
The Elisa guidebook
.pdfIn general, antibodies serve to regulate parasites that exist in the bloodstream and tissue fluids, but they are ineffective once the parasite has become intracellular. Hence, the importance of antibody varies with the infection under study. The presence of encysted morphological forms in the host also reduces the efficacy of antibodies (e.g., Toxoplasma, Entamoeba, Giardia). Many protozoan parasites undergo development in the mammalian host, which is manifested in morphologically distinct form. Such developmental forms often have associated stage-specific surface antigens.
Antibodies can damage parasites directly, induce lysis, activate complement, agglutinate extracellular forms, stimulate antibody-dependent cellular cytotoxicity, and block their entry into their host cells. IgM, IgG, and IgA antibody isotypes are involved in these reactions. The isotype specificity not only depends on in which host compartment the parasite is residing (e.g., respiratory tree, GI tract, GU tract [IgA], bloodstream, lymphoid tissue [IgM, IgG]) but also on the antigens expressed during the different developmental stages and their chronicity.
Page 136
Protozoan parasites can induce chronic infections in mammals, and therefore large amounts of antibody (IgM + IgG) are produced in response to infection. The external surfaces, and the antigens contained therein, are important in the control of protozoan infections and many effective/protective antibody mechanisms are directed against them.
Protozoan parasites are capable of the polyclonal stimulation of B-cells, and, hence, in some infections (e.g., trypanosomiasis) large quantities of non-trypanosomal IgM and IgG antibodies are produced. In such instances, the immunoassayist should have the capability of distinguishing parasite-specific from nonspecific antibody responses. Protozoan parasites are also capable of depressing the immune response (immunodepression) (e.g., Babesia), and in such instances circulating antibody levels are reduced. The above phenomena must be borne in mind when devising ELISAs for protozoan parasites.
5.5.4¡ª
Effect of Antibody in Helminth Infections
Helminths (trematodes, cestodes, nematodes, Acanthocephalans) normally have complicated life cycles, and their larger size and increased complexity cater for the increase in antigenic diversity found within them. In addition, when many developmental forms exist in the host, a stage specificity of antigens (restricted to each stage) has been demonstrated. Helminth parasites induce chronic infections, and such long-term antigen challenge exposure can produce elevated levels of circulating antibodies. Because helminth parasites are normally transmitted by close contact, vectors, water, and possibly aerosolization, they affect numerous tissues and organs of the host. Some helminths have a minimal migratory phase, but the majority are capable of migration throughout the host's soft tissues.
Antibodies of IgM, IgG, IgA, and IgE isotypes are produced in response to helminth antigens, and depending on in which physiological compartment of the host the parasite is residing, each isotype can be utilized in ELISAs for detecting antibody recognition (exposure) of parasite antigens. IgE Igs are elevated in helminth infections, and although only a proportion of the IgE Ig produced has antibody activity against parasite antigen(s), this class of Ig has been implicated in resistance to helminth. Both IgG and IgE antibodies are involved in antibody-dependent cellular cytotoxicity mechanisms against helminth parasites.
Helminth parasites are the most complex infectious disease agents infecting mammals, and hence the host's antibody responses to them are the most diverse. Unlike other infectious diseases, most helminths do not divide in the body of the final host, and therefore the antigenic load is dependent on the infective dose. Exceptions to this are Echinococcus spp. and Strongyloides spp. Antibodies are produced against antigens present within helminths (somatic
Page 137
antigens), antigens on helminth outer surfaces (surface antigens) and antigens in helminth excretions or secretions (excretory-secretory [ES] antigens). The ES antigens of helminths have been shown to confer the most specificity in immunoassays. Not all stages of the helminth life cycle occur in the mammalian host, and therefore it is vital that the immunoassayist consider the pathogen of interest with reference to this. Antigens collected or extracted from stages of the parasite that do not occur in the final host are unlikely to be of use for detecting antibodies to the parasite in that host. Similarly, because helminths develop within the host, the earlier mammalian stages of development stimulate antibodies against antigens of only that stage. Later developmental stages stimulate antibodies not only to their respective stages but also to previous stages if homologous or crossreacting antigens occur in such stages. The humoral immune responses to helminths are complex and care is necessary in developing ELISAs for helminth infections in order that cross-reactions be minimized. Helminths are capable of modulating the immune response, and immunosupression of antibody responses occurs in some diseases (e.g., Haemonchus).
5.6¡ª
Overview on Antibody Production
In the previous sections, the complexity of the humoral response to infectious disease agents was demonstrated. As the agents become structurally more complex, a greater degree of antigenic diversity arises, which can complicate immunodiagnosis. However, it is apparent that infectious disease agents stimulate the production of specific antibodies in hosts, and these antibodies can be used in ELISAs to determine the exposure of a host to a pathogen. Similarly, interactions between host and pathogen cause the release of pathogen antigens into the host's bodily fluids. These antigens can be used in ELISAs to detect current infections. In endemic areas mammals may be infected with more than one pathogen. In cases in which antigens of each pathogen are noncrossreactive with antibodies produced in response to each pathogen, diagnosis by immunoassay is straightforward. Difficulties will inevitably arise when crossreacting antigens (possibly from closely related pathogens) and antibodies occur. Care must be taken when developing ELISAs for such systems, and basic research to define the problems of crossreactions must be undertaken.
In considering antigens of potential usefulness in immunodiagnosis, it is apparent that the greater the number or variety of antigens used to detect circulating antibodies, the wider the antibody diversity detected will be, and hence the likelihood of detecting positive cases. However, owing to the complexity and cross-reactivity of antigens and antibodies, the immunoassayist must select the antigen or group of antigens that stimulate antibody production but do not produce crossreactions.
Page 138
5.7¡ª
Relationship between Antigen and Antibody in Vivo
We have seen that the ingress of antigens into the host's body eventually leads to the production of circulating antibodies to them. In the case of complex antigens (e.g., infectious disease agent antigens), each antigen can stimulate the production of a variety of antibody isotypes with a variety of affinities, and these antibodies can be used in ELISA for the immunodiagnosis of infectious diseases. In certain infectious diseases (e.g., viruses, bacteria, protozoa) in which the pathogens divide in the host's body, as the pathogen burden increases, so does the antigenic load. Similarly, when pathogens are dealt with effectively (e.g., by lysis) the release of previously internalized antigens into the surrounding tissue occurs, thus increasing the free antigenic load in the host.
One function of antibodies is to mop up free antigen and cause the antigen/ antibody complex produced to be removed from the system, normally by phagocytic cells. It is important to consider the kinetics of antigen and antibody appearance and disappearance when dealing with antibody responses to infectious disease agents.
In the early stages of a primary infection, very little circulating antibody is present. When the infectious disease antigens are processed by the host, antibody synthesis occurs and these antibodies, predominantly of the IgM isotype, recognize specific antigens. In this stage of the disease process, antigen levels would be expected to be higher than antibody levels in the fluid sampled, i.e., excess antigen. Because there are relatively higher concentrations of antigen than antibody, all available antibody will be bound to antigen to form immune complexes, leaving the residual antigen free to circulate in bodily fluids. As more antibody is produced in response to continuous antigen challenge, the relative concentrations of antibody and antigen become similar, and as this antibody binds to antigen, less free antigen is present. At a point where the amount of circulating antibody equals the amount of free antigen, following antigen/antibody interaction to produce immune complexes, neither free antibody nor free antigen remains, and at this point both reactants are equivalent. When the concentration of circulating antibody exceeds the concentration of circulating antigen, no free antigen will be present, but a residual amount of antibody will be present, i.e., excess antibody.
This is a simplistic account of the interaction between antibody and antigen, but it demonstrates that whenever specific antibodies to an antigen and that antigen interact, immune complexes are produced. The antibody isotype, antibody affinity, number of epitopes on an antigen molecule, and chronicity of antigen and antibody production are all important in determining the fate of immune complexes. In general, large complexes (those found by interac-
Page 139
tion of antibodies and antigen with numerous epitopes) are removed by phagocytic cells. Smaller complexes (antibodies and antigen with few epitopes) are removed slowly by phagocytes and remain in the circulation longer. The main sites for immune complex removal are the liver (Kupfer cells), spleen, and lungs. Low-affinity immune complexes are smaller than high-affinity complexes, and therefore persist longer in the circulation. Excess antigen and excess antibody immune complexes are normally smaller than antigen/antibody equivalent immune complexes and therefore remain in the circulation longer.
Some of the pathology associated with infectious diseases is owing to immune complex deposition. Thus, in certain instances the concentration of soluble (circulating) immune complexes in bodily fluids can provide insight into immune complex pathology. The immunoassayist should be capable of devising assays for soluble immune complexes. For example, in excess antigen complexes in which an antigenspecific antibody (preferably from a different species from the host) is available, the antigen can be trapped by adsorbing the specific antibody onto the ELISA plate. The reaction can be developed with an anti-host species-specific antibody enzyme conjugate.
Because infectious diseases are often chronic, immune complexes would be expected to be produced owing to persistent antigen and antibody production. In the early stages, excess antigen would be expected, and hence the immunoassayist could measure circulating antigen. Remember that the antigen chosen for measurement has to be specific for the pathogen in question. As the disease state progresses to chronicity, less free antigen will be available owing to increased antibody production and the immunoassayist should measure circulating antibodies, or circulating immune complexes. It should be borne in mind that infectious disease organisms are antigenically complex, may or may not divide within the host, may reinfect the host, or may vary antigenically, and hence the balance previously described between antigen and antibody will vary from pathogen to pathogen. Because pathogens produce numerous antigens of different immunogenicity, many antigen/antibody interactions involving both lowand high-affinity antibodies will be present.
The most useful assays will be those that take into account the preceding considerations. The pathogen/host interactions should be examined as fully as possible before ELISAs are developed. Finally, as already mentioned, many infectious disease agents have immunomodulatory effects varying from immunodepression, including reduction in humoral antibody production, to the induction of T- or B-cell tolerance. Tolerance, in which an organism becomes unresponsive to a particular antigen, may occur in a variety of ways, but the outcome is that antibodies fail to be produced. Infectious disease agents can blockade receptors on antibody-forming cells or mature antigen-specific lymphocytes and make them unresponsive to that antigen. When high levels of
Page 140
antigen such as pneumococcal polysaccharide induce the event, a state of high zone tolerance exists. When low doses of monomeric antigen induce the event, a state of low zone tolerance exists. Thus, in designing ELISAs the effect of parasite antigens on the induction of tolerance must be considered.
5.8¡ª
Diagnostic Usefulness of Antigens and Antibodies in Infectious Diseases
Detection of both antigens and specific antibodies can prove useful in the laboratory diagnosis of infectious diseases. As we have seen, the production of specific antibodies in response to antigen challenge is multifactorial. Not only antigens and antibodies but also immune complexes can be detected by ELISA, and each will aid diagnosis. In general, free circulating antigen is present for shorter periods of time than free circulating antibody. Reinfection with the same pathogen or exacerbation will cause transient increases in free circulating antigen (antigenemia), followed by increased production of circulating antibodies. Both these events can be detected by ELISA, and the immunoassayist must be aware of this. Similarly, soluble immune complexes can be detected by ELISA, although the assay design is somewhat more complicated.
Because antigens of infectious disease agents stimulate the production of specific antibodies, the latter can be used in ELISAs as an indicator of infection. Normally the antibody response is long-lived and often is present in the absence of the inciting antigens. For this reason, the detection of antibodies in the host does not indicate the presence of a current infection, but does indicate exposure. Antibodies to some antigens are more persistent than antibodies to other antigens and, in some instances, may persist for the lifetime of the individual. In such instances, the question, Is the host immune or refractory to reinfection? cannot be answered by detecting antibodies to antigen mosaics of pathogens. Questions such as, Is the residual antibody effective in the prevention of reinfection? can only be answered when the biological effects of these antibodies are known.
Effects such as neutralization, agglutination, attachment onto receptors to prevent intracellular localization, and lysis are well-known biological effects of antibodies on infectious disease agents. If the mechanism of immunity is known (i.e., the effect of antibody on the target antigens), and if the target antigens can be isolated, they can be used in ELISAs to monitor the rate of production and duration of protective antibody. Few of these assays are available at present, and therefore the immunoassayist must utilize other general phenomena associated with the development of the immune response. The following situations expand this idea.
Page 141
5.8.1¡ª Neonates
Maternal antibody of the IgG isotype is transmitted passively to neonates transplacentally and in the colostrum. Other isotypes are also found in colostrum and milk (IgA, IgM), but are not transmitted across the neonatal GI tract. Both IgM and IgG antibodies to antigen can be assayed for, and when IgG antibodies are present in the absence of IgM antibodies, the likelihood of them being passively transmitted is high. When humoral IgM antibodies occur, then the organism must be synthesizing them de novo. If antibodies are assayed for in other physiological compartments of the body (e.g., GI tract ) these conclusions become invalid since both maternal IgM and IgA are secreted in milk and may still be biologically active as co-proantibodies.
5.8.2¡ª
Immunologically Competent Mammals
We have seen that IgM is the first isotype of humoral antibody produced in response to antigenic challenge and that if the challenge persists it is replaced by IgG isotype. If the infection is chronic and the antigen persists, or reexposure occurs, higher levels of IgG will be produced. This information is valuable to the immunoassayist because when total antibody (all isotypes) is assayed, an increase in antibody titer over time is indicative of a current infection. This is also true when isotype-specific second antisera are used. The comparison of IgM and IgG levels is also a useful indicator.
Persistent antigen induces an isotype switch in T-cell-dependent antigens, stimulating the production of IgG rather than IgM. If IgM and IgG levels to the same antigen are compared, the following can be deduced: IgM antibody in the absence of IgG antibody means there has been recent acquisition of infection in which no isotype switch has occurred as yet. IgM and IgG antibody present means prior acquisition of infection, in which isotype switch has occurred, with IgM response present but possibly declining. Prior acquisition of infection where isotype switch has occurred, means that the host has become reexposed to the same antigen, stimulating the production of more IgM antibody.
IgG antibody in the absence of IgM antibody could mean the previous acquisition of infection, in which isotype switch has occurred, and the IgM response is below the assay detection level. In this case, the infection would be expected to be chronic.
When antigens are localized in the respiratory, the GI, tract or GU tract, stimulating local antibody responses, the detection of IgA antibody isotypes would be of value. Similarly, when it is known that pathogens stimulate isotype-specific responses (e.g., helminth), IgE-specific antibody responses can be assayed.
Page 142
5.8.3¡ª
Herd Immunity
Each individual in a group has a varying potential to respond to antigens and hence to mount a protective immune response. The range of immune responses in a group follows a normal distribution pattern whereby the majority of individuals mount an average immune response, and a small proportion mount either a very effective immune response or an ineffective immune response. When vaccination or exposure to a pathogen is concerned, <100% of the population will be adequately protected, and for those individuals concerned, this lack of protection will be serious. This lack of protection may be owing to the inability to recognize antigens or to produce antibodies (agammaglobulinemia). These individuals can act as reservoirs of pathogens for future transmission to other susceptible individuals, and the seriousness of the situation will depend on the mode of transmission, the number of susceptible individuals, and in cases in which vaccination is common practice, the efficacy of vaccination.
Less than 100% protection may be sufficient to prevent the spread of the disease within the population, since the likelihood of a susceptible individual encountering an infected individual becomes reduced. This phenomenon is known as herd immunity and is an important consideration in assessing the potential of individuals in a population to succumb to infection.
Many factors can affect the quantity and quality of an immune response, most of which have been mentioned previously. Other factors that affect the immune response are stress, pregnancy, surgery, concomitant infections, extremes of temperature, and especially malnutrition. All these factors reduce the quantity and quality of the immune response, and when the immunoassayist performs seroepidemiological surveys to assess the herd immunity among various populations, these factors must be taken into account prior to determining levels of adequate protection within a population.
For each infectious disease, antibody levels (if any) in naive, subclinically infected, clinically infected, and immune individuals in the endemic population should be sought. Only in this way can the immunoassayist relate antibody levels to infection. Once these parameters are known, the effect of vaccination in these individuals can be assayed as well as the relationship between protective antibody and immunity.
One major problem is that individuals in an endemic area have had previous exposure of an infectious disease agent, or antigens thereof, and therefore ascribing an antibody titer or threshold above which protection occurs and below which reinfection occurs is difficult, if not impossible.
The situation becomes more complex when assessing the protective effects of vaccination in such a group of individuals. Often individual antibody levels
Page 143
are static owing to chronic exposure and variable owing to previously mentioned factors. In the instances in which naive individuals become immune, seroconversion occurs, but in the majority of instances where individuals have had previous exposure, increases in antibody levels are sought. These may be so small that they become statistically nonsignificant.
5.9¡ª
Antigenic Commonness
Because there is a limited number of amino acids and carbohydrates and so forth produced by living organisms, the number of combinations of these components is limited (for proteins and carbohydrates). Therefore, it is likely that different organisms will produce similar proteins, glycoproteins, and so on. When partial or complete similarities occur in the products of living organisms, a commonness in terms of antibody recognition occurs.
Structural proteins appear to be well conserved and would be expected to demonstrate immunological crossreactivity. The nature of this crossreactivity varies among similar antigenic molecules and depends on the ability of the paratope to fit the epitope.
We have already seen that the paratopes of antibodies will bind to epitopes that are recognized either completely or partially. The immunoassayist must consider the possibility that the same or similar antigens may exist in a variety of infectious agents. For example, many cell surface antigens are carbohydrate in nature and the same epitopes may be present on two cell types, one of which may be pathogenic whereas the other may be a commensal. Similarly, some carbohydrates may be present in widely differing infectious disease agents (e.g., blood group like, ascarone). When polyclonal antibodies are produced in response to infection, antibodies to noncrossreactive as well as crossreactive epitopes will be produced and hence the problem of crossreactivity can be minimized.
The problem of antigenic commonness becomes more obvious when using mAbs in diagnosis. In the developmental stage of an assay, the immunoassayist must consider which other antigens, including antigens from other infectious disease agents, might be involved in crossreactions and develop the assay accordingly, bearing in mind the type of problems associated with such crossreactions.
6¡ª
Other Techniques
The performance of good immunoassays also requires practical expertise in immunochemical techniques (or at least theoretical knowledge of when such techniques are to be used). The following list provides some techniques of use in the purification and characterization of antigens and antibodies:
Page 144
1.Sucrose density gradient centrifugation.
2.Polyacrylamide gel electrophoresis (PAGE).
3.PAGE followed by immunoblotting.
4.Isoelectric focusing.
5.Immunodiffusion in agar/ agarose.
6.Gel chromatography (DEAE, affinity, sephadex).
7.Salt fractionation of IgG.
8.Enzyme conjugation methods.
The nature and preparation of antibody fractions and their relevance in disease and assay should also be examined (i.e., whole molecule, Fc, Fab, F[ab']2, IgM, IgA).
7¡ª Units
Successful assays depend on a good knowledge of units of volume and weight. The concepts of accuracy in dilutions and the relevance of pipetting methods also fall within the necessary practice needed for assays.
7.1¡ª Volumes
The pipets used in microtiter plate assays are graduated in microliters (µL). The relationship of volumes is given in Table 2.
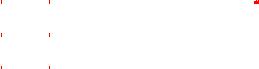
7.2¡ª Weights
Note the relationship of weights as given in Table 3.
8¡ª Dilutions
Difficulties are often encountered in the making of dilutions. It is essential that great care be taken in making the correct dilution and that there be no wasting of expensive reagents through making up convenient dilutions into an unneeded final volume. For example, we may need to make up a 1/1200 dilution of a sample already at a 1/50 dilution in a final volume of 5.5 mL. Often operators attempt to round up volumes so that larger than necessary volumes are made, which is wastes of reagents (e.g., conjugates). Other problems, such as making a 1/20,000 dilution in a final small volume such as 3 mL arise.
All problems of dilution are eased if all volumes in the calculation are converted to microliters (µL). The following examples illustrate this.
8.1¡ª
Making a 1/100 Dilution of Neat Sample at a Final Volume of 10 mL
Neat infers a sample is undiluted. Thus, we require a final volume of 10 mL. Convert the required volume to microliters:
Page 145
Table 2
Relationship of Volumes in Microtiter Assays
Volume |
Symbol |
Cubic centimeter |
Microliters |
|
Liter |
|
L |
1000 |
1,000,000 |
|
||||
Milliliter |
|
mL |
1 |
1000 |
|
||||
Microliter |
|
µL |
0.001 |
1 |
|