
Multiple Bonds Between Metal Atoms / 03-Chromium Compounds
.pdf
Chromium Compounds 55
Cotton
deliberately85 by using the formamidinate ligand 3.15. Here the steric hindrance caused by the methyl groups prevents the xylyl rings from occupying all bridging positions, so as to form a paddlewheel compound. Instead, only two can be accomodated in a transoid fashion. Thus, when Cr2(O2CCH3)4 is used as a starting material the transoid molecule shown in Fig. 3.17 is obtained.85 Axial THF molecules are not excluded, and as a result, the Cr–Cr bond becomes quite long, viz., 2.342(1) Å. When CrCl2 is the starting material the molecule shown in Fig 3.18 is obtained, in which the Cr–Cr distance is so long (2.612(1) Å) that little or no Cr–Cr bonding exists.85 By using the formamidine with o-MeOC6H4 groups on the nitrogen atoms a cisoid molecule, Fig. 3.19, is obtained. Note that there are intramolecular axial interactions (a subject discussed generally in Section 3.3.1) which cause a lengthening of the Cr–Cr bond to 2.037(1) Å.
Fig. 3.17. The structure of Cr2(DXylF)2(O2CCH3)2(THF)2.
Fig. 3.18. The structure of Cr2(DXylF)2(µ-Cl)2(THF)2.
Fig. 3.19. The structure of Cr2(DAnioF)2(O2CCH3)2.
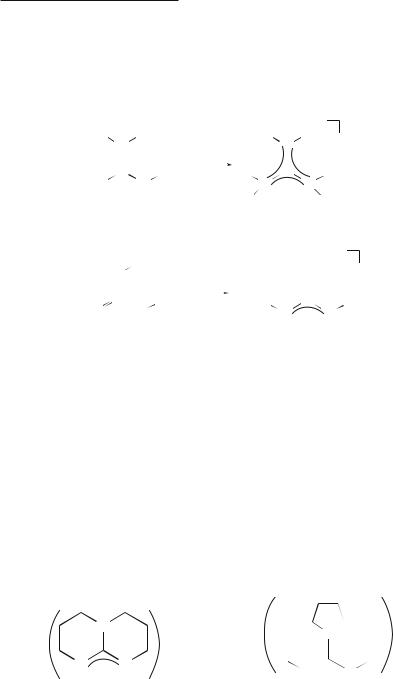
56Multiple Bonds Between Metal Atoms Chapter 3
3.2.5 Guanidinate compounds
While guanidines are best known as very strong organic bases (3.16), they can also be deprotonated (3.17) to give N–C–N bridging ligands. These ligands have the ability to stabilize higher oxidation states of M2n+ cores in general, but some special results are obtained in the case of dichromium species.
|
R |
R |
|
|
|
R |
R |
+ |
|||||
|
|
|
|
|
|||||||||
|
|
N |
|
|
|
|
|
N |
|
|
|
||
|
|
|
|
+ H+ |
|
|
|
|
|
|
|
|
|
|
|
|
|
|
|
|
|
|
|
|
|
||
|
|
C |
|
|
R |
C |
|
|
R |
||||
|
|
R |
|
|
|
|
|||||||
|
RN |
N |
|
|
|
N |
N |
|
|||||
|
|
|
|
R |
|
|
H |
|
|
|
|
R |
|
|
|
|
|
|
|
3.16 |
|
|
|
|
|
||
|
R |
R |
|
|
|
|
|
|
|
- |
|||
|
|
|
|
R |
|
|
R |
||||||
|
N |
|
|
|
|
|
|
|
N |
|
|||
R |
|
|
|
+ B- |
|
|
|
HB + |
|
|
|
|
|
C |
|
|
|
|
|
|
|
||||||
R |
|
|
|
R |
C |
R |
|||||||
|
N |
N |
|
|
|
N |
|
|
N |
||||
|
|
|
|
H |
|
|
|
|
|
|
|
|
3.17
The first guanidinate compound of Cr24+, 3.18, was made with a bicyclic guanidinate anion, abbreviated hpp.88 It is not in itself an especially remarkable compound, although it does have one of the shortest known Cr–Cr bonds, 1.852(1) Å. It led, however, to efforts to prepare other Cr24+ compounds89,90 with guanidinate bridges. One of these, 3.19, provided an unexpected breakthrough. The cyclic voltammogram of this compound displays a reversible oxidation at 0.02 V vs Ag/AgCl. Never before had any dichromium compound been oxidized electrochemically without decomposition. This cation was then isolated by oxidation with AgPF6 and has the structure shown in Fig. 3.20. The only significant structural change, although small, caused by oxidation was an increase of 0.022 Å in the Cr–Cr bond length, from 1.903(4) to 1.925(1) Å. A solid state measurement of the magnetic susceptibility as well as an EPR measurement at X-band frequency (9.5 GHz) confirmed the presence of one unpaired electron, with a g-value of 2.00 ± 0.02. However, the question of where this electron is located was not correctly settled until a later EPR study90 was done at W-band frequency (95 GHz). This showed that the odd electron is located in the Cr25+ core rather than delocalized over the ligand / orbitals.
|
|
|
N |
|
|
|
N |
|
|
||||
|
|
|
|
|
|
|
|
|
|
|
|||
N |
|
N |
Ph |
N |
|
N |
Ph |
4 |
|||||
|
4 |
|
|
||||||||||
|
|
|
|
|
|
|
|
|
|||||
|
|
|
|
|
|
|
|
|
|
|
|
|
|
Cr |
|
|
Cr |
|
Cr |
|
Cr |
|
|
||||
|
|
|
|
|
|
||||||||
|
|
|
|
|
|
||||||||
|
|
|
3.18 |
|
|
|
|
|
3.19 |
|
|
|
|
A measurement of the PES of 3.18 in the gas phase91 gave a β ionization energy of 4.76 eV, which is a surprising low value.

Chromium Compounds 57
Cotton
Fig. 3.20. The structure of the cation of the tetrakis (guanidinate) dichromium compound, 3.19.
3.3Miscellaneous Dichromium Compounds
3.3.1 Compounds with intramolecular axial interactions
Cr–Lax interactions are those whose effect is to place electron density in μ* or /* orbitals. In either case there is weakening and lengthening of the Cr–Cr bonds, as has already been discussed for the cases where the ligands, Lax, are exogenous, that is, independent molecules. This includes the self-association of Cr2(O2CR)4 molecules, discussed in Section 3.1.3. The subject of this section are special cases where the axial ligands are covalently attached to the bridging ligands. It is not always clear whether the axial interaction is with the μ* or the /* orbitals, or perhaps both. A possible but unproven early example that was discovered serendipitously, Cr2(chp)4,60 has already been mentioned (Section 3.2.2).
A designed, unambiguous case of axial / interactions92 occurs in red Cr2(DPhIP)4, 3.20, where the Cr–Cr bond length is 2.265(1) Å. The lengthening effect of the axial ligation is evident by comparison with Cr2(PhIP)4, 3.21, in which the Cr–Cr bond length is 1.858(1) Å. The structure of Cr2(DPhIP)4 is shown in Fig. 3.21, where it can be seen that at each end there are two pendant pyridyl groups that are placed so as to donate their lone pair electron density into the chromium /* orbitals as shown in Fig. 3.22. A similar but less extreme case is presented by comparing the Cr–Cr bond length (1.940[5] Å) in Cr2(dpa)492 with that (1.870(3) Å) in Cr2(map)4.58 The ligands in these two compounds are shown in 3.22 and 3.23, respectively.
N |
|
N |
N |
4 |
N |
N 4 |
|
Cr |
|
Cr |
|
|
Cr |
|
Cr |
|
|
|
|
||||
|
|
|
|
||||
|
|
|
|
||||
|
3.20 |
|
|
3.21 |
|
Fig. 3.21. The structure of the Cr2(DPhIP)4 molecule.

58Multiple Bonds Between Metal Atoms Chapter 3
Fig. 3.22. The manner in which the appended nitrogen atoms of the ligand 3.20 are able to donate lone-pair electron density to a Cr2 /* orbital.
N |
|
N |
N 4 |
N |
NH 4 |
|
Cr |
|
Cr |
|
Cr |
|
Cr |
|
|
|
||||
|
|
|
||||
|
|
|
||||
|
3.22 |
|
3.23 |
|
It is part of the design of 3.20 that the axially interacting lone pairs on the dangling nitrogen atoms are at distances where they can reach only the /* but not the μ* orbitals of the Cr24+ unit. The result for Cr2(DPhIP)4 provides support for the previous proposal (Section 3.1.3) that the Cr–Cr distance of 2.256(4) Å in Cr2(O2CCPh3)4·C6H6 is a result of the donation of C6H6 / electron density into the Cr–Cr /* orbitals.
However, the story is not yet complete on Cr2(DPhIP)4. It occurs in another crystal form where the color is orange, not red, and the Cr–Cr distance is only 2.155(1) Å. Of course, this is still an elongation of about 0.30 Å. To explain this, it is necessary to look more closely at the structures. As indicated by Fig. 3.22, maximum donation to the /* orbitals occurs when the dangling pyridyl ligand and hence, the centroid of the nitrogen lone pair, lies in the same plane as the rest of the ligand. However, rotation about the C–N bond can move the lone pair out of this optimal orientation. This is what happens in the orange crystals (Cr–Cr = 2.155(1) Å) compared to the red ones (Cr–Cr = 2.265(1) Å).
As for Cr2(dpa)4, there is a much smaller lengthing due to axial /* interaction because of an even greater “misdirection” of the nitrogen lone pairs. The increase from Cr2(map)4, 3.23, to Cr2(dpa)4, 3.22, is only 0.07 Å.
Three other examples of the effect of intramolecular donation into Cr2 μ* orbitals have been reported.82 They have already been mentioned in Section 3.2.4 and listed in Table 3.5. These were made using ligands of type 3.24. For X = Me, the Cr–C distance is 1.925(1) Å, and there is no significant donation into any axial orbital. When X is a potential donor, it probably reaches down into the region of the Cr–Cr μ* orbital, although this is not certain. In the series X = MeO, Cl, Br, the Cr–Cr bond lengths greatly increase, to 2.140(2), 2.208(2), and 2.272(2) Å, respectively. Clearly, as the ortho substituents get bigger and “softer” they donate more electron density into the axial orbitals. In these three cases, only one such interaction occurs on each end, rather than two at each end, because only one donor atom at a time can occupy the axial region.

Chromium Compounds 59
Cotton
H
C
N - N
X X
3.24
A molecule with only two o-methoxyphenylformamidinate ligands, 3.25, has also been made.85 Here again, one methoxy oxygen atom at each end can be an axial donor mainly to the μ* orbital. In this case, the Cr–Cr bond length is 2.037(2) Å.
MeO
NN
|
O |
|
|
Me |
Cr |
Cr |
2 |
|
|
|
OO
C 2
3.25
Yet another phenomenon that arises with ligands that have dangling donor atoms is that they can chelate additional metal atoms and hold one or two in axial positions. We mention first the novel case93 of Cr2(DPhIP)4, where, as discussed earlier in this section, the dangling imino nitrogen atoms give rise to axial /* interactions that take the Cr–Cr bond from 1.858(1) Å in Cr2(PhIP)4 to 2.265(1) Å in Cr2(DPhIP)4. This molecule reacts with CuCl to form a product in which a Cu+ is held at each axial position, at distances of 2.628(2) Å and 2.689(2) Å, and the Cr–Cr bond contracts to 1.906(2) Å. It appears that the Cu+ ions have virtually no effect on the Cr–Cr bond.
An interesting example of how the introduction of axial metal atoms can strengthen intramolecular axial interactions, and thus weaken the Cr–Cr bond, is provided by the last two compounds94 in Table 3.6. In Cr2(pyphos)4 there is enough axial interaction by two phosphorus atoms at each end to lengthen the Cr–Cr bond from 1.90 Å (about that in Cr2(mhp)4) to 2.015(5) Å. When the two platinum atoms are anchored in place (at Cr–Pt distances of 2.810 ± 0.01 Å) the Cr–Cr distance goes to 2.389(9) Å. Those who reported these results referred to the platinum atoms acting as “axial donors to the quadruple Cr–Cr bond.” However, this may be a multiple interaction since the platinum atom has all of its d orbitals except dx2-y2 filled.
Table 3.6. Structures with intramolecular axial interactions
Compound |
Cr–Cr, Å |
ref. |
Cr2(PhIP)4 |
1.858 (1) |
92 |
Cr2(DPhIP)4 |
2.265 (1) |
92 |
[Cr2(DPhIP)4Cu2][CuCl2]2 |
1.906 (2) |
93 |
Cr2(dpa)4 |
1.943 (2) |
92 |
Cr2(dpa)4·2CH2Cl2 |
1.940 (1) |
92 |
Cr2(pyphos)4 |
2.015 (5) |
94 |
Cr2(pyphos)4(Me2Pt)2 |
2.389 (9) |
94 |

60Multiple Bonds Between Metal Atoms Chapter 3
3.3.2 Compounds with Cr–C bonds
Some of these have already been mentioned in Section 3.2.1, namely Cr2(DMP)4 and several similar or related ones. Others will now be described.
A phosphine ylid ligand occurs in Cr2[(CH2)P(CH3)2]4.95,96 The structure in which the Cr–Cr bond length is 1.895(3) Å, is shown in Fig. 3.23.
The two compounds, Li4(THF)4[Cr2Me8] and Li4(THF)4[Cr2(C4H8)4], have been reported and their structures described.97-99 Both contain eclipsed Cr–C4 sets of bonds, and the Cr–Cr distances are 1.980(5) and 1.975(5) Å. These structures clearly imply the presence of Cr–Cr quadruple bonds. However, whether they can be regarded as true [Cr2X8]4- compounds is a moot point. A (THF)Li+ ion is located between each opposing pair of RCCr–CrCR components, with distances such that the Li+ ions might be regarded as forming part of five-mem- bered Li C Cr Cr C rings. It may be noted that only lithium compounds of this type have been reported, and there is no indication in the literature that the preparation of a Cr2R84+ compound with any other cation has been attempted.
Fig. 3.23. The structure of Cr2[(CH2)2P(CH3)2]4.
It has more recently been reported100 that the octamethyl compound with diethyl ether in place of THF can be made and that it undergoes the following reaction with excess N,N,N',N'- tetramethylethylenediamine (TMEDA):
|
|
N |
Me |
Me |
N |
[Li(Et2O)]4[Cr2Me8] |
TMEDA |
|
|
||
Li |
Cr |
|
Li |
||
-4Et2O |
|
||||
|
N |
Me |
Me |
N |
|
|
|
||||
|
|
|
|
It is also reported that on addition of excess THF the dinuclear (LiTHF)4Cr2Me8 is formed. The compound [Li(TMEDA)]2[CrMe4] has a square coordinated CrII with four unpaired electrons.
Another molecule having Cr–C bonds is the allyl molecule, Cr2(C3H5)4, which is one of the earliest Cr24+ compounds to be structurally characterized.101,102 The planes of the allyl groups all lie parallel to the Cr–Cr bond. This pyrophoric compound, which has a molybdenum analog, is easily made by reaction of CrCl3 with an excess of allyl Grignard, or by reduction of Cr(C3H5)3 with C2H5Li.103 It is a brownish-black solid with a metallic luster. Both structure determinations are imprecise, but give a Cr–Cr distance of about 1.97-1.98 Å.
An unusual organometallic compound is Cr2(C8H8)3, with one bridging C8H8 and two that are each attached to only one metal atom. It has isostructural molybdenum and tungsten analogs. The Cr–Cr distance is 2.214(1) Å.104 There are two methods of preparation, one from CrCl3 and Na2C8H8105 and the other by passing chromium atom vapor into C8H8.106

Chromium Compounds 61
Cotton
The only compound with four-membered ligands, 1,2-(NMe2)(CH2)C6H4, bridging the two chromium atoms is also an organometallic compound. It contains two of the above ligands and two CH3CO2 and has the structure107 shown in Fig. 3.24, with a Cr–Cr distance of 1.870(1) Å.
Fig. 3.24. The structure of trans-Cr2(O2CCH3)2[1,2-(NMe2)(CH2)C6H4]2.
A curious, and unique organometallic compound108 is Cr2(CH2SiMe3)2(µ-CH2SiMe3)2(PMe3)2, with the structure shown schematically in Fig. 3.25.
Fig. 3.25. A schematic depiction of the molecule Cr2(CH2SiMe3)2(µ-CH2SiMe3)2(PMe3)2.
3.3.3 Other pertinent results
Preparation of the Cr2(tmtaa)2 molecule,109,110 Fig. 3.26, is rather easy, there being five distinct methods, and it is very stable. The Cr–Cr bond (length 2.10(1) Å) appears to be only a triple bond on the basis of MO calculations.109 The reported partial paramagnetism110 may imply a singlet-triplet equilibrium, but a study of the temperature dependence is needed. The eclipsed conformation of the two N4Cr groups is probably a consequence of the nesting of the two macrocyclic rings. Cr2(tmtaa)2 is the only Cr–Cr bonded molecule that is indubitably without bridging ligands (i.e., it is not a paddlewheel).
Fig. 3.26. The structure of the Cr2(tmtaa)2 molecule.

62Multiple Bonds Between Metal Atoms Chapter 3
In the presence of excess pyridine, the molecule splits and when THF is added in large excess the dinuclear species forms again, as shown in the following equation:
|
+4py |
|||
Cr2(tmtaa)2 |
|
|
|
2 trans-Cr(tmtaa)py2 |
|
|
|
||
|
|
|
||
|
-4py |
Based on these observations the energy of the Cr–Cr bond may be expressed as a multiple of the Cr–py bond energy. If ¨G is about zero, the Cr–Cr bond enthalpy would be more than four times the Cr–py bond enthalpy, allowing for the fact that T¨S must be positive (by perhaps 5-10 kcal mol-1) and from each broken Cr–Cr bond four Cr–py bonds are formed.
An attempt has been made to make a molecule with a Cr–Cr bond but only two bridges. A molecule with the desired stoichiometry, Cr2Cl4(dmpm)2, was made,111 but it is highly paramagnetic and has the structure shown in 3.26 with a Cr–Cr distance of 3.24 Å. No [Cr2X8]4- or Cr2X4L4 molecules, analogous to those formed by molybdenum, tungsten, technetium or rhenium appear to exist. All reported compounds of the stoichiometry CrX2L2, MCrX3 and M2CrX4 are paramagnetic, with four unpaired electrons per Cr atom.
PP
|
Cl |
Cl |
|
Cr |
Cr |
Cl |
Cl |
|
|
|
PP
3.26
Divergent-bite ligands.112
Most paddlewheel complexes with four N–C–N type bridging ligands tend to have short Cr–Cr bonds and no axial ligands. However, the short Cr–Cr bonds are possible only when the bridging ligands can have a sufficiently small “bite”, as in the amidinates, where the nitrogen lone pairs naturally point approximately along parallel lines, and can even toe in somewhat without much strain. However, there are some N–C–N type ligands for which the ligand structure dictates that the preferred bonding directions for the nitrogen atoms naturally diverge and toeing in is resisted. This is always true when a 6-membered ring and a 5-membered ring are fused with one nitrogen atom in each, as in 3.27, 3.28, and 3.29. There is enough flexibility in ligand 3.27 (CHIP) so that Cr2(CHIP)4 shows only moderate lengthening of the Cr–Cr bond. In two different crystalline compounds the bond lengths are 2.016 Å and 2.125(2) Å. The molecule containing the shorter of these Cr–Cr bonds is shown in Fig. 3.27.
H
N
N N |
N N |
N N |
3.27 |
3.28 |
3.29 |
With the 7-azaindolate ligand, azin, 3.28, the tendency of the ligand to lengthen the Cr–Cr bond has a dominant effect. As indicated in Fig. 3.28, for a typical Cr–N bond length, the placement of Cr atoms exactly in the directions expected for the nitrogen donor orbitals would lead to a very long distance indeed. In the first Cr24+ compound reported with four azin ligands,113 there are also two axial interactions with DMF ligands and the Cr–Cr distance is very long, 2.604(2) Å.

Chromium Compounds 63
Cotton
Fig. 3.27. The structure of Cr2(CHIP)4.
Fig. 3.28. Calculated geometric parameters for an azin ligand with a typical Cr–N bond of 2.10 Å.
A compound114 containing the [Cr2(azin)4Cl2]2- ion (Cr···Cl = 2.606 Å) has also been examined, and here, too, there is probably no Cr–Cr bond, since the Cr···Cr distance is 2.688(2) Å. This structure is shown in Fig. 3.29. One feature of interest, however, is that the azin ligands all show end-for-end disorder, with superposed 5- and 6-membered rings showing up in each ring position. Azin shows this tendency in other M2(azin)4 compounds, and to avoid it was one of the reasons why the carb ligand was employed in the compound mentioned earlier.
Fig. 3.29. The structure of the Cr2(azin)4Cl22- anion.
The carboline (carb) ligand, 3.29 is very rigid and gives two compounds with much longer Cr–Cr distances and with an axial Cl- ligand at one end. One of these, [Cr2(carb)4Cl]-, shown in Fig. 3.30, has a Cr–Cr distance of 2.5301(1) Å and the other, Cr2(carb)4Cl···Li(acetone) has a distance of 2.517(1) Å.

64Multiple Bonds Between Metal Atoms Chapter 3
Fig. 3.30. The structure of Cr2(carb)4.
Two other complexes with divergent-bite ligands contain the ligands oxindolate,115 (Cr–Cr = 2.495(4) Å) and saccharinate,116 (Cr–Cr = 2.550(4) Å and 2.591(1) Å in two different compounds.
The compound 3.30 has been isolated in two crystal modifications117 but the molecule has essentially the same structure in both with a Cr–Cr distance of 1.874[2] Å. The structure is not at all surprising, but the 1H NMR spectrum of the compound displays a very interesting feature not seen anywhere else. The exceptionally high diamagnetic anisotropy of quadruple bonds has been established in many compounds on the basis of the large downfield shift of protons (such as the methine proton in a formamidinate) that are located over the center of the M–M bond. In 3.30, the protons on the amino nitrogen atoms are located in a region of space where a large upfield shift would be expected and that is what is observed. The signal is shifted 3.0 to 4.5 ppm from where it would normally have been expected.
Ph |
|
|
Ph |
N |
N |
N |
|
H |
Cr |
Cr |
2 |
|
H |
||
|
|
|
|
|
N |
N |
N |
|
Ph |
|
Ph |
2
3.30
A-frames.
Two Cr24+ compounds with A-frame structures are known,82,84 namely, those shown in 3.31. In spite of the perturbation of the regular paddlewheel structure, both retain very short Cr–Cr distances, 1.940 (1) Å and 1.940(2) Å for the o-Cl and o-Br compounds, respectively. In order to allow this the Cr–Cl–Cr angles are extremely small, namely, about 46.7°. These are the most acute angles ever observed in an M–Cl–M unit.
3.31