
Metal-Catalysed Reactions of Hydrocarbons / 13-Reactions of the Lower Alkanes with Hydrogen
.pdf
REACTIONS OF THE LOWER ALKANES WITH HYDROGEN |
545 |
TABLE 13.5. Dependence of the Values of the Constants for Ethane Hydrogenolysis on Ru/A12 O3 at 473 K on the Form of Rate Expression Used63,64
Code |
|
|
R |
k+ |
|
|
KA |
|
|
bH /atm−1 |
ES2 |
kyPH (y + PHa ) |
1.44E−4 |
1.06E−3 |
— |
||||||
|
|
+ |
a |
|
− |
|
|
− |
|
|
ES3 |
kPA /(1 |
|
bH PHa − 1 ) |
8.16E |
|
5 |
3.24E |
|
2 |
— |
ES4 |
kyPH /[y + PH (1+(bH PH )1/2)] |
1.52E−4 |
1.45E+1 |
1.1E+7 |
||||||
ES5 |
kyPH /D |
|
1.53E−4 |
2.08E+7 |
7.31E+3 |
+ In units of mol gRu −1 s−1 atm−1 .
The values of the constants of the rate expression, however, depend critically on the form of the expression used:64 results for the four tested by Shang and Kenney63 are shown in Table 13.5. While values of k are reasonably constant, those for KA vary by more than 1010, presumably because their dimensions are different in each expression: but even bH in the cases where it does appear differs by more than 103, although its units are the same. This sensitivity to mathematical form does not assist the process of identifying the ‘right’ equation. The equation coded ES5B (Table 13.4) has been selected65 as giving values of KA and bH that are of similar size.64
The availability of microprocessor control of the reactor system greatly improves the speed and accuracy with kinetic measurements can be made, and the number of levels of the variables that can be used. The better quality permits a more rigorous comparison between alternate rate expressions, and increases the reliability of the derived constants for that selected. These advantages have been exploited in extensive studies (see ‘Further Reading’ list) of the hydrogenolysis of the lower alkanes on standard platinum catalysts,66−69 as the following examples will illustrate.
Comparison of the rate dependence upon hydrogen pressure for the hydrogenolysis of ethane, propane and n-butane on Pt/Al2O3 (EUROPT-3) (Figure 13.10) shows that the forms of the curves differ markedly in respect of the position and sharpness of the rate maximum.64,65,70 This explains why (if it were not already clear) the ‘order’ depends on the range of hydrogen pressure used, and why the ‘order’ measured at say 0.3 to 0.5 atm pressure becomes more positive as chain-length increases. Qualitatively it is clear that the larger the alkane, the more easily is it chemisorbed and the more effectively can it compete with hydrogen for the surface. The values of the constants of equation ES5B give quantitative support (Table 13.6): with both Pt/SiO2 and Pt/Al2O3 the ratio KA/bH increases with chain-length, although with the more active Pt/SiO2 at a 75 K lower temperature values of KA are smaller, while those of bH are similar (see below). These observations incidentally deprive the question ‘which alkane is the most reactive?’ of any meaning.70
For each alkane the form of the kinetic curve changes with temperature,65 the rate maximum moving to higher hydrogen pressures and becoming less distinct

546 |
CHAPTER 13 |
Figure 13.10. Dependence of rates of hydrogenolysis of C2 to C4 alkanes on hydrogen pressure over Pt/Al2 O3 (EUROPT-3) at 608 K.64,65
TABLE 13.6. Values of the Constants of Equation ES5B for Hydrogenolysis of the Lower Alkanes64
Metal |
Support |
Alkane |
T /K |
k |
KA /atma−1 |
bH /atm |
a |
Rua |
A12 O3 |
C2 H6 |
418 |
29 |
0.60 |
18 |
1.83 |
Rua |
A12 O3 |
C3 H8 |
418 |
80 |
17 |
25 |
2.34 |
Rua |
A12 O3 |
n-C4 H10 |
418 |
116 |
39 |
34 |
1.34 |
Rh |
A12 O3 |
n-C4 H10 |
430 |
189 |
47 |
63 |
1.75 |
Rh |
SiO2 |
n-C4 H10 |
430 |
151 |
30 |
70 |
0.86 |
Rh |
TiO2 |
n-C4 H10 |
430 |
36 |
6.6 |
18 |
1.27 |
Ptb |
A12 O3 |
C2 H6 |
608 |
33 |
10 |
9 |
2.25 |
Ptb |
A12 O3 |
C3 H8 |
608 |
45 |
18 |
5 |
1.52 |
Ptb |
A12 O3 |
n-C4 H10 |
608 |
57 |
27 |
2 |
0.88 |
Ptc |
SiO2 |
C3 H8 |
533 |
9 |
0.7 |
5 |
1.41 |
Ptc |
SiO2 |
n-C4 H10 |
533 |
11 |
2.3 |
6 |
0.90 |
a 1% Ru/A12 O3 after HTR1 (see Section 13.5); b EUROPT-3;
c EUROPT-1.

REACTIONS OF THE LOWER ALKANES WITH HYDROGEN |
547 |
Figure 13.11. Dependence of rate of hydrogenolysis of n-butane on hydrogen pressure at various temperatures on Pt/Al2 O3 (EUROPT-3).64,65
as temperature increases (Figure 13.11).64,65 Hydrogen chemisorption becomes weaker and its coverage decreases, and ‘order’ becomes less negative. This immediately implies (if it were not already obvious) that the activation energy measured at different hydrogen pressures must increase with the pressure used, as is shown in Figure 13.12. Apparent activation energy derived from rates of reaction is therefore not an absolute quantity, and varies with the reactant pressures used.71 Moreover activation energies obtained by altering hydrogen pressure also exhibit compensation, the parameters Ea and ln A being consistent with those found when different alkanes, and different but similar catalysts, are used (Figure 13.13). Compensation between Arrhenius parameters can therefore occur within a single reaction system, and their dependence on operating conditions must go some way to explaining the spread of their values as revealed in Figures 13.3 and 13.5–13.8.71
Application of the rate equation ES5B (Table 13.4) to results for the lower alkanes obtained with EUROPT-3 (0.3%Pt/Al2O3, AKZO CK303) (and to the corresponding rhenium-containing catalyst, EUROPT-4) generated 65 values of the constants k, K3, bH and x at various temperatures, and the use of the Arrhenius equation on k and of the van’t Hoff isochore on K3 and bH yielded satisfactorily linear plots from which a true activation energy Et and heats of adsorption for the alkane (− HA) and hydrogen (− HH) were derived. Values for Et and − HA were respectively 82 and 88 kJ mol−1 for propane, and 76 and 79 kJ mol−1 for n-butane, but for − HA were about zero. This was excused on the basis that measurements related to high surface coverage, at which low values were to be expected. A further curious feature of the results was a marked correlation between

548 |
CHAPTER 13 |
Figure 13.12. Dependence of apparent activation energies for hydrogenolysis of propane and of n-butane over Pt/Al2 O3 (EUROPT-3) on hydrogen pressure: the curves are calculated using equation ES5B and the constants in Table 13.6.64,65
k and KA, which may have had physical significance or may have been an artefact of the kinetic model. These procedures have been further discussed,65 and applied to results obtained with Ru/Al2O3 catalysts. Three-dimensional plots of rate versus alkane and hydrogen pressures for the three linear alkanes emphasise their very different characters (Figure 13.14). The dependence of apparent activation energy on reactant pressures and hence on surface concentrations is implicit in the Temkin equation (5.29), where the contributions of the heats of adsorption to the true activation energy are moderated by the reaction orders ni thus:
Ea = Et + nA HA + nB HB |
(13.10) |
A zero order reflects a lack of dependence of coverage on temperature, and so the relevant heat term disappears. Ea will then decrease as the pressure of that reactant is increased. Application of this equation to the hydrogenolysis of alkanes is not straightforward, because Ea actually increases with hydrogen pressure72 (Figure 13.12). This is because the concentration of the dehydrogenated reactive intermediate increases with temperature because of the endothermic nature of its formation, and because the desorption of hydrogen provides more vacant sites for

REACTIONS OF THE LOWER ALKANES WITH HYDROGEN |
549 |
Figure 13.13. Compensation plot for the total reaction of C2 to C4 alkanes with hydrogen on Pt/Al2 O3 (0.3% Pt, open points and 0.6% Pt, filled points):33 circles, C2 H6 ; triangles, C3 H8 : squares, n-C4 H10 ; diamonds, iso-C4 H10 . The larger triangles and circles derive from the variation of hydrogen pressure (Figure 13.12) for C3 H8 and iso-C4 H10 respectively.
it. The experimentally estimated upper and lower limits for Ea (Figure 13.12) can be compared with the values stemming from the ES5B equation, because
Ea (max) = Et + nA |
HA |
(13.11) |
Ea (min) = Et + nH |
HH |
(13.12) |
where A = alkane, and the n’s the respective ‘orders’. Agreement between the measured and calculated values of Ea is fair rather than good, but perfect agreement is not to be expected because the limiting values of the ‘orders’ are not easily estimated, and the rate expression used may not be entirely valid. The comparison does however supply another means by which the utility of a rate expression may be judged.
13.2.4. A Generalised Model for Alkane Hydrogenolysis
It now becomes possible to sketch a qualitative outline of a model describing kinetic aspects of hydrogenolysis of the lower alkanes.33,71 Let us imagine three curves representing the dependence of rate on hydrogen pressure; for simplicity they are normalised to the same maximum rate (Figure 13.15). The order nH

550 |
CHAPTER 13 |
Figure 13.14. Three-dimensional plots for the hydrogenolysis of ethane (A), propane (B) and n-butane (C) on Pt/Al2 O3 (EUROPT-3) at 608 K calculated using the constants for equation ES5B in Table 13.6.65
Figure 13.15. Schematic diagram showing rate dependence on hydrogen pressure in three different circumstances (see text).30,71

REACTIONS OF THE LOWER ALKANES WITH HYDROGEN |
551 |
Figure 13.16. Schematic diagram showing the dependence of rate, order in hydrogen and apparent activation energy on hydrogen coverage (θH ) (see text): the zones A, B and C are those located about the dashed line in Figure 13.15.30,71
measured in any fixed range of pressure will decrease in the sequence C > B > A, so that these curves could represent either (i) metals or catalysts A, B and C arranged in order of decreasing strength of hydrogen adsorption, or (ii) increasing temperature,71 or (iii) the use of alkanes A, B and C that show increasing strength of adsorption or ease of activation. Now corresponding to each curve there will be an adsorption isotherm, so the three curves can be condensed onto a θH scale, and the ranges over which the three situations A, B and C are met can be identified (Figure 13.16). The value of Ea will be a function of θH. If one elects or is constrained to working at the tail of the curve (Case A), one finds low rate a high Ea and a negative ‘order’ in hydrogen: working near the maximum (case B) there will be faster rates, a lower Ea (= Et ) and an order nH close to zero: and at low θH (case C) low to medium rates, a low Ea and a positive value of nH. The kinetic form thus depends importantly on the magnitude of the hydrogen coverage, as had been anticipated in several earlier works. So the values of Ea obtained using a fixed hydrogen pressure under cases A, B and C will differ because the ranges of θH scanned by the temperature change will not be the same. Thus for example values of Ea found with different alkanes are necessarily not the same (Table 13.1, Figure 13.13) because the θ H ranges differ; they decrease with increasing chain length, but are usually larger for isobutane than n-butane because it has fewer hydrogen atoms
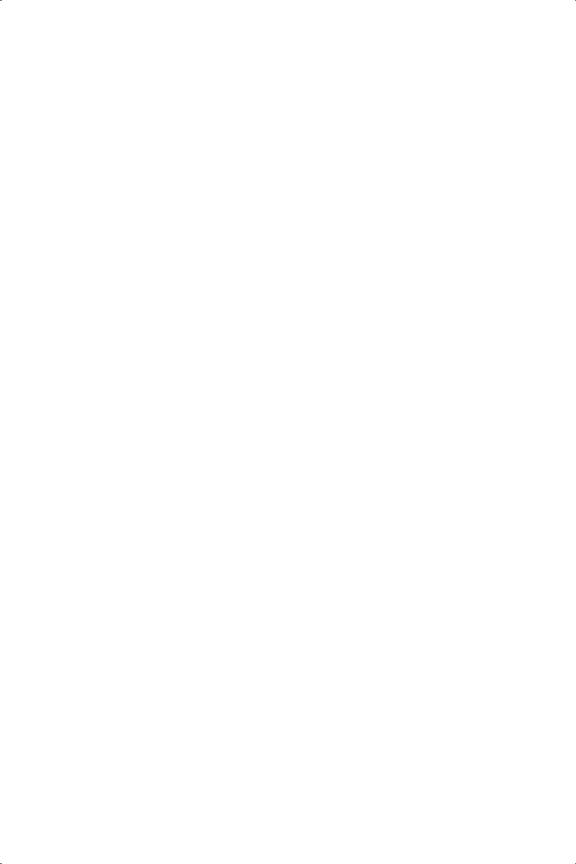
552 |
CHAPTER 13 |
that can be lost on adsorption. The correspondence between measured activation energy and hydrogen ‘orders’ thereby finds a logical explanation.
The very high activation energies encountered in alkane hydrogenolysis (50–250 kJ mol−1; even higher for neopentane) are unlike the low values found in hydrogenations (30–90 kJ mol−1) because of the endothermic nature of the preequilibrium that has to be set up before the reactive state is reached; and because their concentration is temperature-dependent, they are of necessity apparent and not true. The thermochemistry of the surface processes parallels their gas-phase counterparts, and so the ease of forming the key intermediate increases in the sequence ethane < propane < n-butane, as do their heats of dehydrogenation, which are the same as those for hydrogenation (Table 7.1) with the signs changed.
The observation that the activation energy for isomerisation Ei is greater than that for hydrogenolysis Eh is understandable72 in terms of the Temkin equation if that process requires a more dehydrogenated intermediate (Scheme 13.3, see below): the greater summation of the heats of adsorption necessitates a higher Ea. The hydrogen pressure at which rates are maximal decreases with increase in chain branching because the adsorption of the hydrocarbon becomes progressively weaker with respect to the linear molecule.72
13.2.5. Alkane Hydrogenolysis on Metals Other than Platinum
The previous section has concerned reactions on platinum almost exclusively, because of the wealth of information available, but the same modelling procedure has been used for rhodium,47 palladium,73 and especially ruthenium74−79 catalysts, whose curious behaviour will be considered further below. Palladium catalysts proved difficult to work with because they deactivated easily, and indeed the literature contains somewhat few references to work on this metal (Table 13.2 and Figure 13.3): rhodium presented no difficulties. Table 13.6 shows a selection of values of the constants of the ES5B equation (see Table 13.4) obtained by optimum curve fitting,47,64 and whatever their limitations they form a handy way of comparing the effects of varying support, chainlength, and metal. (i) With Ru/Al2O3, k and K A both increase with chainlength, but bH is almost constant. (ii) With n-butane on Rh/SiO2 and Rh/Al2O3 give similar results, but all constants are smaller with Rh/TiO2. The operation of this equation at least succeeds in separating the effects of alkane and hydrogen in generally sensible ways.
13.3.STRUCTURE-SENSITIVITY OF RATES OF ALKANE HYDROGENOLYSIS80,81
It is convenient to examine structure-sensitivity as revealed only by rates, since much of the available information concerns ethane; there are however major and important effects on product selectivities in the reaction of n-butane especially,

REACTIONS OF THE LOWER ALKANES WITH HYDROGEN |
553 |
and these will be mentioned later. There are three manifestations of structuresensitivity, which probably have elements in common, although it is wise to separate them. This section concerns (i) particle-size dependence of specific rates or TOFs, and (ii) their dependence on the face geometry of single crystals. The third aspect, namely, ensemble size and character in bimetallic systems, is also considered later.
Although alkane hydrogenolyses are usually reckoned to be structuresensitive, the evidence arising from particle-size effects is sparse and somewhat contradictory.82,83 Evaluation of the results is rendered the more difficult by the various means that have been used to alter the mean particle size, and it is hard— although perhaps not impossible84,85—to secure alteration without changing other features of the catalyst’s structure and composition.86 Techniques used include (i) changing metal loading (most often), (ii) changing the support, and (iii) changing the conditions of preparation (including precursor, calcination temperature87,88 etc.). Metal-support interactions alone can cause large effects on rates,89 so simultaneous alteration of these factors is not to be recommended. A further difficulty is that large differences in activity may necessitate use of different temperatures, so that direct comparison is not always easy.3,14,90 A selection of the available results is shown in Table 13.7; some simplification has been used in columns 3 to 6, e.g. some other supports and alkane may have been used besides those cited, so reference to the original papers is needed for full details. The following comments can however be made.
(1) A change in rate is usually accompanied by an antipathetic change in activation energy. (2) Variable results have been obtained with ruthenium (entries 3–6), due perhaps to the use of different supports and temperatures: detailed studies (see Further Reading section) to be mentioned later (Section 13.6) resolve some of these problems. (3) With rhodium, iridium and platinum the findings are inconclusive, although those for Pt/C catalysts are particularly clear; in both cases, n-butane isomerisation was size-insensitive. (4) Only with nickel is the verdict almost unanimous, the rates decreasing with size, but only above about 4 nm (entries 1–3). (5) Orders of reaction were usually not much affected by size (entries 3, 9 and 11), but the order in hydrogen became more positive with Rh/SiO2 as dispersion increased. (6) Following the argument developed in the last section, an increase in activation energy might betoken a raising of the strength of hydrogen chemisorption, and vice versa, but there are too few results to support the expected correlation with order of reaction.
Studies of the rates of hydrogenolysis of the butanes on single-crystal surfaces are similarly scarce and unsatisfactory. On various platinum surfaces at 573 K dehydrogenation is the predominant process, followed by isomerisation, with hydrogenolysis only a very minor component.91 The last process was favoured by stepped or kinked surfaces (111) structure, whereas isomerisation preferred the (100) structure, but all clean single-crystal surfaces gave much higher activities

554
TABLE 13.7. Effect of Decreasing Particle Size or Increasing Dispersion on Rates and Activation Energies of Alkane Hydrogenolysis
Entry |
Metal |
Form |
Variant |
Range |
Alkane |
rsp |
T /K |
E/kJ mol−1 |
References |
1 |
Ni |
/SiO2 -A12 O3 |
Method |
<4–8 nm |
C2 H6 |
+ |
540 |
|
209 |
|
Ni |
/A12 O3 |
[Ni], method |
2–8 nm |
C3 H8 |
Max |
600 |
min |
228 |
2 |
Ni |
/SiO2 |
[Ni] |
— |
n-C4 H10 |
+ |
473 |
+ |
107 |
3 |
Ru |
Supported |
Support, [Ru] |
0.09–72% |
C3 H8 |
|
513 |
206 |
|
4 |
Ru |
/SiO2 |
[Ru] |
16–5 nm |
n-C4 H10 |
Max |
600 |
|
220 |
5 |
Ru |
/SiO2 |
[Ru] |
2–30% |
C2 H6 |
— |
508 |
+ |
156 |
6 |
Ru |
/A12 O3 |
[Ru] |
0.07–100% |
C3 H8 |
— |
433 |
106 |
|
7 |
Ru |
/TiO2 |
Method |
24–84% |
n-C4 H10 |
+ |
433 |
— |
99,100 |
8 |
Rh |
/SiO2 |
[Rh] |
2.2–1.6 nm |
n-C4 H10 |
— |
450 |
+ |
93 |
9 |
Rh |
/TiO2 |
? |
30–85% |
C2 H6 |
+ |
573 |
— |
138 |
10 |
Rh |
/SiO2 |
[Rh], method |
12.7–4 nm |
C2 H6 |
Max |
526 |
|
203 |
11 |
Rh |
/SiO2 , A12 O3 |
[Rh] |
7.2–<1 |
n-C4 H10 |
— |
448 |
|
224 |
12 |
Ir |
/SiO2 |
[Ir] |
20–1 nm |
n-C4 H10 |
|
473 |
9 |
|
13 |
Ir |
/TiO2 |
[Ir] etc. |
10–1 nm |
n-C4 H10 |
— |
473 |
+ |
105 |
14 |
Pt |
/A12 O3 |
Method |
15–1 nm |
C2 H6 |
— |
633 |
+ |
211 |
15 |
Pt |
/C |
Method |
8–50% |
n-C4 H10 |
+ |
603 |
|
95 |
16 |
Pt |
/C |
Support, method |
6–20% |
n-C4 H10 |
+ |
583 |
|
96 |
Note: ? indicates information not given; + signifies increase; − decrease; , little change.
13 CHAPTER