
Multiple Bonds Between Metal Atoms / 03-Chromium Compounds
.pdf
Chromium Compounds 45
Cotton
|
H3C |
OMe |
OMe |
3.4 |
3.5 |
It is an airand moisture-sensitive yellow solid with very low solubility in common solvents, and recrystallization appeared impractical. Because of the very low solubility, a polymeric structure had been proposed by one group,52 although the other workers51 suggested a dinuclear, bridged structure of the correct type. With the idea of getting a compound that might have higher solubility without differing significantly in the stereochemistry close to the chromium atoms, the anion derived from p-methylanisole, 3.5, was used, and the corresponding Cr2(2-MeO-5-MeC6H3)4 compound was prepared53 by the reaction:
Me |
|
|
|
|
|
|
|
|
|
|
|
H3C |
|
H3C |
|
|
|
|
|
|
LiBu |
|
|
Cr2(O2CCH3)4 |
|
|
|
|
|
|
|
OMe |
|
|
|
|
OMe 4 |
||
OMe |
|
Li |
|
|
|
|
|
|
|
|
Cr |
|
|
Cr |
|||||
|
|
|
|
|
|
|
|||
|
|
|
|
|
|
This pyrophoric substance resembles the anisole compound very closely, including low solubility, but fortunately crystals, albeit small ones, were obtained and the structure was determined. It is shown in Fig. 3.7. This compound has an even shorter Cr–Cr distance than Cr2(DMP)4, namely, 1.828(2) Å, and this remains the shortest known metal–metal bond in an isolable compound.
Fig. 3.7. The molecular structure of Cr2(2-MeO-5-MeC6H3)4.
In addition to Cr2(2-MeOC6H4)4, Hein and Tille54 had also reported a compound containing the phenoxide dianion 3.6 with a proposed formula of Li2Cr(C6H4O)2·LiBr·3Et2O. It has been found that this compound can indeed be prepared55 and that the presence of LiBr in the reaction mixture, as well as in the product, greatly enhances the tractability of the substance from the point of view of obtaining good crystals. The crystal structure55 shows that the unit of interest is that in Fig. 3.8. In this centrosymmetric unit the Cr–Cr distance is 1.830(4) Å, which is, statistically, indistinguishable from that in Cr2(2-MeO-5-MeC6H3)4. The Br– ions are well coordinated to the Li+ ions, and the Cr···Br distances are too long (3.226(2) Å) to signify bonding.

46Multiple Bonds Between Metal Atoms Chapter 3
Finally, in this same period of time, and still using oxophenyl-type ligands, one more important compound was studied.56 The fact that the Cr–Cr bonds are enormously shorter (c. 1.83 Å) when four oxophenyl-type ligands are present than when there are four carboxyl groups (c. 1.97 Å) posed the interesting question as to what the Cr–Cr distance would be if the ligand set consisted of two of each type. By using the ligand 3.7, where the t-butyl groups are so large as to inhibit the simultaneous attachment of four such ligands, it proved possible to obtain a product in which only two of the four acetate groups of Cr2(O2CCH3)4 are replaced. The crystal structure of the resulting compound, shown in Fig. 3.9, has the intended ligand arrangement, and the Cr–Cr distance is 1.862(1) Å.
Fig. 3.8. The molecular structure of the Cr2(2-oxophenyl)4 portion of
Li6Cr2(OC6H4)4Br2(Et2O)6.
O |
OC(CH3)3 |
3.6 |
3.7 |
Fig. 3.9. The molecular structure of Cr2(O2CCH3)2(2-Me3COC6H4)2.

Chromium Compounds 47
Cotton
We conclude this section by returning to the subject of the formal shortness ratios (FSRs) for the supershort Cr–Cr bonds. We have now introduced the shortest ones of all, and a comparison with other very short bonds of all kinds can be made. In Table 3.2 are listed, in order of increasing FSR, a number of M–M bonds, as well as other chemical bonds with very small FSRs. It can be seen that among homonuclear bonds, only the N>N and C>C bonds are as short as the M–M quadruple bonds typically are, and even these are not as short as the shortest Cr–Cr bonds.
Table 3.2. Formal shortness ratios for some chemical bonds
Compound |
Bond |
FSR |
Cr2(2-MeO-5-MeC6H3)4 |
Cr Cr |
0.767 |
Li6Cr2(C6H4O)4Br2·6Et2O |
Cr Cr |
0.771 |
Cr2[2,6-(MeO)2C6H3]4 |
Cr Cr |
0.779 |
Cr2(O2CCH3)2(C6H4OBut)2 |
Cr Cr |
0.785 |
HCCH |
CɓC |
0.783a |
N2 |
NɓN |
0.786a |
Mo2(hpp)4b |
Mo Mo |
0.797 |
CrMo(O2CCH3)4 |
Cr Mo |
0.826 |
[Cr2(CH3)8]4– |
Cr Cr |
0.835 |
Re2(hpp)4Cl2c |
Re Re |
0.854 |
P2 |
PɓP |
0.860 |
[Re2Cl8]2– |
Re Re |
0.869 |
a Pauling does not list R1 for N or C; their single bond radii, 0.70 and 0.77, have been used here. b Shortest Mo–Mo. See F. A. Cotton and D. J. Timmons, Polyhedron 1998, 17, 179.
c F. A. Cotton, J. Gu, C. A. Murillo and D. J. Timmons, J. Chem. Soc., Dalton Trans. 1999, 3741.
The first examples of extremely short Cr–Cr quadruple bonds in Cr2(DMP)4, Cr2(TMP)4 and a few other compounds containing related 2-oxophenyl ligands prompted a systematic search for more ligands that would support very short Cr–Cr bonds. This effort has been very fruitful and the following sections will discuss such compounds in detail. When the Cr2(DMP)4 and Cr2(TMP)4 compounds were first reported, the Cr–Cr bonds were described as “super-short” because lengths <1.9 Å were so far below those in any previously known metal-metal bonded compounds. Since this time (c. 1978) Cr–Cr bonds throughout the range 1.83-2.2 Å have been found, and the basis for any particular line of demarcation, such as 1.9 Å or 2.0 has become debatable.
3.2.2 2-Oxopyridinate and related compounds
In the search that ensued following the discovery of the compounds just discussed in Section 3.2.1 for more Cr24+ compounds with very short Cr–Cr bonds, the next important class of ligands were the anions of substituted 2-hydroxypyridines, chosen because of their structural similarity to the 3.4 - 3.7 ligands. The first of these was the 6-methyl compound, 3.8, abbreviated mhp. The preparation of Cr2(mhp)4 was carried out by adding NaOMe to a solution of Cr2(O2CCH3)4, followed by Hmhp. Upon recrystallization from CH2Cl2, the yellow crystalline product Cr2(mhp)4·CH2Cl2 was obtained.57 The molecular structure is shown in Fig 3.10. The hydrated acetate can also be used as a starting material, in which case Cr2(mhp)4·H2O is obtained, from which the water can be expelled by heating to 100 °C in vacuum. Cr2(mhp)4 or its solvates are remarkably stable; they are unaffected by water vapor, and atmospheric oxygen attacks them so slowly that only after several weeks in air is surface discoloration (to green) noticeable. Cr2(mhp)4 is one of the most air-stable chromium(II) compounds known.

48Multiple Bonds Between Metal Atoms Chapter 3
H3C N O
3.8
Fig. 3.10. The molecular structure of Cr2(mhp)4.
It was found that Mo2(mhp)4 can be prepared in a similar way. Since there was no W2(O2CCH3)4 available as a starting material, direct reaction of W(CO)6 with Hmhp in refluxing diglyme was tried and found to give an excellent yield of W2(mhp)4. For the molybdenum compound a similar reaction with Mo(CO)6 is also a practical preparative method of comparable efficiency to the reaction of the mhp anion with the acetate, but the reaction of Cr(CO)6 with Hmhp in diglyme takes place so slowly as to make this a distinctly inferior preparative reaction. The isolation of the three M2(mhp)4 (M = Cr, Mo, W) compounds marked the first time homologous multiply-bonded dimetal compounds from the same group in the periodic table had been reported.
The structure of Cr2(mhp)4 (Fig. 3.10) displays a very short Cr–Cr distance (1.889(1) Å). The molybdenum and tungsten compounds are isostructural and form isotypic crystals. It will be noted, upon comparing Figs. 3.6 and 3.10, that there is a qualitative difference between the ligand arrangements (regioisomerism) in the Cr2(DMP)4 and Cr2(mhp)4 molecules. In the former the ligands are arranged with unlike ligand atoms trans (3.9), while in the latter like atoms are trans (3.10). Thus, in the former case a center of symmetry is possible and the idealized symmetry is C2h. In the latter case there can be no inversion center and the idealized symmetry is D2d. Both of these structure types are found in many other M2L4 compounds, and there is no general way of predicting which one will be preferred. They are evidently of very similar stability as far as the metal–metal and metal–ligand bonding are concerned, and the choice in any given case may depend on the interplay of many small non-bonded attractions and repulsions, and perhaps also on crystal packing.

|
|
|
|
|
|
|
|
|
|
|
Chromium Compounds |
49 |
|
|
|
|
|
|
|
|
|
|
|
|
|
|
|
|
|
|
|
|
|
|
|
|
Cotton |
|
O |
|
C |
|
O |
|
N |
|
|
||||
|
|
O |
|
C |
|
|
N |
|
O |
|||
|
|
|
|
|
|
|
|
|
|
|
|
|
M |
|
M |
|
M |
|
M |
|
|
||||
|
|
|
|
|
||||||||
|
|
|
|
|
|
|
|
|
|
|
|
|
C |
|
O |
|
|
N |
|
O |
|
|
|
||
C |
|
O |
|
O |
|
N |
|
|
||||
|
|
3.9 |
|
|
|
|
3.10 |
|
|
Two other closely similar Cr2L4 compounds were soon made by the following reactions.58,59
|
LiBu |
_ |
Cr2(O2CCH3)4 |
Cr2(map)4 |
|||
|
|
|
|
||||
H3C N NH2 |
|
||||||
H3C N NH |
|
|
|||||
CH3 |
|
|
|
|
|
||
N |
+ Cr(CO)6 |
diglyme |
Cr2(dmhp)4 |
|
|||
|
|
|
reflux |
|
|
||
H3C N OH |
|
|
|
|
|
These two molecules closely resemble the Cr2(mhp)4 molecule in having a D2d arrangement of ligands and in having very short bonds, with Cr–Cr distances of 1.870(3) Å in Cr2(map)4 and 1.907(3) Å in Cr2(dmhp)4. It became very clear that a vast array of bridging ligands that are stereo-electronic analogs of carboxylate anions can also serve to support M–M multiple bonds.
A later example of a molecule of this type provided a surprise. With the 6-chloro- 2-oxopyridine anion (chp) the Cr2(chp)4 molecule was prepared from Cr2(O2CCH3)4 and found to have the D2d structure, like all the closely related ones.60 However, the Cr–Cr distance here is longer than in the other cases, viz. 1.955(2) Å. The reason for this has still not been determined, but two hypotheses were considered. One is that lone pairs on the chlorine atoms may interact weakly with the metal atom orbitals, perhaps placing some electron density into the /*-orbital, thus weakening the Cr–Cr bond. The other is that the electron withdrawing effect of the Cl atoms weakens the donor strength of the ring nitrogen atoms and that this, in a manner not completely clear, weakens the Cr–Cr bond.
As a seemingly straightforward way of choosing between these two hypotheses, the preparation of the analogous compound with fluorine atoms in place of the chlorine atoms, Cr2(fhp)4, was undertaken.61 It was reasoned that the fluorine atoms of the fhp- ligands would have less of a donor interaction with the /*-orbitals of the chromium atoms but more of an electron withdrawing effect. Thus, in Cr2(fhp)4 the Cr–Cr distance should be longer than in Cr2(chp)4 if the inductive effect dominates but shorter if the donation to metal /-orbitals is the principal factor.
It turned out that this well-designed experiment was foiled by another example of regioisomerism in paddlewheel complexes (see Section 1.4). The fhp complex was indeed obtained but it was a regioisomer of the Cr2(chp)4 compound, as shown in Fig. 3.11. The four fhp ligands all point in the same direction, thus leaving one axial position unencumbered and coordinated by a THF molecule. Apparently this can happen because, unlike CH3 or Cl, the F atom is small enough that four of them will fit on one end and the formation of the additional axial bond to THF stabilizes this structure. The presence of a tightly bound axial THF (Cr–O = 2.266 Å would cause the Cr–Cr bond to be very long, 2.150(2) Å.

50Multiple Bonds Between Metal Atoms Chapter 3
Fig. 3.11. The structure of the Cr2(fhp)4(THF) molecule.
Table 3.3 lists the structures of the earliest compounds just discussed in sections 3.2.1 and 3.2.2, having extremely short Cr–Cr bonds.
Table 3.3. Some compounds with very short Cr–Cr bonds
Ligands |
dCr–Cr (Å) |
ref. |
(DMP)4 |
1.847 (1) |
47,48 |
(TMP)4 |
1.849 (2) |
48,49 |
[(2-ButO)C6H4]2(O2CMe)2 |
1.862 (1) |
56 |
[(2-MeO)(5-Me)C6H3]4 |
1.828 (2) |
53 |
(2-oxophenyl)4 |
1.830 (4) |
55 |
(mhp)4 |
1.889 (1) |
57 |
(map)4 |
1.870 (3) |
58 |
(dmhp)4 |
1.907 (3) |
59 |
(chp)4 |
1.955 (2) |
60 |
(fhp)4(THF) |
2.150 (2) |
61 |
3.2.3 Carboxamidate compounds
From the stability of the Cr2(oxopyridinate)4 compounds, it was inferred that Cr2(carboxamidate)4 compounds, where the carboxamidate ligand is 3.11, would also be stable. The first one reported,62,63 contains the PhNC(CH3)O ligand and has the structure shown in Fig. 3.12. The Cr–Cr bond length is 1.873(4) Å. A number of other carboxamidate compounds have since been made, and all with known structures are listed in Table 3.4. Those with no axial ligands or very weak ones (CH2Cl2, CH2Br2) have short Cr–Cr bonds (< 2.00 Å). Unligated Cr2(carboxamidate)4 molecules are easy to obtain, even if solvated ones are initially isolated, by driving off the coordinated solvent molecules, and Cr2(carboxamidate)4 molecules do not associate as most Cr2(O2CR)4 molecules do. The increase in the Cr–Cr distance by about 0.07 Å upon replacing Ph by 2-xylyl has no obvious explanation.
It is, however, easy to introduce axial ligands to bare Cr2(carboxamidate)4 molecules. This has been exploited to demonstrate the dominant influence of axial ligation, first with a series of axially ligated molecules derived from the unligated molecule64 shown in Fig. 3.13. With very weak axial ligands, the Cr–Cr distance increases only a little (see Table 3.4). With the addition of first one and then two axial THF molecules, the distance increases to 2.023(1) and then 2.221(3) Å. With the addition of two axial pyridine ligands, the increase is huge, to 2.354(5) Å. The structure of this compound is shown in Fig. 3.14. Completely consistent with this trend are the observations on three other Cr2(carboxamidate)4 molecules, as seen in Table 3.4.

Chromium Compounds 51
Cotton
R'
RC
NO
3.11
Fig. 3.12. The structure of Cr2(PhNC(CH3)O)4. |
|
|
||
Table 3.4. Structures of Cr2 carboxamidate compounds |
|
|
||
R |
R' |
Axial ligand(s) |
Cr–Cr, Å |
ref. |
Ph |
Me |
–– |
1.873 (4) |
62,63 |
Me3C |
Me |
–– |
1.866 (2) |
64 |
2,6-Me2Ph |
Me |
–– |
1.937 (2) |
65 |
2,6-Me2Ph |
Me |
CH2Cl2 |
1.949 (2) |
66 |
2,6-Me2Ph |
Me |
CH2Br2 |
1.961 (4) |
67 |
2,6-Me2Ph |
Me |
THF |
2.023 (1) |
66 |
2,6-Me2Ph |
Me |
2THF |
2.221 (3) |
66 |
2,6-Me2Ph |
Me |
2py |
2.354 (5) |
66 |
Ph |
PhNH |
–– |
1.873 (4) |
68 |
Ph |
PhNH |
2THF |
2.246 (2) |
66,68 |
Me2NC6H4 |
CH3 |
THF |
2.006 (2) |
66 |
Fig. 3.13. The structure of Cr2[(2-xylyl)NC(CH3)O]4.
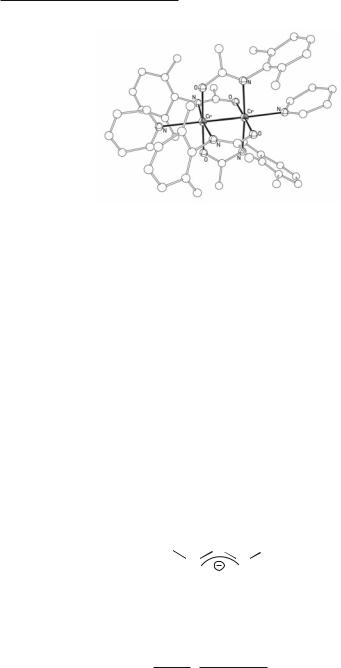
52Multiple Bonds Between Metal Atoms Chapter 3
Fig. 3.14. The structure of Cr2[(2-xylyl)NC(CH3)O]4(py)2.
The behavior of Cr2(carboxamidate)4 compounds in response to axial ligation was reported in 1979 and 1980, and yet for years theoreticians continued to insist69-77 that it was not axial ligation but some property of carboxylate ligands that made Cr–Cr bonds long in axially ligated tetracarboxylates. The message to be drawn from the behavior of the Cr2(carboxamidate)4 compounds, namely, that we must consider very seriously the possibility that the dichotomy of supershort and long (quadruple) Cr–Cr bonds is due primarily to the absence or presence of axial ligands went unnoticed. The lesson to be learned from these events is that clear inference from experimental facts is more likely to be right than the results of calculations of uncertain reliability. There is a well known German adage that makes this point: “Die Theorie leitet; das Experiment entscheidet.”
3.2.4 Amidinate compounds
Amidinate-bridged paddlewheel compounds of M24+ units have emerged as one of the more important classes. A general formula for amidinate ligands is 3.12. While it is in principle possible for R1 and R1' to be different, such unsymmetrical ligands are difficult to make and have played no role, except in cases where R1 is 2-pyridyl and R1' is not.
|
R2 |
|
|
R1 |
|
|
|
C |
R1' |
||
N |
|
|
N |
|
3.12 |
|
The first amidinate compound78 of Cr24+ reported was prepared in a manner that is representative of the most common one from all M2(amidinate)4 compounds:
CH3N(H)C(Ph) NCH3 LiBu
Cr2(O2CCH3)4
Cr2[CH3NC(Ph)NCH3]4
In this paddlewheel structure, shown in Fig. 3.15, the Cr–Cr distance, 1.843(2) Å, is extremely short.
At about the same time as the first amidinate compound was obtained, the first triazinate79 was also obtained by the following reaction:
Li4[Cr2(CH3)8] + 4PhN(H)NNPh Α Cr2(PhN3Ph)4 + 4CH4 + 4LiCH3

Chromium Compounds 53
Cotton
Fig. 3.15. The structure of Cr2[MeNC(Ph)NMe]4.
This is a rare instance of the [Cr2(CH3)8]4- ion being used as a starting material. Cr2(PhN3Ph)4, in which there is a very short Cr–Cr bond, 1.858 (1) Å, is still the only triazinate compound of Cr24+ known. Its structure is shown in Fig. 3.16.
Fig. 3.16. The structure of Cr2(PhN3Ph)4.
Beginning with the first reported amidinate compound in 1979, a great many other Cr2 (amidinate)4 compounds have been made and characterized. While all of those with no special features appear to be quite stable, with very short Cr–Cr distances, a number of bond-weaken- ing features may be introduced. Most of these involve the building in of axial interactions and will be discussed in Section 3.3.1.
The vast majority of the known amidinate compounds of Cr24+ have formamidinate ligands, that is, those in which R2 in 3.12 is hydrogen, and most of these have R1 = R1' = aryl. Those with known structures are listed in Table 3.5. Curiously, what might be called the parent of this series of diaryl formamidinates, the diphenyl compound, has not been reported but no doubt it resembles the p-tolyl compound80 closely. Others listed in Table 3.5 serve to determine the effect of substituents on the Cr–Cr distances. Generally the effects are small and show no meaningful pattern. In the case of the pentafluorophenyl compound,81 it is probably the electronegativity of the C6F5 groups that causes a significant effect, in the expected direction.

54 Multiple Bonds Between Metal Atoms
Chapter 3
Table 3.5. Structures of formamidinate compounds of Cr24+
R in RNC(H)NR |
Cr–Cr, Å |
ref. |
p-tolyl |
1.930(2) |
80 |
o-fluorophenyl |
1.968(2) |
81 |
m-fluorophenyl |
1.916(1) |
81 |
p-fluorophenyl |
1.917(6) |
81 |
pentafluorophenyl |
2.012(1) |
81 |
3,5-difluorophenyl |
1.906(1) |
81 |
o-tolyl |
1.925(1) |
82 |
cyclohexyl |
1.913(3) |
83 |
(o-Clphenyl)3(µ-Cl) |
1.940(1) |
84 |
(o-Brphenyl)3(µ-Cl) |
1.940(2) |
82 |
cis-(o-MeOphenyl)2(O2CCH3)2 |
2.037(1) |
85 |
p-Clphenyl |
1.907(1) |
86 |
3,5-dichlorophenyl† |
1.916(1) |
86 |
m-CF3phenyl† |
1.902(1) |
86 |
m-(CH3O)phenyl† |
1.918(1) |
86 |
o-(CH3O)phenyl |
2.140(2) |
82 |
o-Clphenyl |
2.208(2) |
82 |
o-Brphenyl |
2.272(2) |
82 |
p-C6H5Ph |
1.928(2) |
81 |
[(Me2HC)NC(H)N(Me2HC)]3(µ-BH4) |
1.844(2) |
87 |
trans-[(Xyl)NC(H)N(Xyl)]2(O2CCH3)2(THF)2 |
2.342(1) |
85 |
[(Xyl)NC(H)N(Xyl)]2(µ-Cl)2(THF)2 |
2.612(1) |
85 |
† Also mentioned86 without structures: 3,4-Cl2, p-CF3, p-OCH3
It is evident that ortho substituents can have relatively large effects,82 with the Cr–Cr distances increasing in the order CH3, Cl, CH3O, Br. This will be discussed in Section 3.3.1.
What appears to be a purely steric factor in controlling the formation of Cr2(amidinate)4 compounds was explored83 in the compounds 3.13 and 3.14. In 3.14, the R groups are either methyl or 2-(Me2NCH2)C6H4. While the dinuclear compound 3.13 is a typical paddlewheel with a Cr–Cr bond length of 1.913(3) Å, when the ligands with substituents, even as small as CH3, on the middle carbon atom were employed, only the mononuclear products, 3.14, were isolated. This was attributed to the forcing down of the cyclohexyl groups with consequent reduction of the bite angle of the amidinates, to the extent that they prefer to chelate rather than span even the short Cr–Cr quadruple bond.
|
|
|
|
|
|
|
R |
|
|
|
|
|
|
|
|
|
|
|
|
|
|
|
|
|
|
|
|
|
Cy |
|
C |
Cy |
Me |
Me |
|
|
|
|
|
|
N |
|
N |
||||
|
|
H |
|
|
|
|
|||||
Cy |
Cy |
|
|
Cr |
|
|
|
||||
C |
Cy |
N |
|
N |
Cy |
|
|
||||
N |
|
N |
|
|
|
|
|||||
|
|
4 |
|
C |
|
N |
N |
||||
|
|
|
|
|
|
|
|||||
Cr |
|
|
Cr |
|
|
|
|
|
|
|
|
|
|
|
|
|
|
|
|
|
|
||
|
|
|
|
|
R |
|
Me |
Me |
|||
|
|
|
|
|
|
|
|
||||
|
|
3.13 |
|
|
|
3.14 |
|
3.15 |
Two compounds87 having a mixture of amidinate ligands and others have the formula
Cr2[RNC(H)CNR]3(µ-BH4), with R = (CH3)2CH and c-C6H11. The structure of the former has been reported and it has Cr–Cr =1.844(2) Å. Other mixed ligand complexes were obtained