
ppl_05_e2
.pdf
ID: 3658
Customer: Oleg Ostapenko E-mail: ostapenko2002@yahoo.com Customer: Oleg Ostapenko E-mail: ostapenko2002@yahoo.com
CHAPTER 7
WEIGHT
127

Order: 6026
Customer: Oleg Ostapenko E-mail: ostapenko2002@yahoo.com
Customer: Oleg Ostapenko E-mail: ostapenko2002@yahoo.com
CHAPTER 7: WEIGHT
128

ID: 3658
Customer: Oleg Ostapenko E-mail: ostapenko2002@yahoo.com Customer: Oleg Ostapenko E-mail: ostapenko2002@yahoo.com
CHAPTER 7: WEIGHT
WEIGHT.
Weight and Mass.
We will begin this chapter by differentiating between the concepts of mass and weight.
Because of the inexact way in which the word mass is used in everyday language, it is not uncommon for pilots to confuse the meanings of the terms mass and weight when they are used in a technical context such as in the study of aviation.
Even in aviation, though, confusion still exists. For many years, “Weight and Balance” was the title of a ground school subject in United Kingdom pilot training syllabuses.
Now, under the infuence of the JAA, the subject is called “Mass and Balance”. There is a case, however, for the subject to be called “Weight, Mass and Balance” because the weight of a body is different from the body’s mass. However, the subject’s title remains “Mass and Balance”.
Weight and
mass are two different
concepts.
Weight is a force. Mass is a quantity of matter.
In aircraft operations, the concept of mass is of vital importance when considering horizontal accelerations and decelerations, such as during take-off, landing and horizontal level turns; whereas, in airborne manoeuvres, where accelerations in planes other than the horizontal are concerned, weight as well as mass must be taken into account.
What is the difference between weight and mass?
Put simply, mass is a measure of the amount of matter in a body. Mass can be measured in imperial units such pounds (lbs) or metric units such as kilograms (kg). The standard unit of mass is the kg.
Weight, on the other hand, is a force. To be exact, it is the force which results from a body of a given mass, situated within a gravitational feld, being subjected to a gravitational acceleration as it is attracted to another mass. The standard unit of weight is the Newton (N).
On Earth, the weight of a body is the force acting on the body’s mass by virtue of the presence of the body within the Earth’s gravitational feld.
Any body subjected to the Earth’s gravitational feld which is allowed to fall will accelerate towards the ground (more exactly towards the Earth’s centre) at the same gravitational acceleration (9.81 metres/sec2 or 32 feet/sec2), whatever its mass.
A body will always possess mass, but if there is no gravity and the body is subjected to no gravitational acceleration, such as may be assumed in deep space, the body will have no weight. (See Figure 7.1, overleaf.)
As you can see from Figure 7.1, overleaf, mass is a scalar quantity, possessing magnitude only, while weight is a vector quantity, possessing both magnitude and direction.
In the Earth’s gravitational feld, weight always acts towards the centre of the Earth.
The standard
unit of mass is the kilogram.
The standard
unit of weight is the Newton.
Weight is the
name given to the force acting
on a mass, and
directed to the centre of the gravitational field.
A body will
always possess the same mass,
but if there is no
force of gravity, the body will have no weight.
129

Order: 6026
Customer: Oleg Ostapenko E-mail: ostapenko2002@yahoo.com
Customer: Oleg Ostapenko E-mail: ostapenko2002@yahoo.com
CHAPTER 7: WEIGHT
Weight in Newtons
equals mass in kilograms multiplied by the acceleration
due to gravity, 9.81 m/s2, (on Earth).
w = mg
Figure 7.1 A body will always possess mass, but where there is no gravity, there is no weight.
Gravitational Acceleration – The Link Between Mass and Weight.
The linking factor between mass and weight, then, is gravitational acceleration. So,
weight = mass × gravitational acceleration
or, using the symbols, w for weight, m for mass, and g for the acceleration due to gravity,
w = mg
Obviously, if g = 0, which we may assume to be the case in deep space, w = 0
If we express mass in kg and gravitational acceleration in metres/sec2, weight will be given in Newtons.
Although gravitational acceleration, g, decreases gradually the further a body is removed from the Earth’s surface, as far as aircraft operations are concerned we may consider g to be constant at 9.81 metres/sec2 (32 feet/sec2).
So, for example, the weight of a 5 kg bag of potatoes, on Earth, can be easily found using the formula:
w = mg
w = 5 kg × 9.81 metres/sec2 = 49.05 Newtons
In deep space, however, the 5 kg bag of potatoes will still possess a mass of 5 kg, but its weight will be 0, as depicted in Figure 7.1.
On the Moon, where the force of gravity is weaker than on Earth (the Moon being less massive than the Earth), gravitational acceleration is only 1/6 of the Earth’s acceleration. So on the Moon, g = 1.635 metres/sec2.
130

ID: 3658
Customer: Oleg Ostapenko E-mail: ostapenko2002@yahoo.com Customer: Oleg Ostapenko E-mail: ostapenko2002@yahoo.com
CHAPTER 7: WEIGHT
Consequently, although the mass of the bag of potatoes that we have been considering will still be 5 kg on the Moon, its weight will be less. The weight of the 5 kg bag of potatoes on the Moon can be calculated as follows:
w = mg
w = 5 kg × 1.635 m/sec2 = 8.175 Newtons, (1/6 of its weight on Earth.)
Figure 7.2 On the Moon, the bag of potatoes has the same mass as on Earth, but only 1/6 of the weight.
The bag of potatoes, then, possesses the same mass, on the Earth and on the Moon, but, on the Moon, the weight of the bag of potatoes is only 1/6 of its weight on Earth.
(See Figure 7.2.)
What Does 1 Newton of Weight Feel Like?
Well, an average sized apple weighs around 100 grams which is 0.1 kg. 9.81 metres/sec2 is almost 10 metres/ sec2; therefore, from the equation:
w = mg
we can estimate, without too much inaccuracy, that, on Earth, an apple weighs around 1 Newton (N).
w = mg = 0.1 kg × 10 m/s2 = 1 N
By supporting an apple in your hand, then, you can get an idea of the feel of
1 Newton of weight.
Figure 7.3 An averaged sized apple weighs in the region of 1 Newton.
A body of a
given mass will weigh six times
less on the
Moon than on Earth.
131

Order: 6026
Customer: Oleg Ostapenko E-mail: ostapenko2002@yahoo.com
Customer: Oleg Ostapenko E-mail: ostapenko2002@yahoo.com
CHAPTER 7: WEIGHT
The General Force
Equation tells us that force
in Newtons equals mass in kilograms multiplied by acceleration in m/sec2.
In horizontal acceleration,
it is mass which must be
considered.
When we lift a body against
the force of gravity, it is weight which must be
considered.
The General Equation Linking Force and Acceleration.
Remember, too, that weight is a force: the force pulling a mass to the ground. So, by holding up an apple, you can also get an idea of the magnitude of 1 Newton force.
In fact, if we re-write the weight equation, substituting force, F, for weight, w, and a general expression for acceleration, a, instead of g for gravitational acceleration, we obtain:
F = ma
F = ma is the general acceleration formula, and an expression of Newton’s 2nd Law of Motion which states that the acceleration of a body is proportional to the out-of- balance resultant force acting on the body.
Now, if we wished to accelerate a vehicle of, say, mass 1000 kg along a horizontal path, we are not concerned, in the frst instance, with the vehicle’s weight. Provided we ignore friction and rolling resistance, which we must do for this comparison of the concepts of weight and mass, weight (which acts vertically downwards) can have no affect on the horizontal motion of a mass which takes place in a plane lying perpendicularly to the line of action of the weight.
So, if we also ignore air resistance, it would not matter whether the vehicle of 1000 kg mass were being accelerated horizontally on the Earth’s surface, or on the Moon’s surface, where gravity is only 1/6 of the Earth’s gravity. If we wished to impart to the vehicle a horizontal acceleration of, say, 2 metres/sec2 , we would have to apply the same force on the Earth as on the Moon. We can calculate this force using the equation:
F = ma
F = 1000 kg × 2 metres/sec2 = 2000 Newtons
2000 Newtons, then, would be the force required to impart a horizontal acceleration of 2 metres/sec2 to the vehicle, whether on the Earth or Moon, because, on both celestial bodies, the mass of the vehicle is 1000 kg.
But if we wished to lift the same 1000 kg vehicle, vertically, to load it on a transport device, for instance, the situation between the Earth and the Moon would be very different.
To lift the vehicle, we need to apply an upwards acting force which equals the vehicle’s weight. To calculate that force, we need to use the formula:
w = mg
Now, g, the acceleration due to gravity, is, on Earth, 9.81 metres/sec2, in standard units. We will designate this value by the symbol gE.
But, g, the acceleration due to gravity, on the Moon, is only 1.635 metres/sec2. We will designate this value by the symbol gM.
132

ID: 3658
Customer: Oleg Ostapenko E-mail: ostapenko2002@yahoo.com Customer: Oleg Ostapenko E-mail: ostapenko2002@yahoo.com
CHAPTER 7: WEIGHT
So the force required to lift the vehicle of mass 1000 kg, on Earth, is given by
w = m gE = 1000 kg × 9.81 metres/sec2 = 9810 Newtons
Whereas, the force required to lift the vehicle of mass 1000 kg, on the Moon, is given by
w = m gM = 1000 kg × 1.635 metres/sec2 = 1635 Newtons
Weight is Different from Mass.
The 1000 kg vehicle, having the same mass everywhere, requires the same force to accelerate it horizontally, if we ignore issues like air resistance and friction, whether it is located on the Earth or on the Moon. But, if we wish to lift the vehicle, we have to apply a force to overcome the weight that the vehicle possesses by virtue of its presence within a particular gravitational feld. The lifting force required to counterbalance the weight of the vehicle needs to be 6 times greater on the Earth than on the Moon.
We can see clearly, then, that a body’s weight is quite different from its mass.
Mass and Weight Applied to Aircraft Operations.
So in considering an aircraft’s horizontal acceleration, or deceleration, on a horizontal runway, only the thrust force developed by the propeller and the aircraft’s mass are of interest, if we ignore rolling resistance and friction. The scientifc consideration in horizontal accelerations is the aircraft’s mass, not its weight.
But, as soon as the aircraft gets airborne, the lift force generated by the wings has to counterbalance the weight of the aircraft, if the aircraft is to remain fying. So when we consider the lift force that must be generated at take-off, or to carry a given payload over a given distance at constant speed, it is concept of weight that we are concerned with, not mass.
Why the Confusion Between Weight and Mass?
So why do people confuse mass and weight?
Why, in aviation, do people talk about mass to mean both mass and weight?
And why, in aviation, is the kg used to express both mass and weight, when we know that scientists measure mass in kg and weight in Newtons?
Well, the reason why people confuse mass and weight, in terms of names given to two separate concepts, is that, in a gravitational feld of constant strength, a given mass will always possess the same weight.
In a gravitational feld like that of the Earth, as long as we remain near the Earth’s surface (and, in that sense, aircraft, even at 40 000 feet, are always near the Earth’s surface), the gravitational acceleration, g, may be regarded as constant at 9.81 metres/sec2. So, in the equation, w = mg, because g is a constant, w will always vary in the same proportion as g. So if we double the mass we double the weight, and if we quadruple the mass we quadruple the weight, and so on.
133
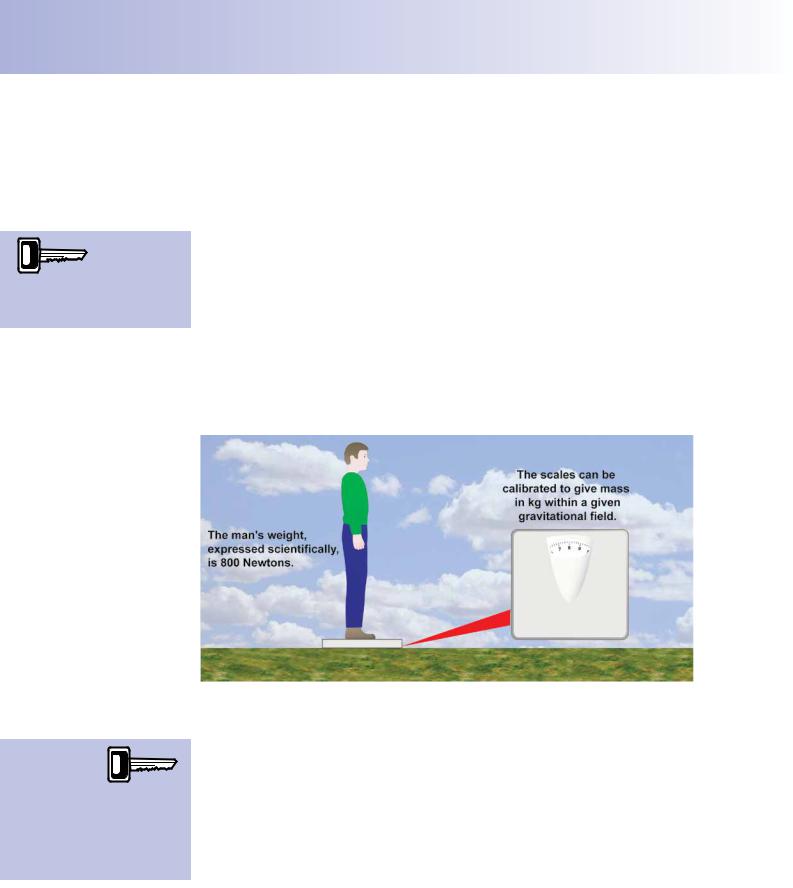
Order: 6026
Customer: Oleg Ostapenko E-mail: ostapenko2002@yahoo.com
Customer: Oleg Ostapenko E-mail: ostapenko2002@yahoo.com
CHAPTER 7: WEIGHT
In a gravitational
field of constant strength, a
given mass will always have the same weight.
Because there
is a directly proportional
relationship
between mass and weight in a gravitational field of constant strength, weighing devices can be calibrated to read mass in kilograms.
Many devices, with which you will be familiar, such as bathroom scales or spring balances, are designed to measure weight, because they are measuring the force with which bodies are pulled towards the centre of the Earth. Therefore, scientifcally speaking, these weighing devices should be calibrated in Newtons. A man should say that he weighs about 800 Newtons, not 80 kilograms (taking g to equal 10 metres/ sec2).
But because, near the surface of the Earth, the relationship between the weight of a body and the mass of a body can be considered to be a constant, weighing devices that we use in our every-day life, such as bathroom scales, are calibrated to give their readings in kilograms, the unit of mass.
This practice is not scientifcally accurate but it is a system that we are used to and, in that part of the Earth’s gravitational feld in which we live, where g is constant at approximately 10 metres/sec2, it is a system which enables us to talk about weight in kilograms, and even to claim that we are measuring mass! As long as you understand the difference between mass and weight, this is a system that will give us useful information and that we can accept. (See Figure 7.4.)
Figure 7.4 On Earth, bathroom scales can be calibrated to show mass in kilograms, but these type of scales are, in reality, measuring weight.
But never forget that it is acceptable to use this system of expressing weight in kilograms only because of the constant relationship between mass and weight which exists near the Earth’s surface. Given that constraint, it is acceptable to calibrate bathroom scales to tell a man that he weighs 80 kg instead of 800 Newtons, and to say that the bathroom scales read mass as well as weight. However, the same bathroom scales would not give an accurate reading of mass on the Moon.
On the Moon, the 80 kg man, would see only 13.3 kg displayed on the scales! But as we now know that mass is the same everywhere, we can see the error immediately, and understand why the man would be surprised. In order to measure his mass on the Moon, the man would have to use a chemical balance, and compare himself with a known mass. (See Figure 7.5.)
134

ID: 3658
Customer: Oleg Ostapenko E-mail: ostapenko2002@yahoo.com Customer: Oleg Ostapenko E-mail: ostapenko2002@yahoo.com
CHAPTER 7: WEIGHT
Figure 7.5 To measure mass accurately, in any gravitational field, the mass to be found is compared with a known mass, using a chemical balance.
Using the chemical balance, both masses are being acted upon by the same gravitational feld. Mass measurements are straight forward to make in this way.
Of course, if the bathroom scales had been calibrated to read 800 Newtons for the weight of the man on Earth, he would not be surprised to learn that he weighed only
133 Newtons on the Moon, knowing that the Moon’s gravitational feld is only 1/6 as strong as that of the Earth.
On the Earth, in a constant gravitational feld, we can get away with pretending that weight can be called mass, and with measuring weight in kg, as if it were mass. Consequently, in aviation, you will often sometimes meet weight and mass spoken about as if they were the same concept, and, where the word weight is used correctly, you will most often fnd weight measured in kilograms. You are now in a position to understand and to forgive this confusion.
Just remember, though, that when you see a light aircraft with its three wheels standing on weighing devices (not “massing” devices”), it is the aircraft’s weight that is being measured, even though that weight is expressed in kilograms and might also, sometimes, be expressed as the aircraft’s mass!
Weight – One of the Forces Acting on an Aircraft in Flight.
Now, having learnt the difference between weight and mass, and understood why some people sometimes confuse the two, and why weight is commonly and unscientifcally measured in kilograms, we may now look at weight as one of the forces which act on an aircraft in fight.
The weight of an aircraft is the force exerted by the Earth’s gravity which, acting on the aircraft’s mass, acts vertically downwards through the aircraft’s centre of gravity, tending to pull the aircraft towards the Earth’s centre.
In aviation, you
will often hear weight and
mass spoken
about as if they were the same concept, with both weight and mass measured in kilograms. This system works, as long as we bear in mind the reason why it works.
135
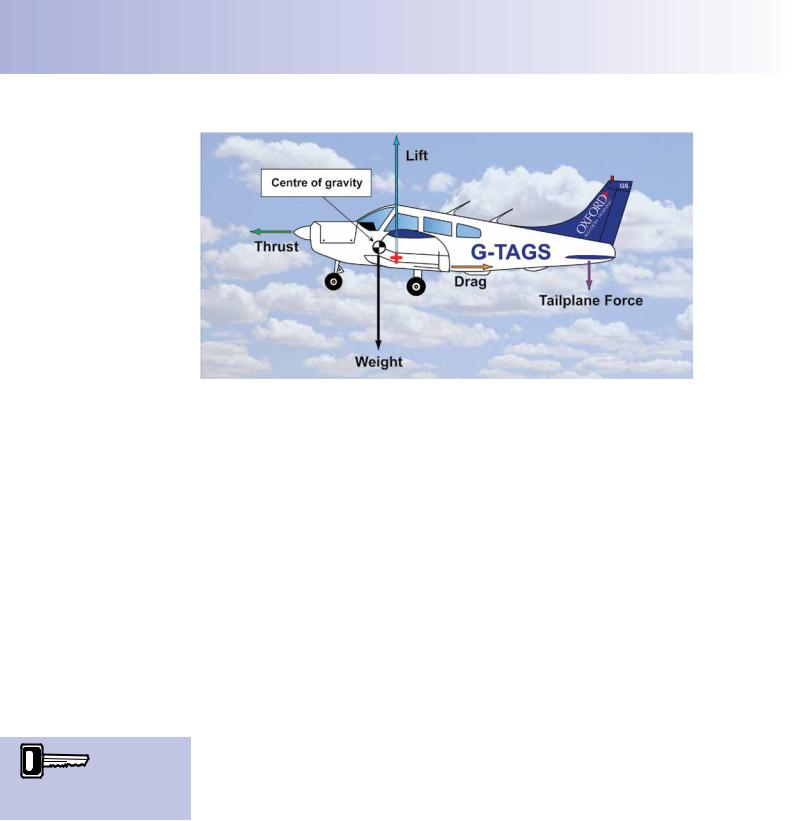
Order: 6026
Customer: Oleg Ostapenko E-mail: ostapenko2002@yahoo.com
Customer: Oleg Ostapenko E-mail: ostapenko2002@yahoo.com
CHAPTER 7: WEIGHT
Weight always acts vertically
downwards towards the
centre of the Earth.
Figure 7.6 The aircraft’s weight acts vertically downwards. The total weight of the aircraft is considered as acting through the aircraft’s centre of gravity.
Of course, as we perceive weight, we should simply say that weight is the force pulling the aircraft towards the ground. Although each aircraft component will have its own weight, the total weight of the aircraft is considered as acting through the aircraft’s centre of gravity. (See Figure 7.6.)
If the aircraft is to fy, the force of weight must be opposed by the force of lift. In steady, straight and level fight (level means at constant altitude), lift, then, must equal weight and must act in the opposite direction to weight. If lift is greater than weight, the aircraft will begin to accelerate upwards; if weight is greater than lift, the aircraft will begin to accelerate downwards. But that is another story.
In Figure 7.6, the lift force is shown exactly counterbalancing the weight, in terms of the size of the forces (magnitude) and their direction. Other fight forces are shown in Figures 7.6, but they will not be mentioned in this chapter. On diagrams depicting the fight forces, it is conventional to show forces as arrows, or vectors, where the length of the arrow is representative of the magnitude of the force, and the direction of the arrow shows the line of action of the force.
The Centre of Gravity.
The centre of gravity, is the point within a body through which all of a body’s weight is considered to act. If an aircraft were to be suspended by a single force, say a rope, attached to the aircraft’s centre of gravity, we could place the aircraft with its longitudinal axis horizontal, and the aircraft would remain horizontal in perfect balance, as depicted in Figure 7.7.
In fact, if the aircraft were suspended exactly through its centre of gravity, we could put the aircraft in any attitude we wished, and it would remain in that attitude, because there could be no out-of-balance force to make it move.
In fight, an aircraft, when manoeuvred, rotates about its centre of gravity, but the aircraft’s weight always acts vertically downwards towards the centre of the Earth. The magnitude of the weight force is of considerable importance because of its effect on aircraft structural integrity; the distribution of the variable elements of the total mass (fuel, passengers and crew, baggage, etc.) will affect the position of the centre of gravity.(See Mass and Balance in Book 6).
136