GRID 201
It is not enough merely to see the possibilities: we must put them into practice on the ground, be determined to learn how they can shape our lives, and, module by module, we must build on that potential. There will be no shortage of individual and new community strategies for energy supply at local and regional levels. Every new technological and conceptual advance strengthens their economic and cultural appeal, and increases their power to throw off the resource supply chains of the conventional energy system.
The solar technology revolution and the solar information society
At first glance, local or individual energy autarky would seem to require a complex structure, and to be impractical and thus unrealistic to achieve on a broad scale. This impression arises, however, because the public has not yet had enough time to become aware of the slew of new technologies and adjust their habits to suit the new ways of working. On closer examination, energy autarky is no more complex and thus no less convenient than the current structure of energy supply.
Once an autonomous decentralized energy supply is in place, it will demand less of the consumer in terms of costs and personal initiative than do conventional energy supplies today. Obtaining energy is not just a matter of putting the plug in the wall and turning on the switch: the electricity bills must be paid and checked; various gadgets and appliances need spare batteries or time must be allowed for recharging; the boiler needs maintaining, gas or oil must be ordered for the next heating season; the car must be refilled regularly, and there are oil changes, maintenance and emissions check to think about. In future, there will also be the need to compare prices to find the cheapest electricity supplier, as is increasingly happening in the telecoms sector. By comparison, the difficulties with solar energy technologies are the current paucity of information and advice, and the fragmented and uncoordinated way that the technologies have so far been introduced. Coordination could be made considerably easier through the use of IT and associated services, which would