
Multiple Bonds Between Metal Atoms / 04-Molybdenum Compounds
.pdf
Molybdenum Compounds 159
Cotton
compounds analogous to the one linked by the terephthalic acid dianion were obtained.576 In these molecules the dimolybdenum units are far apart and there is considerable non-planarity in the linkers. Thus, it is not surprising that the ¨E1/2 values (c. 100 mV) are relatively small.
The linkers B3-B6 provide more interesting results. In Table 4.16 the bond-like lines projecting from the N and O atoms indicate the directions in which bonds may be formed to the Mo24+ units. B3 and B4 correspond to the same orientation (designated _) of the central C–C bond as in the oxalate linker, whereby a separate 5-membered ring is formed about each Mo2 group. B5 and B6 lead to the formation of a 6-membered ring about each Mo2 group, with the two rings fused along a common C–C bond. This arrangement is designated `. It has not been seen with the oxalate linker.
The structures of the _ and ` isomers formed by the B4 and B6 linkers are shown in Fig. 4.34. In the _ isomer it may be noted that the two Mo2 units are perpendicular, whereas in the oxalate-bridged molecule they are coplanar.
_
`
Fig. 4.34. The structures of _ and ` isomers formed by the diamidate linkers B4 and B6.
As the data in Table 4.17 show, there is a major difference in the abilities of the _ and ` diamidate linkers to couple the Mo2 redox centers, far beyond what could be attributed to
the small difference in the Mo2 to Mo2 distances in these isomers. The ¨E1/2 values for the _ isomers (c. 190 mV) are about the same as ¨E1/2 for the oxalate linked compound (212 mV)503, as expected. The C–C single bond connecting the two halves of the molecule is a barrier to
communication. In the ` structure communication is greatly enhanced by the existence of a naphthalene-like / system.
There are five compounds in which cyclic diamidate dianions, B7-B11, are the linkers.502 These provide a range of ¨E1/2 values indicative of relatively weak coupling (B7, B11) to moderate (B8, B10) to fairly strong (B9). No detailed explanation for these variations has been given.

160Multiple Bonds Between Metal Atoms Chapter 4
Tetrahedral linkers.
Five tetrahedral linkers, shown in Table 4.16 as C1-C5 have been investigated. The first three compounds578 which have sulfate, molybdate and tungstate ions as linkers have shorter Mo2 to Mo2 distances than any found in compounds with dicarboxylate or diamidate linkers. It should be noted that in all cases, a tetrahedral linker requires the two Mo24+ units to be orthogonal to each other. For the compounds with C1, C2 and C3 linkers, no oxidized products have yet been isolated.
The linkers C4 and C5 are remarkable in that there is no evidence for their independent existence. Instead, they are both formed and trapped between the dimolybdenum units when Mo2(DAniF)3+, ZnCl2 (or CoCl2) and NaOCH3 are all present in acetonitrile solution and the products crystallize out.579 In each of the five cases, the ¨E1/2 values (Table 4.17) indicate that the coupling of the [Mo2] units, although better than for any dicarboxylate linker, is only moderate. In accord with this, structural studies580 show that the monocations of the compounds with both the C4 and C5 linkers are localized. The monocation with C4 is shown in Fig. 4.35. Thus, in the C4 case there are Mo–Mo distances of 2.116 Å and 2.151 Å, and in the C5 case they are 2.113 Å and 2.151 Å. Localization is confirmed by EPR measurements.
In the doubly-oxidized zinc-bridged compound, the two Mo25+ distances are 2.147(1) and 2.151 Å. EPR and magnetic susceptibility data fully support the idea that there is only negligible communication between the Mo2n+ units through these (MeO)2M(OMe)22− linkers. The +2 cation behaves as a simple diradical with neither ferromagnetic nor antiferromagnetic coupling.
Fig. 4.35. The structure of the [(DAniF)3Mo2]2[Zn(OMe)4] monocation.
4.5.4 Squares: four linked pairs
By a simple adaptation of the synthetic methods just described for making molecules with two linked Mo24+ units, a general method for making molecules with four linked Mo24+ units was devised.582 The first reported compounds were the oxalato-bridged molecule, along with those having the dianions of tetrafluoroterephthalic acid and ferrocenedicarboxylic acid (A1, A4 and A6 in Table 4.16). The procedure is summarized in the following equation:
4[Mo(DAniF)2(CH3CN)4](BF4)2 + 4(Bun4N)2O2CXCO2 Α
[Mo(DAniF)2(O2CXCO2)]4 + 8(Bun4N)(BF4)
Shortly thereafter,583 squares with linkers A2, A3, A5 and A20 (Table 4.16) were also reported. Those that have been structurally characterized are listed in Table 4.18. The structures of four representative squares are shown in Fig. 4.36.

Molybdenum Compounds 161
Cotton
Table 4.18. Structural data for molecular squares with Mo2(DAniF)3+ corners
Linkers |
Mo–Mo distance (Å) |
ref. |
|
A1a |
2.087(1) |
2.094(1) |
582,583 |
A3a |
2.087(1) |
2.089(1) |
583 |
A6a |
2.084(1) |
2.075(1) |
582,583 |
A20a |
2.075(4) |
2.082(3) |
583 |
|
2.082(4) |
2.089(4) |
|
CO32- |
2.092(4) |
2.098(2) |
584 |
a As listed in Table 4.16.
Fig. 4.36. Four molecular square molecules and their crystal stacking patterns.

162Multiple Bonds Between Metal Atoms Chapter 4
The electrochemistry of each of the seven reported squares has been examined, but a satisfactory understanding of the results is lacking. For example, for the oxalato square, three oneelectron oxidations are clearly resolved. The separation, ¨E1/2, between the first (407 mV) and
second (567 mV) is only 160 mV as compared to a ¨E1/2 for Mo2(DAniF)3(O2CCO2)Mo2(DAniF)3 of 212 mV. As shown in Fig. 4.37, there are two possible sequences for the successive oxidation
steps. In comparing these sequences with each other as well as with the results for the oxalate square the following points arise:
a.The main difference is in step (2) and it could be argued that the lower ¨E1/2 just mentioned for the square favors the assumption of sequence A.
b.However, the ¨E1/2 pertinent to step (3) for the oxalate equals only 94 mV, and this is not to be expected for either sequence.
c.There is no indication that step (4) occurs.
Fig. 4.37. Two possible sequences for the successive oxidations of a square.
In fact, step (4) has not been observed for any of the seven squares, and with linkers that are expected to give weaker coupling steps (2) and (3) are practically undifferentiated. For the O2CC>CCO2 bridged square, for example, there is a one-electron oxidation at 518 mV and then two overlapping oxidations at 621 mV. In cases of even weaker coupling, as illustrated by the O2CC6F4CO2 square, steps (1), (2) and (3) are all undifferentiated. Communication occurring in the squares clearly needs further study.
Another characteristic of all the squares is the stacking of the molecules in their crystals. This is illustrated for four of them in Fig. 4.36. In three of these the squares are stacked “in register,” and this is the usual pattern. However, for the O2CC6H4C6H4CO square there is an alternation from one level to the next. In many of the stacks small solvent molecules (e.g., CH2Cl2, toluene) are present in the interior, sometimes ordered and sometimes not.
An atypical square585 is shown in Fig. 4.38. The linkers are carbonate ions and one ligand position on each Mo24+ unit is occupied by a molecule of H2O. This is the only molecular square for which all four successive oxidations have been observed. The first two presumably arise from oxidations at opposite corners and the last two at the remaining corners.
4.5.5 Loops: two pairs doubly linked
The use of inherently bent linkers to form loops has been demonstrated in six instances.586-588 The six linkers used are shown in Table 4.16 (A7, A14, A21, A23-A25) and the compounds are listed in Table 4.19. Linkers A14, A21 and A25 are chiral; the loops made with A14 and A21 are racemic (one R and one S linker), but in the loop made with A25 both linkers in the same molecule have the same (RR or SS) chirality. In all cases, the loops are stacked in the crystals. Fig. 4.39 shows the molecular structure of the chiral loop made with the ligand A25. The stereochemistry of this loop is rather unusual.587 The molecule is a second-order Möbius strip, that is, a Möbius type ring with two twists rather than just one. It may be noted that linker A13 should be able to form a loop, but this has not been attempted.

Molybdenum Compounds 163
Cotton
Fig. 4. 38. The core of the square formed by four [(DAniF)2(H2O)Mo2]2+ units and four carbonate ions.
Table 4.19. Properties of loops
Linkera |
|
Distances, Å |
¨E1/2, mV |
ref. |
||
Mo–Mo bond distancesb |
Mo2···Mo2 separation |
|||||
|
|
|
||||
A7 |
2.088 |
|
6.51 |
109 |
586 |
|
A14 |
2.098 |
|
8.19 |
80 |
588 |
|
A21 |
2.088 |
|
na |
irrev |
586 |
|
A23 |
2.086 |
2.094 |
9.62 |
91 |
586 |
|
A24 |
2.088 |
2.092 |
6.27 |
179 |
586 |
|
A25 |
2.081 |
|
na |
<70 |
587 |
a See Table 4.16.
b If the Mo2 units are crystallographically independent both are given.
Fig. 4. 39. The chiral structure of the molecular loop molecule formed by [(DAniF)2Mo2]2+ units with two SS linkers of type A25 (Table 4.16).
There are two cases where the same linker has been employed to make a pair with two Mo2(DAniF)3 units and also a loop with two Mo2(DAniF)2 units. As shown by the following comparisons, this seems to make very little difference in the communication between the two

164Multiple Bonds Between Metal Atoms Chapter 4
Mo24+ units. Even though in the loops the number of linkers is doubled and the distances are c. 1.2 Å shorter, the communication (¨E1/2) is either no better or poorer.
Table 4.19. Comparison of Loops and Pairs
Linker |
Mo2···Mo2 distance |
¨E1/2, mV |
||
malonate |
pair |
7.65 |
108 |
|
loop |
6.51 |
109 |
||
|
||||
allenedicarboxylate |
pair |
9.40 |
130 |
|
loop |
8.19 |
80 |
||
|
4.5.6 Rectangular cyclic quartets
The title of this section refers to molecules in which two Mo24+ units are linked into a rectangle. There are four types as shown schematically in Fig. 4.40. Note that in (a) and (c) the quadruple bonds persist, in (b) there is partial oxidation and in (d) there has been a cyclic 2+2 addition to give a metallacyclobutadiyne ring. Known compounds of types (a) and (c) and key structural data are listed in Table 4.20.
Fig. 4.40. Four structural types of rectangular cyclic arrays in which Mo2n+ units are linked by small bridging anions.
Table 4.20. Rectangular cyclic compounds of types (a) and (c)
Compound |
Mo–Mo, Å |
Mo2···Mo2, Å |
|
Type (a) structures |
|
[Mo2(DTolF)3]2(µ-H)2 |
2.093(1) |
3.532(1) |
[Mo2(DAni)3]2(µ-H)2 |
2.086(4) |
3.48(1) |
[Mo2(DTolF)3]2(µ-OH)2 |
2.107(1) |
4.073(1) |
|
Type (c) structures |
|
[Mo2(DTolF)2]2(µ-Cl)4 |
2.118(1) |
3.592(1) |
[Mo2(DPhfF)2]2(µ-Cl)4 |
2.123(1) |
3.563(1) |
[Mo2(DAniF)2]2(µ-Cl)4 |
2.119(1) |
3.601(1) |
[Mo2(DAniF)2]2(µ-Br)4 |
structure not reported |
|
[Mo2(DAniF)2]2(µ-I)4 |
2.117(1) |
3.915(1) |
[Mo2I2(PBun3)2]2(µ-I)4 |
2.129(3) |
3.998(3) |
In type (a) X may be H (with either DAniF or DTolF as spectator ligands) or OH (with DTolF). 393,589,590 The structure of [Mo2(DAniF)3]2(µ−H)2 is shown in Fig. 4.41. The hydrido compounds resist hydrolysis by water but are decomposed by acid. The presence of the
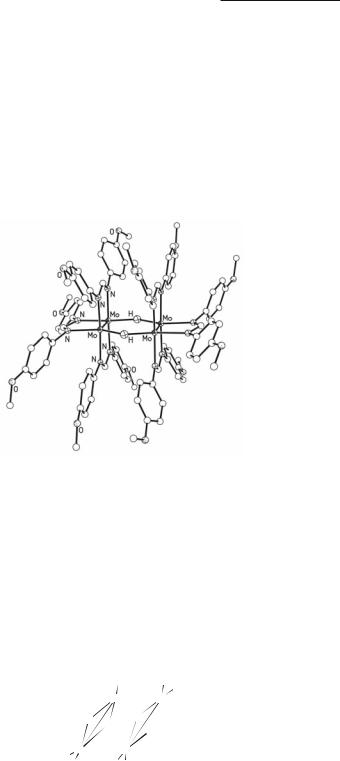
Molybdenum Compounds 165
Cotton
hydrogen atom bridges has been confirmed by neutron diffraction (Mo–H = 1.84(2) Å). The compounds are obtained in 60-70% yield by the reaction:
2Mo2(DArF)3Cl2 + 4NaHBEt3 Α Mo2(DArF)3(µ-H)2Mo2(DArF)3
The OH-bridged product was obtained when Mo2(DTolF)3Cl2 was reduced by metallic potassium in the presence of KOH. It is converted by atmospheric oxygen to the di-oxo compound,393 in which the dimolybdenum units are [Mo2(DTolF)3]2+. Since the oxo-bridged compound displays a normal 1H NMR spectrum the electron spins in these two units are coupled across the µ-O bridges. In the OH-bridged compound393,590 the Mo–Mo quadruple bond lengths are 2.107(1) Å, whereas in the O-bridged molecule, the Mo–Mo distances of 2.140(2) Å are consistent with the bond order of 3.5.
Fig. 4.41. The structure of the [(DAniF)3Mo2]2(µ-H)2 molecule as determined by neutron diffraction.
The first compounds with a central structure of type (d) were reported by McCarley and coworkers,365 who obtained both Mo4Cl8(PBun3)4 and Mo2Cl8(PEt3)4 by an indirect route commencing with Mo2Cl4(PPh3)2(CH3OH)2. The second of these compounds consists of Mo4Cl4(PEt3)4(µ-Cl)4 molecules, in which the arrangement of Cl and PPh3 ligands is as shown in 4.42. Later on it was recognized591 that these centrosymmetric molecules are actually packed in a disordered fashion, as shown in Fig. 4.42. The report of Mo4Cl4(PEt3)4(µ-Cl)4 was followed several years later592 by the development of other synthetic methods and the report of six more compounds of the same type, although none was structurally confirmed. Soon after that Mo2Cl4[P(OMe)3]4(µ-Cl)4 was obtained593 and shown by crystallography to have the 4.41 type structure.
|
|
Cl P |
|
P Cl |
||
|
|
Mo |
|
|
Mo |
|
|
|
|
|
|||
|
|
|
|
|||
Cl |
|
|
Cl |
|
|
|
Cl |
|
|
||||
|
|
Cl |
||||
|
|
|
|
|
||
Mo |
|
|
Mo |
|
|
|
|
|
|
|
|||
|
|
|
|
|||
Cl |
P Cl |
|
|
|||
P |
|
|
4.41

166Multiple Bonds Between Metal Atoms Chapter 4
Fig. 4.42. The disordered packing of [Mo2(PEt3)2Cl2]2(µ-Cl)4 molecules. The minor orientation is shown by broken lines.
In all of the molecules with structures of type (d) two quadruple Mo–Mo bonds originally present in the starting materials have combined in a 2+2 cyclo addition to generate a rectangle of molybdenum atoms in which Mo–Mo single bonds bridged by µ-Cl or other µ-X atoms have been formed. In place of the initial μ2/4β2 bonds there now remain μ2/4 triple bonds. The newly formed single bonds have Mo–Mo distances of about 2.90 Å (i.e., much shorter than the non-bonded distances of c. 3.60 Å in type (c) structures) and the remaining Mo–Mo triple bond lengths are c. 2.21 Å.
Although the Mo4I4(PBun3)4(µ-I)4 molecule was prepared in the expectation that it too would have a structure of type (d), its properties led to the suggestion592 that it might actually be of type (c). A later X-ray study confirmed this.393 The reason it does not conform is presumably that the large µ-I atoms make it impossible for the Mo–Mo single bonds to be formed, as in (d), and thus the Mo–Mo quadruple bonds remain intact, as in (c).
Two unique molecules resulted from attempts to employ dmpm or dppm instead of monodentate neutral ligands in forming cyclobutadiyne type molecules.594 The preparations were carried out in methanol and µ-OCH3 groups are present in the products. In the simpler case Mo2Cl4(µ-dppm)2(µ-Cl)2(µ-OCH3)2 was formed, in which the Mo–Mo triple bond distances are 2.224(1) Å and the Mo–Mo single bonds, each bridged by µ-Cl and µ-OCH3, have lengths of 2.814(1) Å. In the other case, Mo4Cl4(µ-dmpm)2(µ-Cl)3(µ-OCH3)3(µ4-O) there is a quadruply bridging oxygen atom; two of the Mo–Mo distances are about 2.45 Å and the others are c. 2.65 Å and 2.71 Å. The electronic structure was not discussed.
One final point should be noted. All of the rectangular compounds discussed here are made from quadruply-bonded Mo24+ starting materials. There are also some rectangular Mo4 compounds that are made from Mo26+ triply-bonded starting materials, in which the Mo>Mo bonds are retained and there are no Mo–Mo single bonds but only pairs of µ-X atoms linking the Mo>Mo moieties. Such compounds are discussed in Chapter 6.
4.5.7 Other structural types
The most interesting trifunctional linker is the anion of trimesic acid, 4.42. It readily combines with three Mo2(DAniF)3+ units to form the structure595 shown in Fig. 4.43. For this molecular propeller, three strongly overlapping electrochemical oxidations have been observed with a separation of only 112 mv from the first to the third, indicating relatively poor communication through the trimesate ion.

Molybdenum Compounds 167
Cotton
OO
CC
OO
C
OO
4.42
Fig. 4. 43. The “molecular propeller”, in which three (DAniF)3Mo2+ units are attached to a central trimesate anion, 1,3,5-C6H3(CO2−)3.
A more impressive result was obtained596,597 when 4.42 is combined with Mo2(DAniF)22+ in the ratio of 4 to 6 to give the structure shown in Fig. 4.44. This remarkable structure, which has a rhodium analog, is a concentric superposition of an octahedron (vertices at the centers of Mo24+ units) and a tetrahedron (vertices at the centers of the trimesate rings). A number of oxidations were seen in the CV/DPV scans, but so bunched together in a range of about 250 mV that an interpretation was not possible.
Fig. 4.44. The core structure of the tetrahedral/octahedral molecule that assembles four trimesate anions and six (DAniF)2Mo22+ ions.
The participation of the carbonate ion in the formation of a square has already been mentioned (Section 4.5.4). In that case its inherent three-fold symmetry does not influence the sym-

168Multiple Bonds Between Metal Atoms Chapter 4
metry of the product. On the other hand, the carbonate ion has been used to make molecules161 of the type shown schematically as 4.44. Strong coupling among the three dimolybdenum components might be expected but, unfortunately, the electrochemistry of these molecules was not investigated.
N
PP
|
Mo |
|
Mo |
|
|
X = Cl, Br, I |
||
|
X |
|
|
|
|
X |
|
|
|
|
|
|
|
|
CF3CO2 groups |
||
|
O |
|
O |
|
|
|||
|
|
|
|
above and below |
||||
|
Mo |
C |
Mo |
|
||||
|
|
are omitted |
||||||
|
P |
|
|
|
|
P |
||
|
|
|
|
|
|
|||
N |
Mo |
O |
|
|
N |
|||
|
|
Mo |
|
|
||||
|
|
|
|
|
||||
|
P |
|
|
|
|
P |
|
|
|
|
|
|
X |
|
|
|
4.43
References:
1.D. Lawton and R. Mason, J. Am. Chem. Soc. 1965, 87, 921.
2.F. A. Cotton, Z. C. Mester and T. R. Webb, Acta Crystallogr. 1974, B30, 2768.
3.J. V. Brencˇic and F. A. Cotton, Inorg. Chem. 1969, 8, 7.
4.E. W. Abel, A. Singh and G. Wilkinson, J. Chem. Soc. 1959, 3097.
5.E. Bannister and G. Wilkinson, Chem. Ind. (London) 1960, 319.
6.T. A. Stephenson, E. Bannister and G. Wilkinson, J. Chem. Soc., 1964, 2538.
7.G. Holste and H. Schafer, Z. anorg. allg. Chem. 1972, 391, 263.
8.G. Holste, Z. anorg. allg. Chem. 1975, 414, 81.
9.E. Hochberg, P. Walks and E. H. Abbott, Inorg. Chem. 1974, 13, 1824.
10.A. B. Brignole and F. A. Cotton, Inorg. Synth. 1972, 13, 81.
11.R. E. McCarley, J. L. Templeton, T. J. Colburn, V. Katovic and R. J. Hoxmeier, Adv. Chem. Ser. 1976, No. 150, 318.
12.F. A. Cotton and J. G. Norman, Jr, J. Coord. Chem. 1971, 1, 161.
13.F. A. Cotton, J. G. Norman, Jr, B. R. Stults and T. R. Webb, J. Coord. Chem. 1976, 5, 217.
14.R. J. Mureinik, J. Inorg. Nucl. Chem. 1976, 38, 1275.
15.F. A. Cotton and J. L. Thompson, Inorg. Chem. 1981, 20, 3887.
16.F. A. Cotton, L. R. Falvello, A. H. Reid, Jr and J. H. Tocher, J. Organomet. Chem. 1987, 319, 87.
17.G. M. McCann and H. Ryan, Inorg. Chim. Acta 1987, 133, 11.
18.A. Carvill, P. Higgins, G. M. McCann, H. Ryan and A. Shiels, J. Chem. Soc., Dalton Trans. 1989, 2435.
19.(a) R. H. Cayton, M. H. Chisholm and F. D. Darrington, Angew. Chem., Int. Ed. Engl. 1990, 29, 1481. (b) R. H. Cayton and M. H. Chisholm, J. Am. Chem. Soc. 1989, 111, 8921. (c) R. H. Cayton,
M.H. Chisholm, J. C. Huffman and E. B. Lobkovsky, Angew. Chem., Int. Ed. Engl. 1991, 30, 862.
20.A. W. Coleman, J. C. Green, A. J. Hayes, E. A. Seddon, D. R. Lloyd and Y. Niwa, J. Chem. Soc., Dalton Trans. 1979, 1057.
21.E. Carmona, A. Galindo, L. Sánchez, A. J. Nielson and G. Wilkinson, Polyhedron, 1984, 3, 347.
22.D. J. Santure and A. P. Sattelberger, Inorg. Chem. 1985, 24, 3477.
23.F. A. Cotton, L. R. Falvello and C. A. Murillo, Inorg. Chem. 1983, 22, 382.
24.R. J. H. Clark, A. J. Hempleman and M. Kurmoo, J. Chem. Soc. 1988, 973.
25.See for example: F. A. Cotton, Polyhedron, 1986, 5, 3 and references cited therein.
26.F. A. Cotton, L. M. Daniels, P. A. Kibala, M. Matusz, W. J. Roth, W. Schwotzer, W. Wang and
B.Zhong, Inorg. Chim. Acta 1994, 215, 9.