
Astruc D. - Modern arene chemistry (2002)(en)
.pdf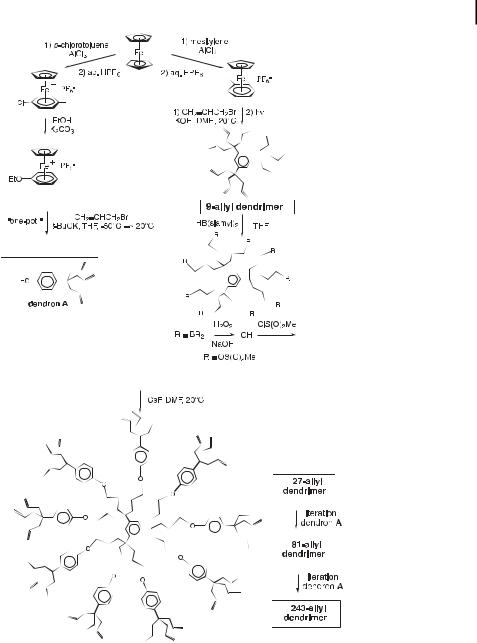
12.8 Convergent and Divergent Syntheses of Large Ferrocenyl Dendrimers with Good Redox Stabilities 423
Scheme 25. Divergent synthesis of polyallyl dendrimers.
decreased from good for the 9-Fc dendrimer to low for the 27-Fc dendrimer and to nil for the higher dendrimers, but solubility in diethyl ether remained good for all the ferrocenyl dendrimers. Likewise, the retention times on thin-layer or column chromatography increased with generation and no migration of the ‘‘243-Fc’’ dendrimer was observed.

424 12 Activation of Simple Arenes by the CpFeþ Group
Chart 1. Third-generation polyallyl dendrimer with |
was hydrosilylated using dimethylferrocenylsilane |
a theoretical number of 243 allyl branches syn- |
Fc(Me)2SiH to ‘‘243-Fc’’, a molecular battery |
thesized according to Scheme 20. This ‘‘243-allyl’’ |
dendrimer with a theoretical number of 243 |
dendrimer (like the 9-allyl, 27-allyl, and 81-allyl |
peripheral ferrocenyl units. |
dendrimers of generations 0, 1, and 2, respectively) |
|
This silane, reported by Pannel and Sharma [73], was later used by Jutzi [74] to synthesize the decaferrocenyl dendrimer [Fe(CCH2CH2SiMe2Fc)10] (Fc ¼ ferrocenyl) from decaallylferrocene.
Cyclic voltammetry of all the ferrocenyl dendrimers on a Pt anode showed all the ferrocenyl centers to be equivalent as only one wave was observed. It was possible to avoid adsorption even using CH2Cl2 for the small ferrocenyl dendrimers, but the use of MeCN was necessary for the medium-sized ones (27-Fc, 54-Fc, and 81-Fc). Finally, adsorption could not be avoided even with MeCN for the ‘‘243-Fc’’ dendrimer. From the intensity of the wave, the number of ferrocenyl units could be estimated using the Anson–Bard equation [75], and the numbers found were within 5 % of the branch numbers except in the case of the ‘‘243-Fc’’ dendrimer, for which the experimental number was too high (250) because of the adsorption.

12.8 Convergent and Divergent Syntheses of Large Ferrocenyl Dendrimers with Good Redox Stabilities 425
of ferrocenyl dendrimers |
243-allyl dendrimers. |
Divergent construction |
allyl, 27-allyl, 81-allyl and |
Scheme 26. |
from the 9- |

426 12 Activation of Simple Arenes by the CpFeþ Group
Scheme 27. Schematic strategy for the divergent construction of ferrocenyl dendrimers exemplified in Schemes 23.
12.9
Polyferrocenium Dendrimers: Molecular Batteries?
The first polyferrocenium dendrimers reported by our group in 1994 and characterized inter alia by Mo¨ssbauer spectroscopy (a ‘‘quantitative’’ technique) were mixed-valence FeII/FeIII complexes [41]. Since then, we have been seeking larger ferrocenyl dendrimers that could also withstand oxidation to their ferrocenium analogues. Syntheses of amidoferrocene dendrimers were simultaneously reported five years ago by our group [60, 61] and by the Madrid group using di erent cores [62]. In our reports, we were able to demonstrate the use of these metallodendrimers as redox sensors for the recognition of oxo anions, with remarkable positive dendritic e ects being seen when the generation was increased. The amidoferrocenyl dendrimers are not the best candidates for showing stable redox activity on a synthetic scale, however, and are even less well-suited for molecular batteries. Indeed, although they give fully reversible cyclic voltammetry waves, it is known that ferrocenium derivatives bearing an electron-withdrawing substituent are at best fragile, if stable at all. This inconvenience is probably exacerbated in the dendritic structures because of the steric constraints that force the ferrocenium groups to encounter one another more easily than as monomers. Thus, we oxidized our silylferrocenyl dendrimers using [NOþ][PF6 ] in CH2Cl2 and obtained stable polyferrocenium dendrimers as dark-blue precipitates, as expected from the known characteristic color of ferrocenium itself. These polyferrocenium dendrimers could be reduced back to soluble orange polyferrocenyl dendrimers using decamethylferrocene as the reductant [76]. No decomposition was observed in either the oxidation or the reduction reaction, and this redox cycle could be cleanly achieved in quantitative yield even with the 243-ferrocenyl dendrimer. The zero-field Mo¨ssbauer spectrum of the 243-ferrocenium dendrimer showed a single line corresponding to the expected spectrum known for ferrocenium itself [77], confirming its electronic structure. Thus, these polyferrocenyl dendrimers are stable electronreservoir systems. Indeed, despite their size, they transfer a very large number of electrons rapidly and ‘‘simultaneously’’ within the electrode. By ‘‘simultaneously’’, we mean that, vi-

12.9 Polyferrocenium Dendrimers: Molecular Batteries? 427
sually, the cyclic voltammogram looks as if it were that of a monoelectronic wave. One must question the notion of the isopotential for the many ferrocenyl units at the periphery of a dendrimer. In theory, all the standard potentials of the n ferrocenyl units of a single dendrimer are distinct, even if all of them are equivalent and independent. This situation arises since the charge of the overall dendrimer molecule increases by one unit every time one of its ferrocenyl units is oxidized to ferrocenium. The next single-electron oxidation is more di cult than the preceding one since, as the dendritic molecule has one more unit of positive charge, it is more di cult to oxidize because of the increased electrostatic factor. Thus, the potentials of the n redox units are statistically distributed around an average standard potential centered at the average potential (Gaussian distribution) [75]. In practice, the situation is complicated by the fact that the dendritic molecule, despite its large size, is rotating much more rapidly than the usual electrochemical time scales [78, 79]. Under these conditions, all the potentials are probably averaged. The fast rotation is also responsible for the fact that all the ferrocenyl units come close to the electrode within the electrochemical time scale. Consequently, there is no slowing down of the electron-transfer due to distance from the electrode, even in large dendrimers. Indeed, the waves of the ferrocenyl dendrimers always appear fully electrochemically reversible, indicating fast electron transfer.
The ferrocenyl dendrimers are also readily adsorbed on electrodes, a phenomenon already well known with various kinds of polymers [80]. When the polymers contain redox centers, the adsorbed systems have long been shown to give rise to a redox wave for which the cathodic and anodic waves are located at exactly the same potential, and for which the intensity of each wave is proportional to the scan rate. Continuous cycling shows the stability of the adsorption of the electrode modified in this way. As expected, the ferrocenyl dendrimers described here show this phenomenon. The stability of the modified electrode produced by soaking a Pt electrode in a solution in CH2Cl2 containing the ferrocenyl dendrimer and cyclic scanning between the ferrocenyl and ferrocenium regions is even better as the ferrocenyl dendrimer is larger. For instance, in the case of the 9-ferrocenyl dendrimer, scanning twenty times is necessary in order to obtain a constant intensity, and this intensity is weak. With the 27-, 54-, 81-, and 243-ferrocenyl dendrimers, only approximately ten cyclic scans are necessary in order to obtain a constant wave, and the intensity is much larger. When such derivatized electrodes are washed with CH2Cl2 and re-used with a fresh, dendrimer-free CH2Cl2 solution, the cyclic voltammogram obtained shows DEp ¼ 0. Other characteristic features are the linear relationship between the intensity and scan rate and the constant stability after cycling many times with no sign of diminished intensity.
Under these conditions, one may note that the argument of the fast rotation of the dendritic molecule being responsible for bringing all the redox centers in turn close to the electrode does not hold for modified electrodes. Some redox centers must be close to the electrode and some must be far away. It is probable that a hopping mechanism in the solid state is responsible for fast electron transfer and for averaging all the potentials of the different ferrocenyl groups of a single dendritic molecule around a mean value. The proximity of the ferrocenyl groups at the periphery of the dendrimer is a key factor in facilitating this hopping since it is known that electron transfer with redox sites that are remote or buried inside a molecular framework is slow, if observable at all [81–87].

42812 Activation of Simple Arenes by the CpFeþ Group
12.10
Large Dendrimers Functionalized on their Branches by the Electron-Reservoir [FeCp(h6-C6Me6)]B Groups: A Molecular Battery in Action
Ferrocenes and ferrocenyl dendrimers are poor reductants. On the other hand, the complexes [Fe(h5-C5R5)(h6-C6Me6)]2þ=þ=0 (R ¼ H or Me) have been shown to be e cient for various stoichiometric and catalytic electron-transfer reactions [26b]. Branching of this sandwich complex by means of a chlorocarbonyl substituent on the Cp ligand leads, upon reaction with dendritic polyamines, to soluble FeII metallodendrimers. Moreover, these FeII metallodendrimers can be reduced to FeI using [FeICp(h6-C6Me6)] (1). Reduction of the monomeric model [FeII(h5-C5H4CONHnPr)(h6-C6Me6)][PF6] with Na/Hg in THF at room temp. gives the deep-blue-green, thermally stable 19-electron complex [FeI(h5-
C5H4CONHnPr)(h6-C6Me6)] (Eq. (1)), which shows the classic rhombic distortion of the FeI sandwich family, as evidenced by EPR in frozen THF at 77 K (3 g values around 2) [8f ]. In view of this stability, we carried out the same reaction of [FeII(h5-C5H4COCl)(h6- C6Me6)][PF6] with the commercial polypropyleneimine dendrimer of generation five (64 amino termini) in MeCN/CH2Cl2 (2:1) in the presence of NEt3. The polycationic metallodendrimer DAB dendr-64-NHCOCpFeII(h6-C6Me6) was obtained as its PF6 salt, and was found to be soluble in MeCN and DMF (Eq. (2)). This dendritic complex was characterized by 1H and 13C NMR and IR spectroscopies, and by cyclic voltammetry (a single reversible wave in DMF at E1=2 ¼ 1:84 V vs. FeCp2 0=þ; DE ¼ 70 mV). Attempts to reduce it with the classic reductants that reduce monomeric complexes [FeII(h5-Cp)(h6-arene)][PF6], such as Na/sand, Na/Hg, or LiAlH4 in THF or DME, failed due to the insolubility of both the metallodendrimer and the reductant in the required solvents. The only successful reductant was the parent 19-electron complex [FeICp(h6-C6Me6)] (in pentane or THF), which reduced the metallodendrimer in MeCN at 30 C to the neutral, deep-green-blue 19-electron FeI dendrimer 6 in a few minutes (Eq. (3)). The exoergonicity of this electron-transfer reaction is 0.16 V; this is due to the electron-withdrawing e ect of the juxta-cyclic carbonyl group on the Cp ring, which lowers the reduction potential of the metallodendrimer as compared to that of [FeICp(h6-C6Me6)]. Although the FeI dendrimer decomposes at 0 C, it could also be characterized by its EPR spectrum at 10 K, which confirmed, as the deep-blue-green color, the FeI-sandwich structure analogous to that of the monomeric model. In contrast to the case of [FeICp(h6-C6Me6)], however, it was not possible to record the EPR spectrum of the solution of the FeI dendrimer above 10 K. This was presumably due to intramolecular relaxation among the peripheral FeI sandwich units. The intermolecular version of this relaxation e ect is known to preclude observation of the spectrum of monomeric FeI sandwich complexes in the solid state above 4 K and in solution above 77 K [24e]. This solution of the 64-FeI dendrimer in acetonitrile was used for the reaction with C60, the FeI/C60 stoichiometry being 1:1 (64 C60 per dendrimer). Upon reaction with a solution of C60 in toluene, the deep-blue-green color of the FeI dendrimer disappeared, leaving a yellow solution that contained [FeIICp(h6-C6Me6)][PF6] and a black precipitate (Eq. (4)). Tentative extraction of this precipitate with toluene yielded a colorless solution, which indicated that no C60 was present. The Mo¨ssbauer spectrum of this black solid at 298 K showed parameters indicative of the presence of an FeII sandwich complex of the same family as [FeIICp(h6-C6Me6)]þ [25a, 25c]. Its EPR spectrum recorded at 77 K was identical to that of [FeIICp(h6-C6Me6)]þ

12.11 Conclusion 429
C60 [88]. It could thus be concluded that C60 had been reduced to its monoanion, as befits a process that is exergonic by 0.9 V [89]. The [dendr-FeII]þ C60 units, being very large, must be located at the dendrimer periphery, presumably in rather tight ion pairs, although the number of fullerene layers and overall molecular size are unknown (Figure 1).
ð1Þ
ð2Þ
ð3Þ
ð4Þ
12.11
Conclusion
CpFeþ-activation of simple aromatics leads to clean, high-yielding benzylic perfunctionalization reactions. This family of reactions, which can be carried out on a large scale under ambient conditions with a variety of arene ligands, gives stars and dendritic cores and can be applied to the one-pot synthesis of the dendron p-HOC6H4C(allyl)3. These building blocks could be assembled to synthesize large polyallyl dendrimers and metallodendrimers containing various redox centers at the extremities of the branches. We have chosen ferrocenyl,

430 12 Activation of Simple Arenes by the CpFeþ Group
Fig. 1. Dendr-64-NHCOCpFe(C6Me6)64þ, 64 C60. resulting from the reaction of 6 with C60 in MeCN/toluene at 30 C, along with its EPR spectrum (bottom, right) in MeCN at 10 K and Mossbauer€ spectrum at 77 K (bottom, left).

References 431
cobaltocenyl, and [FeCp(h6-C6Me6)]þ redox centers to give stable redox systems. The resulting redox properties are redox recognition (sensors showing a dendritic e ect), redox catalysis (as e cient as using monometallic systems with the same driving force), and very e cient derivatization of electrodes (towards more practical sensors). Finally, we have succeeded in using a metallodendrimer containing 64 [FeICp(h6-C6Me6)] units at the branch termini in a synthetic electron-transfer reaction with C60 to give an outer shell of 64 ion pairs [FeþCp(h6-C6Me6)][C60. ] at the periphery of the dendrimer. This latter reaction shows that the metallodendrimer can behave as an electron reservoir, exemplifying the possibilities of a molecular battery operating at a given potential. Redox-stable metallocene dendrimers [90] o er a spectrum of possibilities [91, 92] in connection with electron-transfer processes in nanoscopic devices [93, 94] that will be further exploited in the future.
Acknowledgement
We are grateful to the students, collaborators, and colleagues cited in the references, in particular Jean-Claude Blais (MALDI-TOF mass spectrometry, University Paris VI) and Franc¸ois Varret (Mo¨ssbauer spectroscopy, University of Versailles) for their invaluable contributions to the ideas and experimental e orts that have led to the results presented herein. Financial support from the Institut Universitaire de France (DA), the CNRS, the Universities Bordeaux I and Paris VI, the Ministe`re de l’Enseignement et de la Recherche Scientifique, and the Re´gion Aquitaine is also gratefully acknowledged.
References
1 |
J.-M. Lehn, Supramolecular Chemistry: |
|
245; c) For a review on ferrocene den- |
|
Concepts and Perspectives, VCH, Weinheim, |
|
drimers, see: C. M. Casado, I. Cuadrado, |
|
1995. |
|
M. Mora´n, B. Alonso, J. Losada, Coord. |
2 |
M. Major, J.-M. Lehn, J. Am. Chem. Soc. |
|
Chem. Rev. 1999, 185–186, 53. |
|
1999, 121, 11231. |
10 |
M. A. Hearshaw, J. R. Moss, In Advances |
3 |
V. Balzani, F. Scandola, Supramolecular |
|
in Macromolecules (Ed.: G. Newkome), JAI |
|
Chemistry, Ellis Horwood, New York, 1991. |
|
Press Inc., Stamford, CT, 1999, Vol. 4, pp. |
4 |
V. Balzani (Ed.), Electron Transfer in |
|
1–60. |
|
Chemistry, Vol. II, Wiley-VCH, Weinheim, |
11 |
M. A. Hearshaw, J. R. Moss, Chem. |
|
2001. |
|
Commun. 1999, 1. |
5 |
N. Ardoin, D. Astruc, Bull. Soc. Chim. Fr. |
12 |
G. R. Newkome, C. N. Moorefield, F. |
|
1995, 132, 875. |
|
Vo¨gtle, Dendrimers and Dendrons. |
6 |
V. Balzani, S. Campana, G. Denti, A. |
|
Concepts, Synthesis, Applications, Wiley- |
|
Juris, S. Serroni, M. Venturi, Acc. |
|
VCH, Weinheim, 2001. |
|
Chem. Res. 1998, 31, 26. |
13 |
G. E. Oosterom, J. N. H. Reek, P. C. J. |
7 |
M. Venturi, S. Serroni, A. Juris, S. |
|
Kamer, P. W. N. M. van Leeuwen, Angew. |
|
Campana, V. Balzani, Top. Curr. Chem. |
|
Chem. Int. Ed. 2001, 40, 1828. |
|
1998, 197, 193. |
14 |
D. Astruc, F. Chardac, Chem. Rev. 2001, |
8 |
G. R. Newkome, E. He, C. N. Moore- |
|
101, 2991. |
|
field, Chem. Rev. 1999, 99, 1689. |
15 |
D. Astruc, Electron Transfer and Radical |
9 |
a) I. Cuadrado, M. Mora´n, C. M. Casado, |
|
Processes in Transition Metal Chemistry, |
|
B. Alonso, J. Losada, Coord. Chem. Rev. |
|
VCH, New York, 1995. |
|
1999, 189, 123; b) C. Casado, I. Cuadrado, |
16 |
a) D. Astruc, Topics Curr. Chem. (Ed.: W. |
|
M. Mora´n, B. Alonso, M. Barranco, J. |
|
A. Herrmann), Springer Verlag, Berlin, |
|
Losada, Appl. Organomet. Chem. 1999, 14, |
|
1991, 160, 47; b) D. Astruc, J.-C. Blais, |
432 |
12 Activation of Simple Arenes by the CpFeþ Group |
|
|
|
|
|
E. Cloutet, L. Djakovitch, S. Rigaut, J. |
|
Free Radicals in Biology (Ed.: W. A. Prior), |
|
|
Ruiz, V. Sartor, C. Vale´rio, Topics Curr. |
|
Academic Press, New York, 1976, p. 239. |
|
|
Chem., Dendrimers II (Ed.: F. Vo¨gtle), |
29 |
A. N. Nesmeyanov, N. A. Vol’kenau, I. N. |
|
|
Springer Verlag, Berlin, 2000, 210, 229. |
|
Bolesova, Dokl. Akad. Nauk SSSR 1967, |
17 |
D. Astruc, In The Chemistry of the Metal– |
|
175, 606. |
|
|
|
Carbon Bond (Ed.: F. R. Hartley), Vol. 4, |
30 |
C. C. Lee, A. S. Abd El Aziz, R. L. |
|
|
Wiley, New York, 1987, pp. 625–731. |
|
Chowdhurry, U. S. Gill, A. Piorko, |
18 |
D. Astruc, Tetrahedron Report N 157, |
|
R. G. Sutherland, J. Organomet. Chem. |
|
|
|
Tetrahedron 1983, 39, 4027. |
|
1986, 315, 79. |
19 |
L. Balas, D. Jhurry, L. Latxague, S. |
31 |
R. G. Sutherland, C. H. Zhang, A. |
|
|
|
Grelier, Y. Morel, M. Hamdani, N. |
|
Piorko, C. C. Lee, J. Org. Chem. 1989, 67, |
|
|
Ardoin, D. Astruc, Bull. Soc. Chim. Fr. |
|
137. |
|
|
1990, 127, 401. |
32 |
See refs. [67–69] and L. Djakovitch, |
20 |
A. N. Nesmeyanov, N. A. Vol’kenau, |
|
Ph.D. Thesis, University Bordeaux I, 1994. |
|
|
|
I. N. Bolesova, Tetrahedron Lett. 1963, |
33 |
For the synthesis of [FeIICp(h6- |
|
|
1725. |
|
C6Me6)][PF6], see refs. [25, 34, 35, 51c, |
21 |
T. P. Gill, K. R. Mann, Inorg. Chem. |
|
p. 792]. |
|
|
|
1980, 19, 3007. |
34 |
P. L. Pauson, W. E. Watts, J. Chem. Soc. |
22 |
F. Moulines, M. Kalam-Alami, V. |
|
1963, 2990. |
|
|
|
Martinez, D. Astruc, J. Organomet. |
35 |
D. Astruc, J.-R. Hamon, M. Lacoste, |
|
|
Chem. 2001, 125, 643–644. (special issue |
|
M.-H. Desbois, E. Roma´n, Organometallic |
|
|
dedicated to F. Mathey). |
|
Synthesis (Ed.: R. B. King), 1988, Vol. IV, |
23 |
A. N. Nesmeyanov, Adv. Organomet. Chem. |
|
p. 172. |
|
|
|
1972, 10, 1. |
36 |
J.-R. Hamon, J.-Y. Saillard, A. Le Beuze, |
24 |
a) R. E. Dessy, F. E. Stary, R. B. King, M. |
|
M. McGlinchey, D. Astruc, J. Am. |
|
|
|
Waldrop, J. Am. Chem. Soc. 1966, 88, 471; |
|
Chem. Soc. 1982, 104, 3755. |
|
|
b) A. N. Nesmeyanov, N. A. Vol’kenau, |
37 |
F. Moulines, D. Astruc, Angew. Chem. |
|
|
L. S. Shilovstseva, V. A. Petrakova, J. |
|
Int. Ed. Engl. 1988, 27, 1347. |
|
|
Organomet. Chem. 1973, 61, 329. |
38 |
F. Moulines, D. Astruc, J. Chem. Soc., |
25 |
a) J.-R. Hamon, D. Astruc, P. Michaud, |
|
Chem. Commun. 1989, 614. |
|
|
|
J. Am. Chem. Soc. 1981, 103, 758; b) |
39 |
J.-R. Hamon, P. Hamon, K. |
|
|
J.-R. Hamon, G. Althoff, E. Roma´n, P. |
|
Boukheddaden, J. Linare`s, F. Varret, |
|
|
Batail, P. Michaud, J.-P. Mariot, F. |
|
D. Astruc, Inorg. Chim. Acta 1995, 240, |
|
|
Varret, D. Astruc, D. Cozak, J. Am. |
|
105. |
|
|
Chem. Soc. 1979, 101, 5445; c) M. V. |
40 |
E. Alonso, D. Astruc, unpublished work. |
|
|
Rajasekharan, S. Giezynski, J. H. |
41 |
J.-L. Fillaut, J. Linares, D. Astruc, |
|
|
Ammeter, J.-R. Hamon, P. Michaud, |
|
Angew. Chem. Int. Ed. Engl. 1994, 33, 2460. |
|
|
D. Astruc, J. Am. Chem. Soc. 1982, 104, |
42 |
C. Vale´rio, B. Gloaguen, J.-L. Fillaut, |
|
|
2400; d) J. C. Green, M. R. Kelly, M. P. |
|
D. Astruc, Bull. Soc. Chim. Fr. 1996, 133, |
|
|
Payne, E. A. Seddon, D. Astruc, J.-R. |
|
101. |
|
|
Hamon, P. Michaud, Organometallics |
43 |
J.-L. Fillaut, R. Boese, D. Astruc, Synlett |
|
|
1983, 2, 211; e) D. Astruc, Acc. Chem. Res. |
|
1992, 55. |
|
|
1986, 19, 377. |
44 |
J.-L. Fillaut, D. Astruc, New J. Chem. |
26 |
a) J. Ruiz, F. Ogliaro, J.-Y. Saillard, J.-F. |
|
1996, 20, 945. |
|
|
|
Halet, F. Varret, D. Astruc, J. Am. |
45 |
H. W. Marx, F. Moulines, T. Wagner, |
|
|
Chem. Soc. 1998, 120, 11693; b) D. |
|
D. Astruc, Angew. Chem. Int. Ed. Engl. |
|
|
Astruc, Acc. Chem. Res. 2000, 33, 287. |
|
1996, 35, 1701. |
27 |
a) D. Astruc, E. Roma´n, J.-R. Hamon, P. |
46 |
J. Ruiz, E. Alonso, J. Guittard, J.-C. |
|
|
|
Batail, J. Am. Chem. Soc. 1979, 101, 2240; |
|
Blais, D. Astruc, J. Organomet. Chem. |
|
|
b) H. J. Trujillo, C. M. Casado, J. Ruiz, |
|
1999, 582/1, 139. |
|
|
D. Astruc, J. Am. Chem. Soc. 1999, 121, |
47 |
F. Moulines, L. Djakovitch, J.-L. |
|
|
5674. |
|
Fillaut, D. Astruc, Synlett 1992, 57. |
28 |
a) J.-R. Hamon, D. Astruc, J. Am. Chem. |
48 |
V. Marvaud, D. Astruc, Chem. Commun. |
|
|
|
Soc. 1983, 105, 5951; b) I. Fridovitch, |
|
1997, 773. |