
- •VOLUME 3
- •CONTRIBUTOR LIST
- •PREFACE
- •LIST OF ARTICLES
- •ABBREVIATIONS AND ACRONYMS
- •CONVERSION FACTORS AND UNIT SYMBOLS
- •EDUCATION, COMPUTERS IN.
- •ELECTROANALGESIA, SYSTEMIC
- •ELECTROCARDIOGRAPHY, COMPUTERS IN
- •ELECTROCONVULSIVE THERAPHY
- •ELECTRODES.
- •ELECTROENCEPHALOGRAPHY
- •ELECTROGASTROGRAM
- •ELECTROMAGNETIC FLOWMETER.
- •ELECTROMYOGRAPHY
- •ELECTRON MICROSCOPY.
- •ELECTRONEUROGRAPHY
- •ELECTROPHORESIS
- •ELECTROPHYSIOLOGY
- •ELECTRORETINOGRAPHY
- •ELECTROSHOCK THERAPY.
- •ELECTROSTIMULATION OF SPINAL CORD.
- •ELECTROSURGICAL UNIT (ESU)
- •EMERGENCY MEDICAL CARE.
- •ENDOSCOPES
- •ENGINEERED TISSUE
- •ENVIRONMENTAL CONTROL
- •EQUIPMENT ACQUISITION
- •EQUIPMENT MAINTENANCE, BIOMEDICAL
- •ERGONOMICS.
- •ESOPHAGEAL MANOMETRY
- •EVENT-RELATED POTENTIALS.
- •EVOKED POTENTIALS
- •EXERCISE FITNESS, BIOMECHANICS OF.
- •EXERCISE, THERAPEUTIC.
- •EXERCISE STRESS TESTING
- •EYE MOVEMENT, MEASUREMENT TECHNIQUES FOR
- •FETAL MONITORING
- •FETAL SURGERY.
- •FEVER THERAPY.
- •FIBER OPTICS IN MEDICINE
- •FICK TECHNIQUE.
- •FITNESS TECHNOLOGY.
- •FIXATION OF ORTHOPEDIC PROSTHESES.
- •FLAME ATOMIC EMISSON SPECTROMETRY AND ATOMIC ABSORPTION SPECTROMETRY
- •FLAME PHOTOMETRY.
- •FLOWMETERS
- •FLOWMETERS, RESPIRATORY.
- •FLUORESCENCE MEASUREMENTS
- •FLUORESCENCE MICROSCOPY.
- •FLUORESCENCE SPECTROSCOPY.
- •FLUORIMETRY.
- •FRACTURE, ELECTRICAL TREATMENT OF.
- •FUNCTIONAL ELECTRICAL STIMULATION
- •GAMMA CAMERA.
- •GAMMA KNIFE
- •GAS AND VACUUM SYSTEMS, CENTRALLY PIPED MEDICAL
- •GAS EXCHANGE.
- •GASTROINTESTINAL HEMORRHAGE
- •GEL FILTRATION CHROMATOGRAPHY.
- •GLUCOSE SENSORS
- •HBO THERAPY.
- •HEARING IMPAIRMENT.
- •HEART RATE, FETAL, MONITORING OF.
- •HEART VALVE PROSTHESES
- •HEART VALVE PROSTHESES, IN VITRO FLOW DYNAMICS OF
- •HEART VALVES, PROSTHETIC
- •HEART VIBRATION.
- •HEART, ARTIFICIAL
- •HEART–LUNG MACHINES
- •HEAT AND COLD, THERAPEUTIC
- •HEAVY ION RADIOTHERAPY.
- •HEMODYNAMICS
- •HEMODYNAMIC MONITORING.
- •HIGH FREQUENCY VENTILATION
- •HIP JOINTS, ARTIFICIAL
- •HIP REPLACEMENT, TOTAL.
- •HOLTER MONITORING.
- •HOME HEALTH CARE DEVICES
- •HOSPITAL SAFETY PROGRAM.
- •HUMAN FACTORS IN MEDICAL DEVICES
- •HUMAN SPINE, BIOMECHANICS OF
20.Noon GP, BallJr JW, Papaconstantinou HT. Clinical experience with BioMedicus centrifugal ventricular support in 172 patients. Artif Organs 1995;19(7):756–760.
21.Noon GP, Lafuente JA, Irwin S. Acute and temporary ventricular support with BioMedicus centrifugal pump. Ann Thorac Surg 1999;68(2):650–654.
22.Williams M, Oz M, Mancini D. Cardiac assist devices for endstage heart failure. Heart Dis 2001;3(2):109–115.
23.Bourque K, et al. HeartMate III: pump design for a centrifugal LVAD with a magnetically levitated rotor. ASAIO J 2001;47(4):401–405.
24.Bourque K, et al. Incorporation of electronics within a compact, fully implanted left ventricular assist device. Artif Organs 2002;26(11):939–942.
25.Chen C, et al. A magnetic suspension theory and its application to the HeartQuest ventricular assist device. Artif Organs 2002;26(11):947–951.
26.Terumo Heart, Inc. Press Release, Jan. 19, 2004 [Online] : Available at http://www.terumocvs. com/newsandevents/rendernews.asp?newsId=5. Terumo [5/17/2005]. Accessed 2005.
27.Doi K, et al. Preclinical readiness testing of the Arrow International CorAide left ventricular assist system. Ann Thorac Surg 2004;77(6):2103–2110.
28.James NL, et al. Implantation of the VentrAssist Implantable Rotary Blood Pump in sheep. ASAIO J 2003;49(4):454–458.
29.Arrow International-Cardiac Assist-CorAide [online] Arrow International. Available at http://www.arrowintl.com/products/cardassist/. [2/28/05]. Accessed 2003.
30.Ventracor Half-Year Report Summary, Feb 16 2005 [online] Ventracor. Available at http://www.ventracor.com/default.asp?cp¼/ news/newsitem.asp%3FnewsID%3D316. [5/19/2005]. Accessed 2005.
31.Goldstein DJ. Worldwide experience with the MicroMed DeBakey Ventricular Assist Device as a bridge to transplantation. Circulation 2003;108(Suppl 1):II272–277.
32.MicroMed Technology Press Release, Jan. 20 2005 [Online] MicroMed. Available at http://www.micromedtech.com/news/ 01-20-05.htm. [5/19/2005]. Accessed 2005.
33.Burke DJ, et al. The Heartmate II: design and development of a fully sealed axial flow left ventricular assist system. Artif Organs 2001;25(5):380–385.
34.Frazier OH, et al. First clinical use of the redesigned HeartMate II left ventricular assist system in the United States: a case report. Tex Heart Inst J 2004;31(2):157–159.
35.Westaby S, et al. The Jarvik 2000 Heart. Clinical validation of the intraventricular position. Eur J Cardiothorac Surg 2002;22(2):228–232.
36.Frazier OH, et al. Use of the Flowmaker (Jarvik 2000) left ventricular assist device for destination therapy and bridging to transplantation. Cardiology 2004;101(1–3):111–116.
37.Copeland JG, et al. Cardiac replacement with a total artificial heart as a bridge to transplantation. N Engl J Med 2004; 351(9):859–867.
38.DeVries WC. The permanent artificial heart. Four case reports. JAMA 1988;259(6):849–859.
39.Weiss WJ, et al. Steady state hemodynamic and energetic characterization of the Penn State/3M Health Care Total Artificial Heart. ASAIO J 1999;45(3):189–193.
40.Dowling RD, et al. The AbioCor implantable replacement heart. Ann Thorac Surg 2003;75(6 Suppl):S93–S99.
41.ABIOMED AbioCor Press Release, Nov. 4, 2004 [Online] ABIOMED. Available at http://www.abiomed.com/news/ Fourteenth-AbioCor-Patient.cfm. [5/19/2005]. Accessed 2004.
Reading List
Lee J, et al. Reliability model from the in vitro durability tests of a left ventricular assist system. ASAIO J 45(6):595–601 1999.
HEART–LUNG MACHINES |
459 |
Raman J, Jeevanadam V. Destination therapy with ventricular assist devices. Cardiology 101(1–3):104–110 2004.
Rosenberg G. Artificial Heart and Circulatory Assist Devices. In: Bronzino J, editor. The Biomedical Engineering Handbook, Boca Raton (FL): CRC Press; 1995: 1839–1846.
Song X, et al. Axial flow blood pumps. ASAIO J 49(4):355–364 2003.
Stevenson LW, Kormos RL. Mechanical Cardiac Support 2000: Current applications and future trial design. J Thorac Cardiovasc Surg 121(3):418–424 2001.
Zapanta CM, et al. Durability testing of a completely implantable electric total artificial heart. ASAIO J 51(3):214–223 2005.
See also HEART VALVE PROSTHESES; HEMODYNAMICS; QUALITY OF LIFE MEASURES, CLINICAL SIGNIFICANCE OF.
HEART–LUNG MACHINES
DAVID D’ALESSANDRO
ROBERT MICHLER
Montefiore Medical Center
Bronx, New York
INTRODUCTION
The heart–lung machine is perhaps the most important contribution to the advancement of surgery in the last century. This apparatus was designed to perform the functions of both the human heart and the lungs allowing surgeons to suspend normal circulation to repair defects in the heart. The development of a clinically safe and useful machine was the rate-limiting step to the development of modern cardiac surgery. Since its inception, the heart– lung machine has enabled the surgical treatment of congenital heart defects, coronary heart disease, valvular heart disease, and end-stage heart disease with heart transplantation and mechanical assist devices or artificial hearts.
The heart–lung machine consists of several components that together make up a circuit that diverts blood away from the heart and lungs and returns oxygenated blood to the body. Commercial investment and production of these components has resulted in wide variability in the design of each, but the overall concept is preserved. During an operation, a medical specialist known as a perfusionist operates the heart–lung machine. The role of the perfusionist is to maintain the circuit, adjust the flow as necessary, prevent air and particulate emboli from entering the circulation, and maintain the various components of the blood within physiologic parameters.
HISTORY OF CARDIAC SURGERY AND ASSISTED CIRCULATION
The emergence of modern heart surgery and the ability to correct congenital and acquired diseases of the heart were dependent on the work of innovative pioneers who developed a means to stop the heart while preserving blood flow to the remainder of the body. This new technology was aptly named cardiopulmonary bypass (CPB), which simply
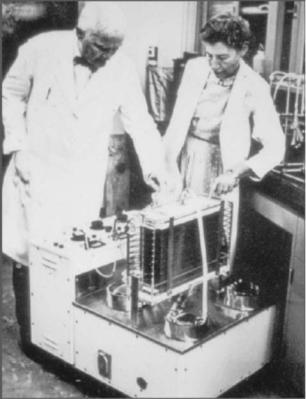
460 HEART–LUNG MACHINES
means circulating blood around the heart and lungs. Since the inception of the heart–lung machine, continued refinements and widespread adoption of CPB led to the rapid growth of congenital and adult heart surgery.
Lessons learned during World War II and corresponding advances in critical care emboldened surgeons to consider surgical solutions for diseases of the heart, an organ long considered to be inoperable. One such surgeon was Dr. Dwight Harken who pioneered closed heart surgery, which he initially used to remove foreign bodies from the heart such as bullets and shrapnel. Having achieved success with this approach, he and others modified the techniques, developing a blinded technique for performing closed valvuloplasty primarily used to ameliorate rheumatic valvular disease. Although this method proved safe and reproducible, its applicability to other diseases of the heart was limited.
The next leap came when surgeons attempted openheart procedures using brief periods of total circulatory arrest. As the brain is highly sensitive to hypoxic injury, very few patients were successfully treated with his approach. The safety of circulatory arrest was greatly increased when Dr. Bill Bigelow at the University of Minnesota introduced the concept of induced hypothermia. This method of lowering body temperature to reduce metabolic demand provided protection for the oxygen-starved organs allowing for modestly longer periods of circulatory arrest. Inspired by Bigelow’s work, Lewis and Taufic first used this approach clinically on September 2, 1952, at the University of Minnesota (1). Under moderate total body hypothermia, Lewis and Taufic used a short period of circulatory arrest to repair a congenital defect in a 5 year-old girl. This was a landmark achievement in surgery and marks the true beginning of open-heart surgery. For the first time, surgeons had the ability to open the heart to repair defects under direct vision. Despite this great achievement, however, the relatively brief periods of circulatory arrest that this technique provided were sufficient only for the repair of simple defects and did little to broaden the scope of surgically treatable cardiac diseases.
The development of assisted circulation was a quantum leap in the field of cardiac surgery. Although investigation into mechanical means of circulation began during the early part of the twentieth century, an effective and safe design would not emerge for several years. An alternative approach named cross-circulation was used for several years in the interim. Dr. C. Walt Lillehei, again at the University of Minnesota, was the first to use this technique clinically when on March 26, 1954, when he repaired a VSD in a 12 month-old infant. During this operation, the child’s mother acted as a blood reservoir, a pump, and an oxygenator, allowing a safe period of extended circulatory arrest to repair a complicated congenital defect. The child’s circulation was connected in series to her mother’s diverting oxygen poor blood away from the patient’s heart and lungs and returning oxygen-saturated blood to her arterial system. Although many were amazed by and congratulatory of Dr. Lillehei’s efforts, critics were outspoken of their disapproval of a technique that risked the death of two patients for the benefit of one. Nevertheless, these early successes provided proof of the concept and a mechanical substitute for cross-circulation soon followed.
Figure 1. John and Mary Gibbon with their heart–lung machine. (Reprinted with permission from Thomas Jefferson University Archives.)
John and Mary Gibbon are credited with building the first usable CPB machine, a prototype of which John Gibbon employed in 1935 to support a cat for 26 min (2) (Fig. 1). This pump, which used two roller pumps and other similar early designs, were traumatic to blood cells and platelets and allowed for easy air entry into the circulation, which often proved catastrophic. Later, in 1946, Dr. Gibbon in collaboration with Thomas Watson, then chairman of IBM, made further refinements. Together they were able to successfully perform open-heart surgery in dogs, supporting the animals for period exceeding 1 h (3). Finally on May 6, 1953, Dr. Gibbon used his heart–lung machine to successfully repair an atrial septal defect in an 18 year-old girl, marking the first successful clinical use of a heart–lung machine to perform open-heart surgery. Gibbon’s first attempt in 1952 followed two unsuccessful attempts by Clarence Dennis et al. in 1951 (4), and it too ended in failure. Sadly, subsequent failures led Gibbon to finally abandon this new technology, finally giving up heart surgery completely.
Kirklin’s group at the Mayo Clinic modified the Gibbon pump oxygenator and reported a series of eight patients in 1955 with a 50% survival (5). Around the same time, Frederick Cross and Earl Kay developed a rotating disk oxygenator that had a similar effectiveness (6,7). This apparatus was similar in design to that used by Dennis et al. several years earlier (8). While this Kay-Cross unit became commercially available, it shared many of the
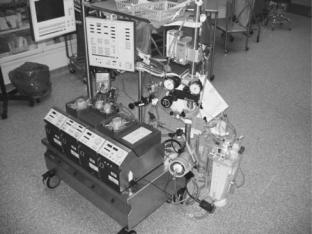
Figure 2. Typical cardiopulmonary bypass circuit demonstrating the various components. This circuit contains four accessory pumps for suction/venting and the administration of cardioplegia. (Reprinted with permission from Ref. 13.)
limitations of the Gibbon system. These pumps were cumbersome, difficult to clean and sterilize, and were inefficient and thus wasteful of blood.
Collaboration between Richard DeWall and Lillehei provided the next advance in pump oxygenators, and in 1956, they reported their first clinical experience using a bubble oxygenator in seven patients, five of whom survived
(9). Further refinements by Gott et al. (10) resulted in a reproducible, disposable plastic sheet oxygenator, a system that was readily accepted by pioneering centers around the world. The bubble oxygenator became the predominant pump oxygenator for the next three decades. More recently, the membrane oxygenator, an early design of which was described by Kolff et al. in 1956 (11), has replaced it. Lande et al. described the first commercially available disposable membrane oxygenator (12), but its advantages to the bubble oxygenator were not readily apparent. By the mid-to-late 1980s, advanced microporous membrane oxygenators began to supplant the bubble oxygenator in the clinical arena and they remain the predominant design in use today (Fig. 2).
THE CIRCUIT
The first requirement of a CPB circuit is a means to evacuate and return blood to the circulation. Special drainage and return catheters known as cannulae have been devised for this purpose. One or more venous cannulae are placed in a central vein or in the heart to empty the heart. The venous cannulae are connected to a series of poly(vinyl chloride) tubing that deliver the blood to the remainder of the circuit.
The next component is a reservoir that collects and holds the blood. The reservoir is often used to collect shed blood from the operative field and to store excess blood volume while the heart and lungs are stopped. Drainage of the blood is accomplished by gravitational forces and is sometimes assisted with vacuum. The movement of blood
HEART–LUNG MACHINES |
461 |
through the remainder of the circuit, however, requires the use of a pump. Many pump designs have been used over the years, but the most common in current use are the roller pump and the centrifugal pump. A roller pump is a positive displacement pump and has been the most prolific design to date for this purpose. These pumps use rollers mounted on the ends of a rotating arm that displace blood within the compressed tubing, propelling it forward with faint pulsatility. Both the rate and direction of rotation and the occlusion pressure can be adjusted to adjust the flow. In contrast, centrifugal pumps use a magnetically driven impeller design that create a pressure differential between the inlet and the outlet portion of the pump housing propelling blood. Less traumatic to the blood elements, the centrifugal pump has largely replaced the roller pump for central circulation at most large centers.
The blood is actively pumped through a series of components before it is returned to the body. A heat exchange system is used to first lower the blood and body temperature during heart surgery. Controlled hypothermia reduces the body’s energy demands, safely allowing reduced or even complete cessation of blood flow, which is necessary during certain times in the course of an operation. Reversing the process later rewarms the blood and body.
The pump oxygenator is by far the most sophisticated component of the circuit. As discussed, the membrane oxygenator is the predominant design in use today. Membrane oxygenators employ a microporous membrane that facilitates gas exchange in the circulating blood. Pores less than 1 mm prevent the leakage of serum across the membrane yet allow efficient gas exchange. An integrated gas mixer or blender enables the perfusionist to adjust the oxygen and carbon dioxide content in the blood and thus regulate the acid–base balance. In addition, an inhalational gas vaporizer provides anesthetic to the patient for the period during CPB. In line oxygen saturation monitors supplemented with frequent blood gas analysis ensure physiologic requirements are met during CPB.
Finally, an in line filter (typically 40 mm) prevents air and thromboembolic particles from returning to the arterial circulation. In parallel with this circuit, several additional roller pumps are used to provide suction that returns shed blood form the operative field. These are critical in open-heart procedures where a dry operative field is imperative for good visualization. An additional pump is often needed to deliver a cardioplegia solution, a mixture of blood and hyperkalemic solution used to stop the heart. Various monitors, hemofiltration units, blood sampling manifolds, gauges, and safety valves are usually present.
The main arterio-venous circuit is generally primed with a balanced electrolyte solution, which obviates the need for the blood prime used in the earlier, higher volume circuits. Other prime constituents include buffers such as sodium bicarbonate, oncotics such as albumin and mannitol, and anticoagulation in the form of heparin. Some protocols also call for the addition of an antifibrinolytic such as aminocaproic acid or aprotinin to the prime.
The overall construction of the CPB circuit is highly variable among institutions depending on the preferences of the surgeons and perfusionists. Although the principals of perfusion are universal, individual and group practices
462 HEAT AND COLD, THERAPEUTIC
are not. Perfusionists are a highly trained group of specialists dedicated to the safe operation of the heart–lung machine. Perfusion education and training has evolved concurrently with improvements in the bypass circuit, and accredited perfusion programs are included in many allied health curriculums at select universities. Perfusionists are required to pass a board examination, and many government organizations are now enacting licensure legislation. These persons must be able to adapt to the ever-changing technology present in cardiac surgery as well as to protocols and circuitry that vary among surgeons and institutions.
FUTURE DIRECTIONS
There are many commercial designs currently available that incorporate several of these components into a single disposable unit. Some units, for example, combine a reservoir with a membrane oxygenator and a heat exchanger. Continued innovation has resulted in more efficient, compact designs that limit the blood contacting surface area and decrease the need for large priming volumes and thus the need for patient transfusions. Improving biocompatibility of materials has lessened the inflammatory response that is associated with extracorporeal (outside the body) circulation. Sophisticated cannula designs are less traumatic to the vessels and have improved flow dynamics, causing less sheer stress on circulating red blood cells. New safety mechanisms such as alarm systems, pop-off valves, and automatic air evacuation devices will further increase the efficacy of these lifesaving machines.
The field of cardiac surgery is similarly evolving. Minimally invasive approaches to many heart operations are developing driven by patient demand as well as efforts to reduce postoperative hospital stay and patient morbidity. Recent debate has also focused on the possible adverse neurologic sequelae associated with the use of CPB. A growing number of coronary bypass procedures are now performed without the use of a heart–lung machine. Some centers have demonstrated success with this approach, decreasing the need for postoperative blood transfusions and end-organ dysfunction. To date, however, there is no conclusive evidence that avoidance of the heart lung machine results in improved neurologic outcomes or patient survival.
The creation of the heart–lung machine was the ratelimiting step to the development of the field of cardiac surgery. The ability to stop the heart while preserving flow the remainder of the heart has given surgeons the ability to repair defects in the heart and great vessels in patients of all ages. The pioneers in this field demonstrated remarkable courage and conviction in persevering in the face of overwhelming odds. Their collaborative efforts during one of the most prolific periods in the history of medicine have had a remarkable impact on human health. The future of cardiac surgery is largely dependent on continued advances in perfusion techniques and in the components of the heart–lung machine. In this endeavor, industry plays a pivotal role in developing and manufacturing improved products tailored to meet the needs of an everchanging field. Ultimately, evidence-based outcomes research will
help ensure these innovations result in improved outcome for patients.
BIBLIOGRAPHY
Cited References
1.Lewis FJ, Taufic M. Closure of atrial septal defects with the aid of hypothermia; experimental accomplishments and the report of one successful case. Surgery 1953;33:52–59.
2.Gibbon JH Jr. Artificial maintainance of circulation during experimental occlusion of the pulmonary artery. Arch Surg 1937;34:1105–1131.
3.Gibbon JH Jr., et al. the closure of interventricular septal defects in dogs during open cardiotomy with the maintainance of the cardiorespiratory functions by a pump oxygenator. J Thor Surg 1954;28:235–240.
4.Dennis C, et al. Development of a pump oxygenator to replace the heart and lungs: an apparatus applicable to human patients and application to one case. Ann Surg 1951;134: 709–721.
5.Kirklin JW, et al. Intracardiac surgery with the aid of a mechanical pump oxygenator system (Gibbon type): Report of eight cases. Proc Mayo Clin 1955;30:201–206.
6.Kay EB, et al. Certain clinical aspects in the use of the pump oxygentor. JAMA 1956;162:639–641.
7.Cross FS, et al. Description and evaluation of a rotating disc type reservoir oxygenator. Surg Forum 1956;7:274–278.
8.Dennis C, Karleson KE, Nelson WP, Eddy FD, Sanderson D. Pump-oxygenator to supplant the heart and lunf for brief periods. Surgery 1951;29:697–713.
9.Lillehei CW, et al. Direct vision intracardiac surgery in man using a simple, disposable artificial oxygenator. Dis Chest 1956;29:1–8.
10.Gott VL, et al. A self-contained, disposable oxygenator of plastic sheet for intracardiac surgery. Thorax 1957;12:1–9.
11.Kolff WJ, et al. Disposable membrane oxygenator (heart-lung machine) and its use in experimental surgery. Clev Clin Q 1956;23:69–97.
12.Lande AJ, et al. A new membrane oxygenator-dialyzer. Surg Clin North Am 1967;47:1461–1470.
13.Gravlee GP, Davis RF, Kurusz M, Utley JR, editors. Cardiopulmonary Bypass; Principles and Practice, Philadelphia: Lippincott Williams and Wilkins; 2000.
Reading List
Edmunds LH. Cardiopulmonary bypass after 50 years. New Engl J Med 2004;351:1603–1606.
Iwahashi H, Yuri K, Nose´ Y. Development of the oxygenator: Past, present and future. J Artif Organs 2004;7:111–120.
See also BLOOD GAS MEASUREMENTS; PULMONARY PHYSIOLOGY; RESPIRATORY MECHANICS AND GAS EXCHANGE.
HEAT AND COLD, THERAPEUTIC
MARY DYSON
United Kingdom
INTRODUCTION
Therapeutic levels of heating accelerate the resolution of inflammation, relieve pain, and promote tissue repair and
regeneration. Therapeutic levels of cooling reduce inflammation, relieve pain, and can reduce the damage caused by some injurious agents. To use therapeutic heating (thermotherapy) and therapeutic cooling (cryotherapy) effectively, the clinician should know:
The physiological effects of heat and cold
What devices are available to produce temperature changes
How they work
When and when not to use them
Their advantages and disadvantages.
This information assists the clinician in selecting the most suitable therapy and device for each patient.
The aim of this article is to provide this information, following a brief description of the history of these therapies, (this being included because history informs current and future usage).
HISTORY OF THERMOTHERAPY AND CRYOTHERAPY
Heat and cold have been used to treat diseases, aid healing, and reduce pain for many millennia. Exposure to the warmth of sunlight and thermal mineral springs continues to be used to this day. The ancient Greeks and Romans also advocated heated sand and oils for the treatment of injuries (1). Heated aromatic oils were rubbed on the body before massage, a form of therapy still in use today. In contrast, lesions accompanied by burning sensations (e.g., abscesses) were commonly treated by the application of cooling substances such as cold water and ice packs, another form of therapy still in use today. Fever was treated with cold drinks and baths; eating snow alleviated heartburn.
Thermotherapy
In the seventeenth century, one treatment for arthritis and obesity was to bury patients up to their necks in the sunwarmed sand of beaches. Warm poultices made from vegetable products such as leaves and cereals were used to treat musculoskeletal and dermal ailments. Molten wax was used to treat bruises around the eyes and infected eyelids, a technique not recommended because of the danger of getting hot wax into the orbit and damaging the eye.
In the eighteenth century, hot air produced by ovens and furnaces was used to induce perspiration and improve the circulation (2).
In the nineteenth century, Guynot found that wounds healed faster when the ambient temperature was 30 8C than when the ambient temperature was lower than this. After the invention of electric light bulbs, these were used to produce light baths and heating cabinets that were used to treat neuralgia, arthritis, and other conditions. Different wavelengths of light were also produced, initially by the use of prisms and passing light through media of different colors, and most recently by the use of lasers. There is evidence that different wavelengths of the electromagnetic spectrum including light and infrared radiation have
HEAT AND COLD, THERAPEUTIC |
463 |
different biomedical effects (3). When light is used to produce heat, both phototherapeutic and thermotherapeutic effects are produced that may reinforce each other.
In the late nineteenth and early twentieth century, the availability of electricity as a power supply led to the development of a host of novel thermotherapeutic devices including whirlpool baths, paraffin baths, and diathermy machines. In diathermy, high frequency electric currents are used to heat deeply located muscles. This effect was discovered in the 1890s when d’Arsonval passed a 1 A current at high frequency through himself and an assistant. He experienced a sensation of warmth (4). d’Arsonval worked on the medical application of high-frequency currents throughout the 1890s, work that led to the design of a prototype medical device by Nagelschmidt in 1906 and the coining of the term ‘‘diathermy’’ for what had previously been known as ‘‘darsonvalization.’’ The first diathermy machines were long wave, and in the second decade of the twentieth century, these were used to treat a wide range of diseases, including arthritis, poliomyelitis, and unspecified pelvic disorders. In 1928, short-wave diathermy was invented, superceding long-wave diathermy in Europe after the Second World War.
Therapeutic ultrasound also became popular in the post-war period, initially as a method of heating tissues deep within the body to relieve pain and assist in tissue repair. It was demonstrated experimentally that ultrasonic heating was accompanied by primarily nonthermal events such as micromassage, acoustic streaming, and stable cavitation. These events, which also occur at intensities less than are required to produce physiologically significant heating of soft tissues, produce cellular events that accelerate the healing process (3,5). Therapeutic ultrasound is generally used at frequencies in the 0.75–3 MHz range. The higher the frequency, the shorter the wavelength and the lower the intensity needed to produce physiologically significant heating of soft tissues. However, higher frequencies, because they are more readily absorbed than lower frequencies, are less penetrative and are therefore less suitable for heating deep soft tissues. Since the 1990s, low kilohertz ultrasound, also known as long-wave ultrasound, has been used to initiate and stimulate the healing of chronic wounds (6). Although long-wave ultrasound is not primarily a heating modality (7), as with all therapies in which energy is absorbed by the body, it is inevitably transduced into heat, although in this instance insufficient to be clinically significant (8).
Cryotherapy
Until the invention in 1755 of artificial snow, which was made by placing a container of water over nitrous ether as the latter vaporized, only cold water and naturally occurring ice and snow were available as means of cooling the body. In the nineteenth century, ice packs were used over the abdomen to reduce the pain of appendicitis and over the thorax to reduce the pain of angina. Ice was also used as a local anesthetic. In 1850, evaporative cooling methods were introduced; for example, ether applied to the warm forehead had a cooling effect as it evaporated. Since the early days of physical therapy, the local application of ice has
464 HEAT AND COLD, THERAPEUTIC
been used to treat acute musculoskeletal injuries; usually a combination of rest, ice, compression, and elevation (RICE) are recommended.
Contrast Bath Hydrotherapy
Hydrotherapy is the use of water as a therapeutic agent. Hot or cold water, usually administered externally, has been used for many centuries to treat a wide range of conditions, including stress, pain, and infection. Submersion in hot water is soothing and relaxing, whereas cold water may be anti-inflammatory and limit the extent of tissue damage. In contrast bath hydrotherapy, the patient is exposed to hot and cold water alternately to stimulate the circulation. The blood vessels dilate in the heat and constrict in the cold. Pain is also reduced. Contrast baths have been used to treat overuse injuries such as carpal tunnel syndrome and tendonitis of the hand and forearm (http://www.ithaca.edu/faculty/nquarrie/contrast.html). Although there has been little research on the efficacy of contrast baths, they remain in use and may be of clinical value (9).
THE PHYSIOLOGICAL EFFECTS OF HEAT AND COLD
When healthy we keep a fairly constant body temperature by means of a very efficient thermoregulatory system. We are homoeothermic organisms. Homoeothermic is a pattern of temperature regulation in which cyclic variation of the deep body (core) temperature is maintained within arbitrary limits of 2 8C despite much larger variations in ambient temperature. In health and at rest, our core temperature can be maintained by the thermoregulatory system within 0.3 8C of 37 8C in accordance with the body’s intrinsic diurnal temperature cycle. Superimposed on the diurnal temperature cycles are monthly and seasonal temperature cycles. Hyperthermia is a core temperature greater than 39 8C. Hypothermia is a core temperature less than
358C.
The physiological effects of heat and cold are generally
independent of the agent producing the temperature change, although in some cases, for example, ultrasound therapy, primarily nonthermally induced events accompany those induced thermally (3).
Physiological Effects of Heat
Heating of tissues occurs when these tissues absorb energy. The different effects of heating are due to many factors, including the following (10):
The volume of tissue absorbing the energy
The composition of the absorbing tissue
The capacity of the tissue to dissipate heat, a factor largely dependent on its blood supply
The rate of temperature rise
The amount by which the temperature is raised
Cell Metabolism. Cell metabolism increases by about 13% for each 1 8C increase in temperature up to the temperature at which the proteins of the cell, many of which
are vital enzymes, coagulate. Enzyme activity first increases as the temperature increases, then peaks, then declines as the enzymes denature, and is finally abolished when the temperature reaches about 45 8C when heat kills the cells. Only temperature increases less than those producing enzyme denaturation are therapeutic. The cells’ membranes are particularly sensitive to heat, which increases the fluidity of their lipoproteinaceous components, producing changes in permeability (11). At sublethal temperatures, heat-shock proteins, which give some protection to cells reexposed to heat, accumulate in the cells.
Abnormal and normal cells are affected differently by mild hyperthermia ( 40 8C). Synthesis of deoxyribonucleic acid (DNA), ribonucleic acid (RNA), and proteins can all be inhibited by mild hyperthermia in abnormal cells, irreversibly damaging their membranes and killing the cells; this does not occur in normal cells subjected to mild hyperthermia (12). The technique can therefore be used to kill abnormal cells selectively.
Collagen. The extensibility of collagen is increased by thermotherapy. Raising the temperature of a highly collagenous structure such as a tendon increases the extent of elongation produced by stretch of a given intensity (13). Joint capsules are also highly collagenous, so thermotherapy decreases the resistance of a joint to movement. Cryotherapy, however, increases this resistance. Changes in resistance to movement are also due to changes in the viscosity of the synovial fluid.
Laboratory experiments suggest that heat should be applied to tendons with caution because in vitro exposure of excised tendons to temperatures in the range of 41–45 8C is accompanied by a reduction in their tensile strength (13). It is unlikely, however, that the stresses produced by passive stretch during physical therapy and normal exercise will reach the levels at which rupture occurs, although overvigorous exercise could be damaging, particularly in those who ignore protective pain.
Blood Flow. Thermotherapy causes blood vessels to widen (vasodilatation), increasing local blood flow and causing the skin to redden (erythema). Heat-induced vasodiltation has several causes, including the following:
The direct effect of heat on the smooth muscle cells of the arterioles and venules.
If there is local damage, then the damage-induced release of vasodilators such as bradykinin will cause further vasodiltation.
Bradykinin, histamine, and other chemical mediators released in response to injury and to heating increase capillary and venule permeability that, together with an increase in capillary hydrostatic pressure, can produce local swelling of the tissues (edema). The application of local heat immediately after injury should therefore be avoided (14).
Heating also induces changes in blood flow in subcutaneous tissues and organs. These changes depend on the amount of heating. First blood flow increases in these structures, but then it decreases if heating is sufficient for the core temperature to rise, as blood is diverted to the
skin where heat is lost from it to the external environment as part of the thermoregulatory process.
Different local heating techniques can have different effects on blood flow, due to differences in their depth of penetration. Methods producing superficial heating include infrared radiation and contact with a heated material; those producing deep heating include short-wave and microwave diathermy. Infrared (IR) radiation increases cutaneous blood flow (15) but not in the underlying skeletal muscle (16). In contrast, diathermy increases blood flow and temperature in both skin and skeletal muscle in humans (17), hyperemia being sustained for at least 20 min after the cessation of treatment, probably because of an increase in the metabolic rate of the heated tissues.
Neurological Effects. Therapeutic heat produces changes in muscle tone and pain relief.
Muscle Tone. Increased muscle tone can sometimes be reduced by the application of either superficial or deep heat. In 1990, Lehmann and de Lateur (18) showed that heating skin and skeletal muscle of the neck relieved muscle spasm secondary to underlying pathology. The Ia afferents of muscle spindles increase their firing rate on receipt of heat within the therapeutic range, as do tendon organs (19). Most secondary afferents decrease their firing rate (20). Collectively these neurological changes reduce muscle tone locally.
Pain Relief. People in pain generally consider heat to be beneficial, even on the intense pain experienced by patients with cancer (21).
Therapeutic heat produces vasodiltation and may therefore relieve pain related to ischemia. Pain related to muscle spasm may also be relieved by therapeutic heat; this reduces muscle spasm secondary to underlying pathology (18). Heat may also act as a counterirritant and relieve pain via the pain gate mechanism (22), in that the thermal sensations take precedence in the central nervous system over nociceptive sensations.
Increasing the temperature within the therapeutic range increases the velocity of nerve conduction. An increase in sensory conduction increases the secretion of pain-relieving endorphins.
Tissue Injury and Repair. The initial response of vascularised tissues to injury is acute inflammation. This is characterized by:
Heat
Redness
Swelling (edema)
Pain
Loss of function
During it a host of chemical mediators and growth factors are secreted; these collectively limit the extent of tissue damage and lead to healing. The application of therapeutic levels of heat can accelerate the resolution of acute inflammation leading to faster healing. Although uncomfortable, acute inflammation is not a disease but a
HEAT AND COLD, THERAPEUTIC |
465 |
vital component of tissue repair and regeneration. The pain associated with it is generally of a short duration and is of survival value in eliciting protective actions.
The management of acute trauma by thermotherapy and cryotherapy is based on a pathophysiological model often referred to as the secondary injury model (23). According to this model, secondary injury is the damage that occurs as a consequence of the primary injury in previously unaffected cells. The mechanisms initially hypothesized as producing this damage were classified as being either enzymatic or hypoxic (24). Since then, knowledge of the mechanisms involved in cell death from trauma has increased dramatically and a third mechanism is now postulated, namely the delayed death of primary injured cells. A review and update by Merrick in 2002 (25) attempts to reconcile the secondary injury model with current knowledge of pathophysiology. Secondary hypoxic injury has been reclassified as secondary ischemic injury, and specific mechanisms for ischemic injury have been identified. In addition to changes of vascular origin, there is now evidence that apparently secondary injury may be due, in part, to the delayed death of cells subjected, for example, to mitochondrial damage during the primary trauma. A better understanding of secondary injury should inform methods of treatment, some of which, although traditional, may need to be altered to improve their effectiveness. For example, the rationale that shortterm cryotherapy of acute injuries was effective because it limited edema through vasoconstriction has been replaced by the currently accepted theory that it also retards secondary injury, regardless of the cellular mechanisms by which this occurs, be they lysosomal mechanisms, protein denaturation mechanisms, membrane permeability mechanisms, mitochondrial mechanisms, and/or apoptotic mechanisms (25). By reducing metabolic demand, the application of cryotherapy as soon as possible after an acute injury should reduce the speed and, possibly the extent, of secondary injury. There is evidence that continuous cryotherapy for 5 hours after a crush injury inhibited the loss of mitochondrial oxidative function that follows such injuries (25). The effect of continuous and intermittent cryotherapy for other durations on this and other pathophysiological events remains to be examined. This must be done if clinical treatments are to be improved.
Systemic and local therapeutic heating can reduce postoperative wound infection (26). The use of a warm-up dressing (Augustine Medical), which radiates heat and provides a moist wound environment, eradicated methi- cillin-resistant Staphylococcus aureus (MRSA) infection from pressure ulcers within 2 weeks (27). It should be appreciated that patients with MRSA-infected pressure ulcers, also known as pressure sores and bed sores, have increased morbidity and mortality; these ulcers can kill. Warming the tissues induces vasodiltation, giving rise to the high oxygen tension required for the production of oxygen-free radicals; these are an important part of the body’s defense against bacterial infection (28) and initiate collagen synthesis and re-epithelialization. Vasodiltation also aids healing by increasing the efficiency with which the cells and growth factors needed for this are transported to the injured region and the efficiency with which waste products are removed from it.
466 HEAT AND COLD, THERAPEUTIC
Warming has been shown to increase the proliferation of fibroblasts (29), the cells that synthesize collagen, and of endothelial cells (30), the cells lining blood vessels, in vitro. If this also occurs in vivo, then warming will accelerate the formation of granulation tissue. More research is required to confirm whether therapeutic heating reduces the risk of wound infection and accelerates healing in a clinically significant fashion. The data gathered to date suggest that it may. If so, then ‘‘there is a real prospect of reducing complications, improving patient outcomes, shortening hospital stays and minimizing costs’’ (31).
Physiological Effects of Cold
The physiological effects of therapeutic cold (9) depend on a range of factors, including
The volume of tissue to which cooling is applied
The composition of the tissues cooled
The capacity of the tissues to moderate the effects of cooling
The rate of temperature fall
The amount by which the temperature of the tissues is lowered
Cell Metabolism. The vital chemical processes occurring in cells generally slow as the temperature is lowered. These processes are catalyzed by enzymes, many of which are associated with the cells’ membranes. Cell viability relies on passive and active membrane transport systems, the latter involving ionic pumps activated by enzymes. These transport systems are necessary to maintain the intracellular ionic composition required for cell viability. Below a threshold temperature, the pumps fail, and consequently, the membranes lose their selective permeability; the intracellular concentration of Naþ and Ca2þ increases whereas that of K+ decreases. Between normal body temperature and this threshold, cooling is therapeutic. The application of therapeutic levels of cold can reduce cell degeneration and therefore limit the extent of tissue damage (31). The induction of mild hypothermia in the brain of a baby starved of oxygen at birth can interrupt the cascade of chemical processes that cause the neurons of the brain to die after being deprived of oxygen (32), reducing disability.
Collagen. Collagen becomes stiffer when cooled. People with rheumatoid arthritis generally experience a loss of mobility of their affected joints at low temperatures, due in part to increased stiffness of the collagen of their joint capsules (9).
Blood Flow. Lowering the skin temperature is detected by dermal thermoreceptors that initiate an autonomic reflex narrowing of the blood vessels of the skin (vasoconstriction). This results in reduction of the flow of blood to the dermis. Cold also has a direct constrictor effect on the smooth muscle of the blood vessels, and the arteriovenous anastomoses that shunt blood to the skin close. The resulting reduction in the flow of blood to the dermis diminishes heat loss through it. Countercurrent heat exchange between adjacent arteries and veins reduces heat loss.
These processes collectively reduce the rate at which the core temperature of the body falls.
Dermal vasoconstriction induced by lowering the temperature of the skin to approximately 10 8C is followed after a few minutes by cold-induced vasodiltation (CIVD) followed by cycles of alternating vasoconstriction and vasodiltation, resulting in alternating decrease and increase on dermal blood flow. Originally thought to be either a local neurogenic axon reflex or due to the local release of vasodilator materials into the tissues, CIVD is now believed to be due to paralysis of vascular smooth muscle contraction in direct response to cold (33). This reaction may provide some protection to the skin from damage caused by prolonged cooling and ischemia. However, in CIVD of the skin, the erythema produced is a brighter red than in erythema produced by heating because at low temperatures, the bright red oxyhemoglobin dissociates less readily than at higher temperatures. Therefore, although in CIVD the skin receives oxygen-rich blood, it is still starved of oxygen, suggesting that cryotherapy may not aid wound healing (9).
In contrast to skin, the blood flow in the skeletal muscles is determined more by local muscle metabolic rate than by temperature changes at the skin because muscles are insulated from these by subcutaneous fat.
Neurological Effects. Cold can be used therapeutically to affect muscle tone and pain.
Muscle Tone. Although cooling generally decreases muscle tone, this can be preceded by a temporary increase in tone (34), possibly related to tactile stimulation accompanying the application of cryotherapy by means of ice massage. Decrease in muscle tone in response to cryotherapy is likely to be due to a decrease in muscle spindle sensitivity as the temperature falls, together with slowing of conduction in motor nerves and skeletal muscle fibers.
Pain Relief. The immediate response of the skin to cold is a stimulation of the sensations of cold and pain. However, if the cold is sufficiently intense, nerve conduction is inhibited, causing both sensations to be suppressed. Less intense cold can be used as a counterirritant, its effects being explicably by the pain gate theory of Wall and Melzack (35). Enkephalins and endorphins may also be involved (36).
Tissue Injury and Repair. Cryotherapy has beneficial effects on the acute inflammatory phase of healing in that it reduces bleeding, decreases edema at the injury site, gives pain relief, and reduces local muscle spasm, as described above. Once these events have occurred, it should be replaced by thermotherapy because, as previously described, this accelerates the resolution of acute inflammation leading to faster healing.
DEVICES AVAILABLE FOR PRODUCING TEMPERATURE CHANGE
Thermotherapy Devices
These can be classified into those producing superficial heating and those producing deep heating. All should be

Figure 1. Hydrocollator pack for moist heating by conduction. Each pack consists of silica gel covered by cotton fabric. After submersion in hot water, the pack is applied to the area to be heated over layers of terry cloth.
used with caution in patients unable to detect heat-induced pain or over tissues with a poor blood supply because these cannot dissipate heat efficiently.
Superficial Heating. This is provided by devices that transfer energy to the body by conduction, convection, or radiation (37).
Conduction. Devices that heat by conduction include hydrocollator packs, hot water bottles, electric heating pads, and baths of heated paraffin wax.
Hydrocollator packs Figs. 1 and 2 consist of cotton fabric bags containing a silica gel paste that absorbs water equal to 10 times its weight. They are placed in thermostatically controlled tanks of hot water, and after they have absorbed this, one or more packs are placed on toweling-covered skin, into which heat is conducted until the pack has cooled, usually or 20–30 min, depending on the ambient temperature. Heat is also conducted into the subcutaneous tissues to a depth of about 5 mm. The transportation of blood warmed in the superficial tissues to deeper tissues may result in the latter also becoming warmer. Hot water bottles and thermostatically controlled electric heating pads act in a similar fashion.
Figure 2. Hydrocollator packs being heated in a thermostatically controlled tank containing hot water.
HEAT AND COLD, THERAPEUTIC |
467 |
When and When Not to Use These Devices. They are useful in the relief of pain and muscle spasm. Abdominal cramps can be treated effectively through the induction of smooth muscle relaxation. Superficial thrombophlebitis and localized skin infections such as boils or furuncles may also be treated by heat conduction with these devices. It has been advised that these and other primarily heating devices should not be used over injured tissue within 36 h of the injury. Nor should they be used where there is decreased circulation, decreased sensation, deep vein thrombophlebitis, impaired cognitive function, malignant tumors, a tendency toward hemorrhage or swelling, an allergic rash, an open cut, skin infection, or a skin graft (http://www.colonialpt.com/CPTFree.pdf). A search of Eng- lish–language textbook and peer–reviewed sources and computerized databases from January 1992 to July 2002 (38) has revealed ‘‘generally good agreement among contraindication sources for superficial heating devices’’; however, agreement ranged from 11% to 95% and was lower for pregnancy, metal implants, edema, skin integrity, and cognitive/communicative concerns.
Advantages and Disadvantages. The moist heat produced by the hydrocollator packs has a greater sedative effect than the dry heat produced by the other conduction devices listed above. The hydrocollator packs are, however, heavy because of the amount of water they absorb and should not be applied to very frail patients, for example those with advanced osteoporosis. Hot water bottles occasionally leak, producing scalding and should therefore be inspected after filling and before each use. Electric pads should be thermostatically controlled and used with an automatic timer to reduce the risk of burning the skin.
Paraffin Wax Baths. Molten paraffin wax is mixed with liquid paraffin wax and kept molten in a thermostatically controlled bath (Fig. 3) at between 51.7 and 59.9 8C. Typically used for heating hands or feet, these are either dipped into the bath several times so that a thick layer of wax forms on them, or immersed in the bath for 20–30 min. After dipping, the hand or foot is wrapped in a cotton towel to retain the heat; after 20–30 min, the towel and wax are peeled off.
When and When Not to Use These Devices. Paraffin wax baths are suitable for treating small areas with irregular surfaces. The heat provides temporary relief of joint stiffness and pain in patients with chronic osteoarthritis and rheumatoid arthritis. By increasing the elasticity of collagen, it helps to increase soft-tissue mobilization in the early stages of Dupuytren’s contracture and after traumatic hand and foot injuries provided that the lesions have closed. Paraffin wax baths should not be used to open or infected wounds.
Advantages and Disadvantages. Molten paraffin wax is suitable for applying heat to small irregular surfaces such as those of the hands and feet. The baths can be used several times without changing the wax, and several patients can be treated at the same time if the baths are sufficiently large.

468 HEAT AND COLD, THERAPEUTIC
Figure 3. Paraffin wax bath used for heating hands and feet by the dip method.
A disadvantage is that the wax collects debris from the surface of the skin and other particles that settle at the bottom of the bath and are difficult to remove without emptying the bath. Treatments must be supervised and the temperature of the wax monitored to avoid the risk of burning.
Convection. This involves immersion of either the whole of the body or part of the body in heated water. Hubbard tanks (Fig. 4), hydrotherapy pools (Fig. 5), akin
Figure 4. Hubbard tank used for partial or total body submersion.
to heated swimming pools, and baths can be used for either total or partial immersion. The Hubbard tanks can be fitted with agitation devices. Some baths incorporate low-frequency ultrasound transducers. It is recommended that the water temperature should not exceed 40.6 8C when used for total immersion and 46.1 8C when used for partial immersion (1). Treatments typically last for 20–30 min.
Hydrotherapy is useful for treating multiple arthritic joints simultaneously and for treating extensive soft-tissue injuries. Although total immersion can raise the core body temperature, the main use of hydrotherapy is for the relief of pain, muscle spasm, and joint stiffness. Exercise regimes can be incorporated into hydrotherapy treatment to take advantage of the increase in joint movement made possible by the decrease in joint stiffness and the pain associated with movement. The addition of an agitation device to a Hubbard tank allows it to be used for the gentle debridement of burns, pressure ulcers, and other skin conditions, provided that thorough cleaning and disinfection of the tank are carried out between treatments. Baths incorporating low frequency (kHz) ultrasound transducers have the additional advantage that they can stimulate the repair process as well as assist in debridement.
The greater the surface of the body that is immersed, the greater the number of joints and the greater the area of skin and subcutaneous tissue that can be heated. Immersion also provides buoyancy, helping the patient to exercise more easily.
Disadvantages are that
Each patient needs one-to-one supervision to ensure that the mouth and nose are kept free from water.
The equipment is relatively expensive and is timeconsuming to maintain.
Radiation. Heat transmission by radiation is generally provided by infrared lamps (Fig. 6). These are photon producers, emitting wavelengths from the red end of the visible part of the electromagnetic spectrum, together with IR. The production of visible light provides a visual indication when the lamps are active. The IR component provides heating.
Infrared emitters used for heating are either nonlaser or laser devices, the former being the most commonly used.
Nonlaser.
Luminous
Nonluminous
Luminous emitters are effectively light bulbs, each mounted in the center of a parabolic reflector that projects a bright beam like a floodlight. About 70% of the energy emitted consists of IR rays in the wavelength range of 700–4000 nm.
Luminous emitters can be classified into those with large, high-wattage (600–1500 W) bulbs, and those with smaller, lower wattage (250–500 W) bulbs.
1. The large luminous emitters are used to treat large regions of the body such as the lumbar spine. They

HEAT AND COLD, THERAPEUTIC |
469 |
are positioned about 0.6 m from the patient and used for 20 min.
2. The small luminous emitters are used to treat smaller regions of the body such as the hands and feet.
Figure 6. Luminous generator infrared lamp used for superficial heating by radiation. The height and angle of the lamp can be adjusted.
Figure 5. Hydrotherapy pool used for partial or total body submersion.
They are positioned closer to the patient, about 0.45–0.5 m away, and they are typically used for 20 min.
The former produce a greater sensation of warmth than the latter. The skin becomes temporarily erythematous, resembling blushing. If the heat is too great because, for example, the lamp is too close to the skin, erythema ab igne, a mottled appearance indicative of damage, may be produced.
Nonluminous emitters are usually cylinders of either metal or an insulating material, the radiation source, around which a resistance wire, the heat source, is coiled. They emit longer wavelengths of IR that penetrate deeper than the shorter IR wavelengths of the luminous emitters. About 90% of the emission of the nonluminous IR device is in the wavelength range of 3500–4000 nm.
When and When Not to Use These Devices. IR radiation when used as a heating modality can alleviate pain and muscle spasm. It also accelerates the resolution of acute inflammation. It should not be applied to skin that is photosensitive. It should only be applied to skin free from creams that, if heated, could burn the skin. Goggles should be worn to protect the eyes from radiation damage to either the lens or retina. Patients with abnormally low blood pressure should not receive any form of treatment that increases the temperature of large areas of skin and subcutaneous tissue because of the redistribution of blood to these areas and away from vital organs such as the brain, heart, and lungs.
Advantages and Disadvantages. IR heating is suitable for use in the home as well as in clinics and hospitals. Because the devices described in this section are noncontact, they can be used to treat open and infected wounds, but only for short periods because they cause fluid evaporation and wounds need to be kept moist if they are to heal efficiently.
470 HEAT AND COLD, THERAPEUTIC
The main disadvantage is that of burning if the IR heater is placed too close to the patient. Only mechanically stable devices should be used to ensure that they do not fall on the patient.
Laser. Lasers emitting IR energy have been used to treat injured tissue in Japan and Europe for over 25 years (39). The term ‘‘LASER’’ is an acronym for light amplification by the stimulated emission of radiation. Laser devices are a very efficient means of delivering energy of specific wavelengths to injured tissue. Laser radiation is coherent; that is the waves are in phase, their peaks and troughs coinciding in time and space. Some semiconductor diodes produce coherent and others noncoherent IR radiation. Both can accelerate repair and relieve pain, as does red light (39). The technique of using low intensity lasers therapeutically is usually referred to as LILT, an acronym for low intensity laser therapy, or low level laser therapy (LLLT) to distinguish it from the surgical use of high intensity lasers. Surgical CO2, ruby, and Nd-YAG surgical lasers can be used for thermal biostimulation provided that the amount of energy absorbed by the tissues is reduced sufficiently. Manufacturers achieve this either by defocusing and spreading the beam over an area large enough to reduce the power density below the threshold for burning or by scanning rapidly over the area to be treated with a narrow beam. The patient will then feel only a mild sensation of heat (39).
Nonsurgical lasers are typically used at power densities below those producing a sensation of heating; although their bioeffects are primarily nonthermal, absorption of light inevitably involves some heating. LILT can be used in either continuous or pulsed mode, the latter further reducing heating.
Single probes, each containing a single diode, are used to treat small areas of skin and small joints. Some are suitable for home use; their output is generally too low to produce the sensation of heat although the radiation they emit is transduced into heat after absorption. The effects of these devices on pain and tissue repair are essentially nonthermal. Clusters of diodes are used in clinics and hospitals to treat large areas of skin, skeletal muscle, and larger joints. These cluster probes generally include, in the interest of cost-effectiveness, nonlaser diodes. Treatment times vary with the type of probe and the area to be treated. Typically they range from about 3 to 10 min. They are calculated in terms of the time taken to deliver an effective amount of energy, generally 4 or more J cm 2, joules being calculated by multiplying the power density of the probe (in W cm 2) by the irradiation time in seconds. The larger cluster probes have sufficient output to produce a sensation of warmth. They also have essentially nonthermal effects that assist in the relief of pain and the stimulation of tissue repair (39).
When and When Not to Use These Devices. LILT devices are effective on acute and chronic soft-tissue injuries of patients of all ages. Most are designed for use in clinics and hospitals, but small, handheld devices are also available for home use as part of a first aid kit. The radiation they produce is absorbed by all living cells and transduced into chemical and thermal energy within these cells, which are
activated by it. Temporary diltation of superficial blood vessels occurs, aiding oxygenation.
Contraindications for LILT have been described in some detail by Tuner and Hode (39). They include the following:
As a matter of prudence, LILT should not be applied over the abdomen during pregnancy to ensure that no harm comes to the fetus although it can be applied to other parts of the body.
In patients with epilepsy who may be sensitive to
pulses of light in the 5–10 Hz range, it is advisable to use either unpulsed (continuous) LILT or much higher pulsing frequencies.
Treatment over the thyroid gland is contraindicated because this gland may be sensitive to absorbed electromagnetic radiation in the red and IR parts of the spectrum.
Patients with cancer or suspected cancer should only be treated by a cancer specialist.
Advantages and Disadvantages. LILT is easy to apply directly to the skin. The treatment is rapid and painless, the only sensations being of those of contact and, if the power is sufficiently high, of warmth. If used over an open wound, this should first be covered with a transparent dressing through which the radiation can pass unimpeded. The use of goggles is recommended as a precaution. The larger devices require clinical supervision, but some of the smaller predominantly nonthermal devices are suitable for home use.
Deep Heating. Deep heating devices produce heat within the tissues via electrostatic, electromagnetic, and acoustic fields. The term diathermy is used to describe the conversion or transduction of any form of energy into heat within the tissues. The devices used for deep heating include short-wave, microwave, and ultrasound generators. They are relatively expensive and should only be used by trained operators.
Short-Wave diathermy (SWD). SWD equipment Figs. 7 and 8 produces nonionizing radiation in the form of radio waves in the frequency range of 10–100 MHz. The most commonly used frequency is 27.12 MHz; this has a wavelength of 11 m.
SWD machines consist of:
An oscillating circuit that produces the high frequency current
A patient circuit connected to the oscillating circuit through which electrical energy is transferred to the patient
A power supply
The patient’s electrical impedance is a component of the patient circuit. Because the patient’s electrical impedance is variable, the patient circuit must be tuned to be in resonance with the oscillating circuit to ensure maximum flow of current through the patient.

Figure 7. SWD equipment. The SW energy leaving the insulated disk-shaped applicators produces small eddy currents in the tissues, thus heating them.
The most commonly used means of application of SWD are as follows:
The capacitor plate technique
The inductive method
The capacitor plate technique entails the placement of two capacitor plates near to the part of the patient’s body that is to be heated, with a spacer between each plate and
HEAT AND COLD, THERAPEUTIC |
471 |
the skin. The spacer allows the electric radiation to diverge just before entering, preventing thermal damage to the surface of the skin. The current density depends on the resistance of the tissues and on capacitance factors. Both subcutaneous fat and superficial skeletal muscle can be heated with this technique. The patient feels a sensation of warmth. Treatment is usually for 20 min day 1.
In the inductive method, a high-frequency alternating current is applied to a coiled cable generally incorporated into an insulated drum that is placed close to the part of the body requiring treatment. Alternatively, but these days rarely, an insulated cable is wrapped around the limb to be treated. The passing of an electric current through the cable sets up a magnetic field producing small eddy currents within the tissues, increasing tissue temperature. The patient feels a sensation of warmth. Treatment is usually for 20 min day 1.
Pulsed Shortwave. Some SWD devices allow the energy to be applied to the patient in short pulses. This form of application is termed pulsed short–wave diathermy (PSWD). The only physical difference between SWD and PSWD is that in the latter the electromagnetic field is interrupted at regular intervals. Pulsing reduces the amount of energy available for absorption by the tissues and therefore reduces the thermal load, allowing its nonthermal effects to be exploited without the risk of a potentially damaging thermal overload. It has been suggested that the applied energy produces ionic and molecular vibration affecting cellular metabolism (40).
With some PSWD machines, the therapist can vary the:
Pulse repetition rate (PRR)
Pulse duration (PD)
Peak pulse power (PPP).
The mean power applied is the product of these three variables.
Figure 8. Applicator heads for administration of SWD to produce deep heating of tissues.

472 HEAT AND COLD, THERAPEUTIC
Figure 9. Microwave diathermy equipment. The microwave generator contains a magnetron that produces high frequency alternating current. This current is carried by a coaxial cable shown on the left to a transducer (upper left) containing an antenna and a reflector. The antenna converts the electric current into microwaves that are collected by the reflector. This focuses them and beams them into the body, where they are transduced into heat.
Tissues with good conductivity, such as muscle and blood, i.e., with a high proportion of ions, should absorb energy preferentially from the SWD field. However, there is still debate about which tissues are heated the most during SWD and PSWD treatments.
Microwave Diathermy. Microwaves are that part of the electromagnetic spectrum within the frequency range of 300 MHz–300 GHz and, therefore, with wavelengths between 1 m and 1 mm. Microwave diathermy, although deeper than superficial (surface) heating, is not as deep as capacitative short-wave or ultrasonic heating (40).
Microwave generators (Fig. 9) contain a magnetron, which produces a high frequency alternating current that is carried to a transducer by a coaxial cable. The transducer consists of an antenna and a reflector. The electric current is transduced into electromagnetic energy on passing through the antenna. The reflector focuses this energy and beams it into the tissues to be heated (1).
On entering the body the microwave energy is absorbed, reflected, refracted, or transmitted according to the physical properties of the tissues in its path. When microwaves
are absorbed, their energy is transduced into heat. Tissues with a low water content (e.g., superficial adipose tissue) absorb little microwave energy, transmitting it into those with a high water content (e.g., skeletal muscle) that are very absorptive and therefore readily heated, warming adjacent tissues by conduction. As with SWD, there is no objective dosimetry, the intensity of treatment being judged by the patient’s sensation of warmth (41).
When and When Not to Use These Devices. As with other forms of heating, SWD and microwave diathermy are used to relieve pain and muscle spasm. They are also used to stimulate repair.
According to the Medical Devices Agency of the United Kingdom, there are several groups of patients on whom these devices must not be used:
1. Those with implanted drug infusion pumps because the energy provided during therapy may affect the pump’s electronic control mechanisms causing temporary alteration in drug delivery.
2. Women in the first trimester of pregnancy should not be treated with SWD because heating may be teratogenic.
3. Patients with metallic implants because metals are heated preferentially and may burn the surrounding tissue.
4. Patients fitted with active (i.e., powered) implants such as neurostimulators and cardiac pacemakers/ defibrillators because there have been reports of tissue damage, including nerve damage adjacent to stimulation electrodes on implanted lead systems.
5. Patients with pacemakers are also unsuitable for SWD because the frequency of the short-wave may interfere with cardiac pacing.
Patients with rheumatoid arthritis have had their joint pain reduced and walking time increased after treatment with microwave diathermy, and it has been suggested that the heat produced in the joints may have potentiated the effects of concurrent anti-inflammatory medication (42).
In the interests of safety, microwave diathermy should be restricted to patients in whom skin pain and temperature sensation are normal. It should not be used near the eyes, sinuses, and moist open wounds, all of which could be heated excessively because of their high water contact. Nor should it be used on any of the groups of patients in whom SWD is contraindicated.
Advantages and Disadvantages. The devices do not need to be in direct contact with the body; however, the body part being treated must be immobile during treatment because movement interferes with the field, resulting in too little heating in some regions and too much in other. Its disadvantages include its potential for burning tissue exposed to it due, for example, to treatment over regions in contact with metal. As with SWD, the therapist must be careful to avoid being exposed to the radiation, bearing in mind that some of the microwaves will be reflected from the patient.
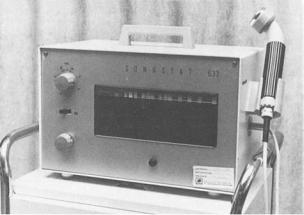
Figure 10. Portable ultrasound therapy equipment. The ultrasound transducer is housed in the head of the applicator shown on the right.
Ultrasound. Ultrasound therapy devices (Fig. 10 designed to produce clinically significant tissue heating operate at 0.75–3.0 MHz, the lower frequencies mechanical waves being more penetrative and affecting deeper tissues than the higher frequency waves. The ultrasound (US) is produced by the reverse piezoelectric effect when a high frequency alternating current is applied to a ceramic crystal causing it to vibrate at the same frequency. The crystal is located inside an applicator fitted with a metal cap into which these vibrations pass. A coupling medium such as either water or a gel with a high water content transmits the ultrasonic vibration into the tissues. US is transmitted readily by water and by tissue fluids. As US is more readily absorbed by protein than by fat, it can be used to heat muscle and collagenous tissue selectively without heating subcutaneous fat to a clinically significant level. Heating occurs where the US energy is absorbed. If the US enters the tissue at an angle other than 908, it is refracted; the transducer should therefore be held perpendicular to the surface being treated.
Absorption, and therefore heating, is greatest at higher frequencies. As a consequence, it is generally accepted that higher frequency US (e.g., 3 MHz) penetrates less deeply into the body than lower frequency US (e.g., 1 MHz). However, in some circumstances, 3 MHz may heat deeper tissues than originally theorized, when for example, it is transmitted into muscle over a bony prominence via a gel pad coated on both sides with an ultrasound transmitting gel (43). In this clinical investigation, it was found that 3 MHz ultrasound applied over the lateral malleolus of the ankle heated the musculotendinous tissue deep to the peroneal groove 0.5 cm deeper than suggested by others. The 3 MHz ultrasound produced heating deeper into muscle in healthy volunteers than did the 1 MHz ultrasound. This interesting but surprising observation may be an artifact due to variations in coupling of the US transducers to the skin. The authors point out that air trapped at the gel/pad interfaces might result not only in nonuniform heating but also in hot spots on the US transducer faceplate and possibly the skin.
HEAT AND COLD, THERAPEUTIC |
473 |
In recent years, US therapy devices producing kilohertz US have been developed (6); these are more penetrative than the MHz devices but produce little heat, using the nonthermal effects of US to produce their effects (7).
When US enters tissue, its intensity gradually decreases as energy is lost from the US beam by absorption, scattering, and reflection. This lessening of the force of the US is termed attenuation. US is scattered by structures smaller than its wavelength. It is reflected at the interfaces between materials with different acoustic impedances, e.g., air and skin, collagen and ground substance, periosteum, and bone. Muscle and bone are preferentially heated at their interfaces with other tissues, e.g., tendon and periosteum. This is because mode conversion occurs when US waves are reflected from these interfaces, the longitudinal incident waves being changed into reflected transverse waves, creating additional heating. Therapists can take advantage of this enhanced thermal effect to target inflamed joints. The thickness of tissue through which the US must pass for its intensity to be reduced to half the level applied to its surface is termed the half-value thickness. The half-value thickness of 1 MHz US is theoretically three times more than that of 3 MHz US although artifacts during clinical use may alter this (43).
The intensity range of US necessary to elevate tissue temperature to between 40 and 45 8C is 1.0–2.0 W cm 2 applied in continuous mode for 5–10 min (44). This increase is in the generally accepted thermal therapeutic range. Temperatures exceeding this can cause thermal necrosis and must be avoided. In poorly vascularized tissues, even the upper end of the therapeutic range can produce thermal damage. When US is absorbed or when mode conversion occurs, heat is generated in the tissues. This produces local increases in blood flow and softens collagenous deposits such as those in scars.
If the temperature increase is less than 1 8C, this is not considered to be clinically significant. Therapeutic effects produced by US used in a manner that produces a temperature increase of less than 1 8C are considered to be predominantly nonthermal. These effects, which include stable cavitation and acoustic streaming (3,44), occur at intensities lower than those necessary to produce therapeutic increases in temperature. The amount of acoustic energy entering the tissues can be reduced by pulsing the US. A commonly used pulsing sequence is 2 ms ON, 8 ms OFF. By reducing the total amount of energy supplied to the tissues, the thermal load on these tissues is reduced. The intensity during the pulse is sufficient to permit the predominantly nonthermal therapeutic events to occur. This is of particular value when ultrasound is used to treat tissue with a blood supply too poor to ensure adequate heat dissipation via the circulation.
The nonthermal therapeutic effects of US include stable cavitation and acoustic streaming. Cavitation is the production of bubbles of gas a few microns in diameter in fluid media such as tissue fluid and blood. The bubbles increase in size during the negative pressure or rarefaction part of the US wave and decrease during the positive pressure part. If the intensity is sufficiently great, the bubbles collapse, damaging the tissues. At lower intensities, within the therapeutic range, the
474 HEAT AND COLD, THERAPEUTIC
cavities are stable and acoustic streaming is increased around them. Acoustic streaming has been shown to cause reversible changes in membrane permeability to calcium and other ions, stimulating cell division, collagen synthesis, growth factor secretion, myofibroblast contraction, and other cellular events that collectively accelerate the resolution of acute inflammation leading to more rapid tissue repair (3).
When and When Not to Use These Devices. US therapy is used as a thermotherapeutic modality to treat acute and chronic injuries of the
Skin, e.g., pressure ulcers and venous ulcers
Musculoskeletal system, e.g., arthritis, bursitis, muscle spasms, and traumatic injuries to both soft tissue and bone.
In addition to superficial injuries, US can be used to heat tissues at a considerable depth from the surface of the skin. Sites of pathology such as damaged skeletal muscle, tendon, and ligaments within 5 cm of the surface can be heated preferentially; any adipose tissue superficial to these lesions is heated less because it absorbs less US than highly proteinaceous muscle, tendons, and ligaments. It is the most suitable form of thermotherapy to use on deeply located areas of damage in obese patients. At least 3 treatments per week are generally recommended, daily treatment being preferable. The treatment head should be moved throughout treatment to reduce the possibility of thermal tissue damage due to local hot spots and mechanical damage due to standing wave formation.
In the interests of safety, continuous US should only be used to heat tissues in patients sensitive to heat-induced pain. In patients lacking this sensitivity, pulsed ultrasound can be used. Although this may not heat the tissues significantly, nonthermal events will occur that can accelerate healing (3,44).
It should not be used either over tumors because it can increase cell division or over the eye because of the risk of collapse cavitation. Nor should it be used on any of the groups of patients in whom SWD is contraindicated.
Advantages and Disadvantages. Its differential absorption makes it the therapy of choice for treating muscle, tendons, ligaments, and bone without heating superficial adipose tissue.
The effects of ultrasound are, however, local and only small regions can be treated because of the small size of the treatment heads, rarely greater than 5 cm2. Another disadvantage is that a coupling medium such as water or a gel with a high water content must be used to transit the US from the applicator to the tissues. If there is an open wound, this must be covered with an ultrasound transmitting dressing such as Opsite to the surface of which the coupling medium can be applied. Alternatively the intact tissue adjacent to the wound and from which reparative tissue grows into the wound can be treated (3). Care must be taken to ensure that there are no bubbles in the coupling medium because reflection from these
can reduce the efficiency to energy transfer from the treatment head to the tissue. If the US energy is reflected back into the treatment head because of inadequate coupling, this will increase in temperature and could burn a patient, lacking adequate sensitivity to heat-induced pain.
Cryotherapy
Cryotherapy produces a rapid fall in the temperature of the skin and a slower fall in the temperature of the subcutaneous tissues, skeletal muscle, and the core temperature of the body. The rate of fall in skeletal muscle and the core temperature is dependent, in part, on the amount and distribution of adipose tissue. In a slender person with less than 1 cm of adipose tissue in the hypodermis, cooling extends almost 2.5 cm into the skeletal muscle after 10 min of applying cryotherapy to the skin surface. In an obese person with more than 2.5 cm of adipose tissue in the hypodermis, cooling extends only 1 cm into the muscle in the same time (1). To get deeper cooling in an obese person, the cooling agent must be applied for longer than in a slender person, for example, 30 min compared with 10 min to get adequate cooling extending 2.5 cm into the muscle. This concept has been confirmed recently by Otte et al. (45) who found that although 25 min of treatment may be adequate for a slender patient with a skinfold of 20 mm or less, 40 min is needed if the skinfold is between 21 and 30 mm, and 60 min if the skinfold is between 30 and 40 mm. The subcutaneous adipose tissue thickness is an important determinant of the time required for cooling in cryotherapy.
Cryotherapy is used to relieve pain, retard the progression of secondary injury, and hasten return to participation in sport and work. In a literature review by Hubbard et al. in 2004 (46), the conclusion drawn was that cryotherapy may have a positive effect on these aims, but attention was drawn to the relatively poor quality of the studies reviewed. There is a clear need for randomized, controlled clinical studies of the effect of cryotherapy on acute injury and return to participation in sport or work.
The main equipment used in cryotherapy is a refrigerator/freezer necessary for cooling gels and for producing ice. The ice is mixed with water, reducing the temperature of the water to just above its freezing point. The temperature of an injured limb can be reduced by immersing it in this ice/water mixture, an effective but initially uncomfortable experience for the patient. Alternatively, a cold compress containing the mixture can be applied to the region to be cooled. Also, a terry cloth can be soaked in the mixture, wrung out, and then applied. Blocks of ice can be used to massage an injured area if the skin over the injury is intact, an initially uncomfortable experience for both patient and therapist.
Another technique is to spray a vapor coolant on the skin. As the coolant evaporates, the temperature of the skin is reduced, but there is no clinically significant cooling of subcutaneous tissues. Ethylene chloride, chlorofluoromethane, or preferably a non-ozone-depleting vapor coolant, is sprayed over the area to be treated in a stroking fashion at a rate of about 1 cm s 1 (1). Concern over the ozone-depleting properties of chlorofluorocarbons has led
to the development of vapor coolant sprays that are not ozone-depleting (http://www.gebauerco.com/Default.asp) and that may be substituted for those noted above.
When and When Not to Use Cooling. The main use of cryotherapy is after acute skin and musculoskeletal injury to reduce swelling, bleeding, and pain. It helps give temporary relief to painful joints. It also reduces muscle spasms and spasticity, again temporarily. Trigger points, myofascial pain, and fibrositis may be treated with vapor coolant sprays.
Cooling is an excellent way of minimizing the effect of burns and scalds by reducing the temperature at the injury site provided that it is done as soon as possible after the incident. It can also slow brain damage after the deprival of oxygen in, for example, babies for whom delivery has been prolonged and difficult. A cool cap filled with chilled water is placed on the baby’s head within a few hours of birth and kept there for several days while the baby is in intensive care. A computerized controller circulates cold water through the cap, reducing the temperature of the brain by several degrees, minimizing cell death within the brain. The results of a clinical trial showed a significantly lower disability and death rate in children at risk of post-natal brain damage due to oxygen deprival if they were given this treatment than in those whose brains had not been cooled in this way (47). It has been suggested that a similar technique may help patients with hemorrhagic strokes where bleeding has occurred on to the surface of the brain.
Mild systemic hypothermia has also been reported to reduce brain damage after severe head trauma. Patients in whom the core temperature was reduced to and maintained at 33–35 8C with a cooling blanket for 4 days had reduced extradural pressure, an increase in the free radical scavenger superoxide dismutase, and consequently, less neuron loss and improved neurological outcomes (48).
Retarding secondary injury is an important benefit of cryotherapy. Secondary tissue death occurring after the initial trauma has been attributed to subsequent enzymatic injury and hypoxic injury (23). Cryotherapy reduces tissue temperature, slowing the rate of chemical reactions and therefore the demand for adenosine triphosphate (ATP), which in turn decreases the demand for oxygen, leading to longer tissue survival during hypoxia. By decreasing the amount of damaged and necrotic tissue, the time taken to heal may be reduced. In an extensive review of the secondary injury model and the role of cryotherapy, Merrick et al. (24) addressed the following question: ‘‘Is the efficacy of short-term cryotherapy explained by rescuing or delaying the death of the cells that were primarily injured but not initially destroyed?’’ He recommended the replacement of the term ‘‘secondary hypoxic injury’’ by ‘‘secondary ischemic injury’’ because hypoxia presents tissue with the challenge of reduced oxygen only, whereas ischemia presents inadequacies in not only oxygen but also fuel and anabolic substrates and in waste removal, all of which may contribute to secondary injury. Short-term cryotherapy may lessen the demand for these necessities.
Cooling should not be used:
In people who are hypersensitive to cold-induced pain
HEAT AND COLD, THERAPEUTIC |
475 |
Over infected open wounds
In people with a poor circulation
Advantages and Disadvantages. Disadvantages are that many people find prolonged exposure to cold therapy uncomfortable. Frostbite can occur if the skin freezes, as is possible if vapor coolant sprays are overused. Furthermore, its effects on chronic inflammatory conditions are generally temporary.
SUMMARY
Changing the temperature of tissues can reduce pain, muscle spasms, spasticity, stiffness, and inflammation. Heating the tissues by a few degrees centigrade increases tissue metabolism and accelerates the healing process. Cooling tissue by a few degrees centigrade can limit secondary damage to soft tissues, nerves, and the brain after trauma.
The correct selection of either thermotherapy or cryotherapy depends on an understanding of the physiological effects of heat and cold, and on knowledge of the patient’s medical condition. It is suggested that acute skin lesions and musculoskeletal injuries be treated:
First by the application of cold as soon as possible after the injury to limit its extent
Followed a few hours later by the application of moderate heat to accelerate the resolution of inflammation enabling healing to progress more rapidly.
It is recommended that the progress of healing be monitored noninvasively so that treatment can be matched to the response of the injury. In recent years this has become possible by means of high resolution diagnostic ultrasound or ultrasound biomicroscopy (49).
Having decided which is required, heat or cold, the next question is which modality to use. The following should be considered:
Size and location of the injury
Type of injury
Depth of penetration of the modality
Ease of application
Duration of application
Affordability
Medical condition of the patient
Contraindications
Patients heal their own injuries if they can to. This can be facilitated by the timely and correct application of therapeutic heat or cold. Their pain can also be relieved, improving their quality of life.
BIBLIOGRAPHY
Cited References
1.Tepperman PS, Kerosz V. In: Webster JG, ed., Encyclopedia of Medical Devices and Instrumentation. 1st ed. New York: John Wiley & Sons; 1988. p 14811–1493.
476HEAT AND COLD, THERAPEUTIC
2.Lehmann JF. Therapeutic Heat and Cold. 3rd ed. Baltimore: Williams & Wilkins; 1982.
3.Dyson M. Adjuvant therapies: ultrasound, laser therapy, electrical stimulation, hyperbaric oxygen and negative pressure therapy. In: Morison MJ, Ovington LG, Wilkie LK, editors. Chronic Wound Care: A Problem-Based Learning Approach. Edinburgh: Mosby; 2004. 129–159.
4.Guy AW, Chou CK, Neuhaus B. Average SAR and distribution in man exposed to 450 mMHz radiofrequency radiation. IEEE Trans Microw Theory Tech 1984;MTT–32:752–762.
5.Dyson M, Suckling J. Stimulation of tissue repair by ultrasound. A survey of the mechanisms involved. Physiotherapy 1978;64:105–108.
6.Peschen M, Weichenthal MM, Schopf E, Vanscheidt W. Lowfrequency ultrasound treatment of chronic venous ulcers in an outpatient therapy. Acta Dermatovenereol 1997;77:311–314.
7.Ward AR, Robertson VJ. Comparison of heating of nonliving soft tissue produced by 45 kHz and 1 MHz frequency ultrasound machines. J Org Sports Physiotherapists 1996;23:258– 266.
8.Kitchen S, Dyson M. Low-energy treatments: non-thermal or thermal? In:Kitchen S,editor. Electrotherapy. Evidence-Based Practice. Edinburgh: Churchill Livingstone; 2002. 107–112.
9.Stanton DB, Bear-Lehman J, Graziano M, Ryan C. Contrast baths: What do we know about their use? J Hand Ther 2003;16:343–346.
10.Kitchen S. Thermal effects. In: Kitchen S, editor. Electrotherapy. Evidence-Based Practice. Edinburgh: Churchill Livingstone; 2002. 89–105.
11.Bowler K. Cellular heat injury: Are membranes involved? In: Bowler K, Fuller BJ, editors. Temperature and animal cells. Cambridge: Company of Biologists; 1987. 157–185.
12.Westerhof W, Siddiqui AH, Cormane RH, Scholten A. Infrared hyperthermia and psoriasis. Arch Dermatol Res 1987;279:209–210.
13.Lehmann JF, Masock AJ, Warren CG, Koblanski JN. Effects of therapeutic temperatures on tendon extensibility. Arch Phys Med Rehab 1970;51:481–487.
14.Feebel H, Fast H. Deep heating of joints: A reconsideration. Arch Phys Med Rehab 1976;57:513–514.
15.Millard JB. Effects of high frequency currents and infrared rays on the circulation of the lower limb in man. Ann Phys Med 1961;6:45–65.
16.Wyper DJ, McNiven DR. Effects of some physiotherapeutic agents on skeletal muscle blood flow. Physiotherapy 1976;62: 83–85.
17.McMeeken JM, Bell C. Microwave irradiation of the human forearm and hand. Physiother Theory Practice 1990;6:171– 177.
18.Lehmann JF, de Lateur BJ. Therapeutic heat. In: Lehmann JF, editor. Therapeutic Heat and Cold. 4th ed. Baltimore: Williams & Watkins; 1990. 444.
19.Mense S. Effects of temperature on the discharges of muscle spindles and tendon organs. Pflug Arch 1978;374:159–166.
20.Lehmann JF, de Lateur BJ. Ultrasound, shortwave, microwave, laser, superficial heat and cold in the treatment of pain. In: Wall PD, Melzack R, editors. Textbook of Pain. 4th ed. New York: Churchill Livingstone; 1999. 1383–1397.
21.Barbour LA, McGuire DS, Kirchott KT. Nonanalgesic methods of pain control used by cancer outpatients. Oncol Nurs For 1986;13:56–60.
22.Doubell P, Mannon J, Woolf CJ. The dorsal horn: state dependent sensory processing, plasticity and the generation of pain. In: Wall PD, Melzack R, editors. Textbook of Pain. 4th ed. New York: Churchill Livingstone; 1999. 165–182.
23.Knight KL. Cryotherapy in Sports Injury Management. Champaign (IL): Human Kinetics; 1995.
24.Merrick MA, Rankin JM, Andrea FA, Hinman CL. A preliminary examination of cryotherapy and secondary injury in skeletal muscle. Med Sci Sports Exerc 1999;31:1516–1521.
25.Merrick MA. Secondary injury after musculoskeletal trauma: A review and update. J Athl Train 2002;37:209–217.
26.Melling DAC, Baqar A, Scott EM, Leaper DJ. Effects of preoperative warming on the incidence of wound infection after clean surgery: a randomized controlled trial. Lancet 2001;358:876–880.
27.Ellis SL, Finn P, Noone M, Leaper DJ. Eradication of methi- cillin-resistant Staphylococcus aureus from pressure sores using warming therapy. Surg Infect 2003;4:53–55.
28.MacFie CC, Melling AC, Leaper DJ. Effects of Warming on Healing. J Wound Care 2005;14:133–136.
29.Xia Z, Sato A, Hughes MA, Cherry GW. Stimulation of fibroblast growth in vitro by intermittent radiant warming. Wound Rep Regen 2000;8:138–144.
30.Hughes MA, Tang C, Cherry GW. Effect of intermittent radiant warming on proliferation of human dermal endothelial cells in vitro. J Wound Care 2003;12:135–137.
31.Zarro V. Mechanisms of inflammation and repair. In: Michlovitz SL, editor. Thermal Agents in Rehabilitation. Philadelphia: Davis; 1986. 3–17.
32.Gluckman PD, Wyatt JS, Azzopardi D, Ballard R, Edwards AD, Ferriero DM, Polin RA, Robertson CM, Thoresen M, Whitelaw A, Gunn AJ. Selective head cooling with mild systemic hypothermia after neonatal encephalopathy: multicentre randomized trial. Lancet 2005;365:632–634.
33.Keatinge WR. Survival in Cold Water. Oxford: Blackwell; 1978. 39–50.
34.Price R, Lehmann JF, Boswell-Bessett S, Burling S, de Lateur R. Influence of cyrotherapy on spasticity at the human ankle. Arch Phys Med Rehab 1993;74:300–304.
35.Wall PD, Melzack R, editors. Textbook of Pain. 4th ed. New York: Churchill Livingstone; 1999.
36.Fields HL, Basbaum AI. Central nervous system mechanisms of pain. In: Wall PD, Melzack R, editors. Textbook of Pain. 4th ed. New York: Churchill Livingstone; 1999. 309–330.
37.Tepperman PS, Devlin M. Therapeutic heat and cold. Postgrad Med 1983;73:69–76.
38.Batavia M. Contraindications for superficial heat and therapeutic ultrasound: Do sources agree? Arch Phys Med Rehabil 2004;85:1006–1012.
39.Tuner J, Hode L. The Laser Therapy Handbook. Grangesberg: Prima Books AB; 2004.
40.Scott S, McMeeken J, Stillman B. Diathermy. In: Kitchen S, editor. Electrotherapy. Evidence-Based Practice. Edinburgh: Churchill Livingstone; 2002. 145–170.
41.Low J. Dosage of some pulsed shortwave clinical trials. Physiotherapy 1995;81:611–616.
42.Weinberger A, Fadilah R, Lev A, et al. Treatment or articular effusions with local deep hyperthermia. Clin Rheumatol 1989;8:461–466.
43.Hayes BT, Merrick MA, Sandrey MA, Cordova ML. ThreeMHz ultrasound heats deeper into tissue than originally theorized. J Athl Train 2004;39:230–243.
44.Sussman C, Dyson M. Therapeutic and diagnostic ultrasound. In: Sussman C, Bates-Jensen BM, editors. Wound Care. A Collaborative Practice Manual for Physical Therapists and Nurses. 2nd ed. Gaithersburg: Aspen Publishers; 2001. 596–620.
45.Otte JW, Merick MA, Ingersoll CD, Cordova ML. Subcutaneous adipose tissue thickness alters cooling time during cryotherapy. Arch Phys Med Rehabil 2002;83:1501–1505.
46.Hubbard TJ, Aronson SL, Denegar CR. Does cryotherapy hasten return to participation? A systematic review. J Athl Train 2004;39:88–94.