
Metal-Catalysed Reactions of Hydrocarbons / 08-Hydrogenation of Alkenes and Related Processes
.pdf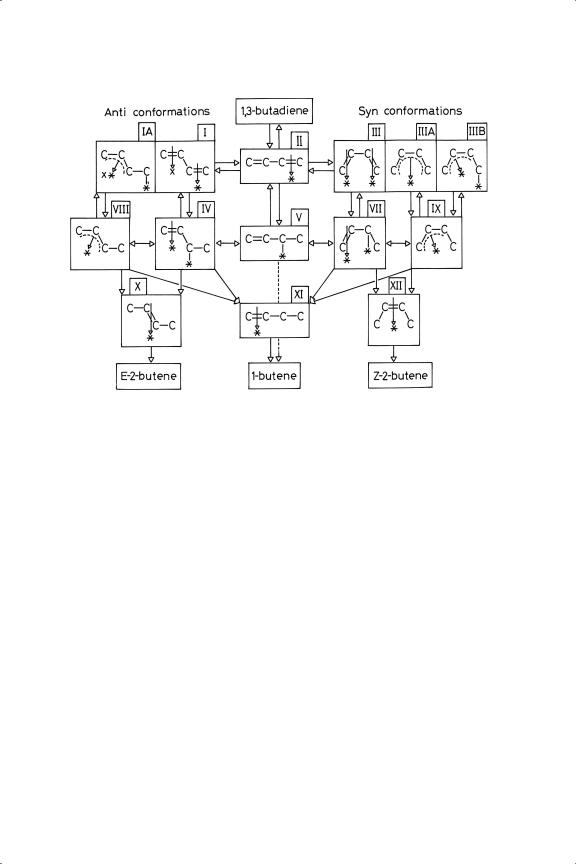
HYDROGENATION OF ALKADIENES AND POLY-ENES |
377 |
Figure 8.6. Mechanistic scheme for the hydrogenation of 1,3-butadiene.
rotation about the central C––C bond, can accomplish this. Thus adding the second hydrogen atom to an anti-π-butenyl must give E-2-butene (or 1-butene), and a syn- π -butenyl must lead to Z-2-butene (or 1-butene). The cards appear to be stacked in favour of E-2-butene and 1-butene from the outset, although a mechanism leading to Z -2-butene can be envisaged.
It is absolutely necessary to appreciate the limitations of conventional symbolism for representing the bonds between atoms in a molecule and even more so for showing bonds between adsorbed species and the metal atoms to which they are attached. Even such a simple molecule as carbon monoxide presents insuperable difficulty in this regard. Our discussion of the extensive measurements made on chemisorbed ethene (Section 4.42) have shown that a range of bonding interactions between the pure di-σ and the pure π forms is possible, the former being closely shown by platinum and the latter by palladium. These may therefore be regarded as canonical structures from which intermediate forms are constructed by mixing. The same distinction between these metals has been shown by the NEXAFS study46 referred to in Section 8.3.2. Unfortunately, no more extensive work has been done on butadiene, and in particular there are no structural studies by LEED or PED to show how the molecule might be affixed to the surface: it would be useful to have independent confirmation of the presumed prevalence of the anti conformation. In writing mechanisms, therefore, we have to remember
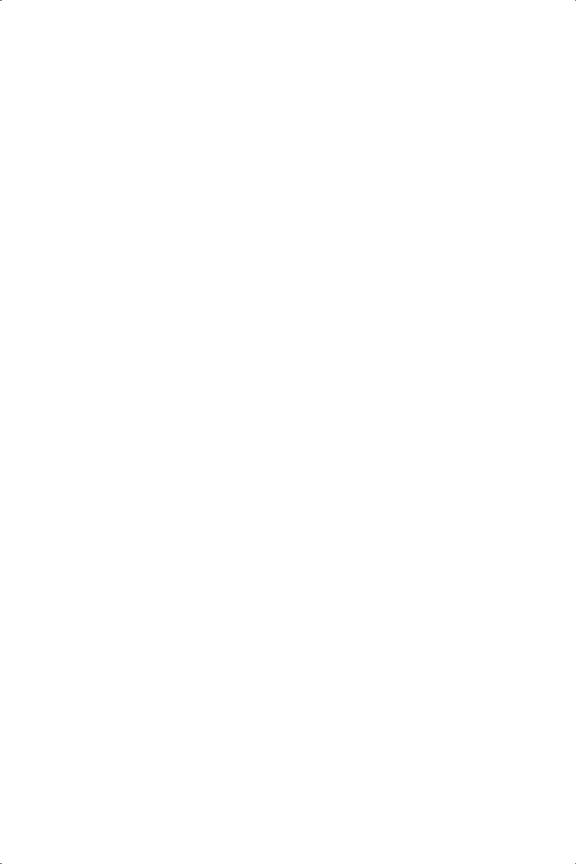
378 |
CHAPTER 8 |
that our symbolic structures only reflect the predominant character of the species in question; the truth may either be that hybrid structures exist on uniform sites, or that a range of forms between the limiting ones reside on sites of different character, depending on the coordination numbers of the contributing atoms and the presence of other adsorbed species in the neighbourhood. The literature makes frequent reference to the need to invoke different sites to explain certain features of the results,14,59 although direct evidence for their existence is rarely attainable.
Wells and his associates have devised comprehensive schemes of mechanism that embrace all features of the experimental results:10,14,22,38,59 these are combined in Figure 8.6 in a slightly different format, but retaining their numbering of the adsorbed species. On the first line are five representations of chemisorbed butadiene: on the second are shown the consequences of adding a hydrogen atom to the right-hand terminal carbon atom. Species I, III and IIIA are symmetrical, but addition to the left-hand terminal carbon atom of IA and IIIB leads respectively to E - and Z -2-butene by somewhat different routes to those shown. Addition to the second carbon atoms counting from the right leads in all cases to 1-butene; addition to an internal carbon atom in a π-butenyl or π3-butadiene will give respectively a 1,3-di-σ or a 1,3,4-tri-σ species, which may be the route by which n-butane is formed. However, in most cases Stot is high, so this last possibility is usually ignored. This scheme provides a framework within which mechanistic considerations may be debated; of course not all processes occur on all catalysts.
The full mechanism provides a plethora of routes to the observed products, and by adjustment of the positions at which hydrogen atoms are added, and the relative rates of the steps, it should be possible to account for every conceivable blend. To facilitate discussion of how the nature of the metal and the state of its surface determines the choice of elementary steps, the complete scheme has been factorised into three parts.14,54 In mechanism 1, the sequence is simply II → V → 1-butene. In mechanism 2, species I and III can equilibrate via II, and lead respectively through IV or VIII to 1-butene + E-2-butene, and through VIII or IX to 1-butene + Z -2-butene. The existence of species VIII and IX is not however an absolute requirement to account for the products. Provided the constraint that favours the anti- conformation is relaxed somewhat in the adsorbed state, and the structures I and III (and their counterparts) are of comparable stability, the occurrence of Z/E ratios of about unity can be explained. Indeed on the very simple-minded assumption that they are equally probable, and that the two ways of adding the second hydrogen atom are also equally balanced, we should obtain the three isomers in the ratio 50:25:25, which is not so far from the observed with several metals (e.g. Sc, V, Ir). The Z/E ratio is said to be fixed by the relative stabilities IV and VII, equilibrating via V: but equilibration of I and III via II will have the same effect.
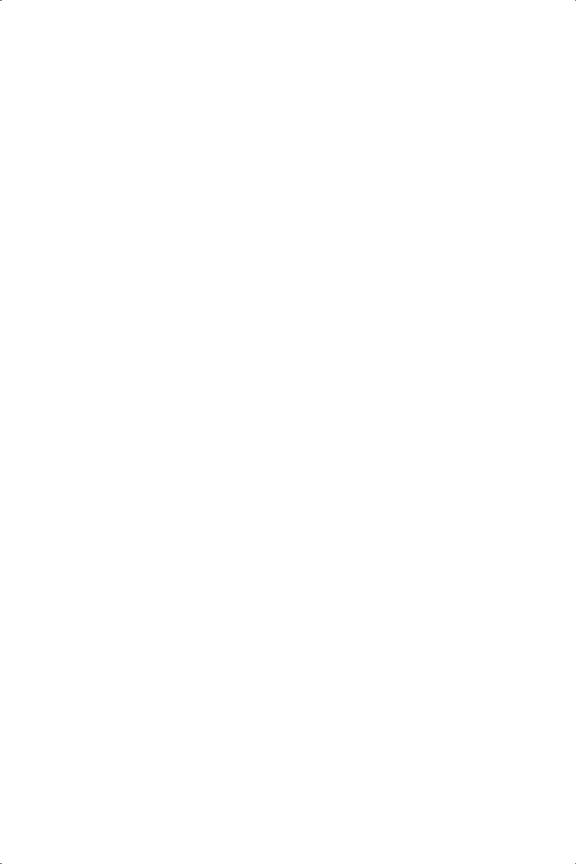
HYDROGENATION OF ALKADIENES AND POLY-ENES |
379 |
Concerning mechanisms 1 and 2, it has been suggested that there ought to be a negative correlation between S1 and the E/Z ratio; in fact, there is only an approximate connection with the pure metals.14,54 The tendency of S1 to increase as the d-band is filled is clearer, and has been assigned to a decreasing tendency to form π3 and π4 intermediates. As a generalisation this may have some truth, but there are important vertical differences as well, seen most clearly in Group 10. It may well be that the state of hybridisation of the C––M bonds in butadiene (measured by an unknown π σ factor as for ethene (Section 4.4.2)), and hence by inference in the butenyls, is a dominant factor. The simple mechanism 1 is clearly important for platinum, and for copper and manganese which have respectively filled and half-filled d-shells. The behaviour of nickel resembles that of platinum, with palladium (3d10 in the free state) as always being the odd man out: gold also resembles platinum more than silver or copper. The low Stot usually shown by platinum may have its origin in the preponderance of structure III, which from the data for heats of hydrogenation cannot be much more strongly adsorbed than the butenes.
Because of the impossibility of conformational interconversion in π3 species, if they can be formed from gaseous butadiene without the intervention of II, and if the route is then solely through VIII and IX, then we may expect a high Z/E ratio, reflecting the relative stabilities of the syn- and anti- conformations in the gas phase. But the route IA → VIII can give both 1-butene and E -2-butene, and their sum sometimes exceeds 90% and can approach the 95% expected on the basis of the gaseous stabilities (e.g. with Mn, Pd, and especially the Type B Fe, Co and Ni, see Table 8.6). Mechanism 3 therefore advocates only states in which there is a delocalised π3 bond attaching intermediates to the surface. This mechanism is pre-eminently adopted by palladium and by surfaces showing Type B characteristics: the effect of sulfur and other electronegative species is said to favour the formation of π3 and π4 species, but for reasons not entirely clear also to inhibit mechanism 1,14,54,66 which certainly contributes in the case of palladium.38 It is one of the surprising conclusions of the isotopic labelling work, which has been pursued with great diligence, that metal surfaces must exhibit heterogeneity, or at least various adsorbed species must be possible, although the overall reaction shows all the symptoms of structure-insensitivity.
8.3.6. Hydrogenation of 1, 3-Butadiene by Bimetallic Catalysts5,22,87
There have been numerous studies of this reaction catalysed by bimetallic catalysts, of which palladium has been the predominant component. The objective of much of this work has been to discover catalysts giving superior selectivity to butenes (Stot), especially in the presence of excess butenes in the reactant flow. The choice of palladium as the constant partner88 needs no explanation, as it stands out by its good activity and selectivity; nevertheless it is not perfect, and to seek to improve it further is not a waste of time (unlike gilding refined gold ).
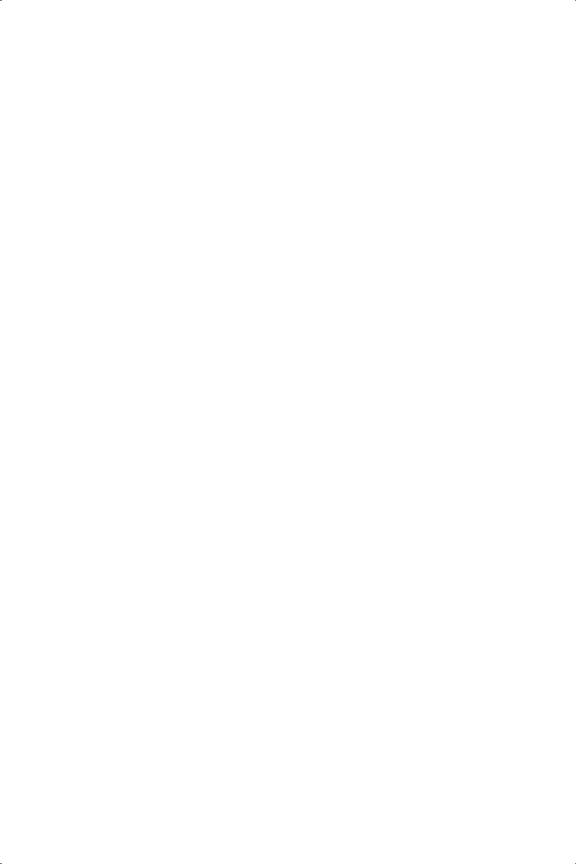
380 |
CHAPTER 8 |
Three types of work have been undertaken using (1) single crystal and polycrystalline alloys and surface alloys, (2) intermetallic compounds, and (3) conventional supported bimetallic catalysts.
An early study89 using nickel-copper films afforded Arrhenius parameters that showed good compensation over a range of activation energies from 21 to 62 kJ mol−1, corresponding to almost constant activities at the median temperature; rates were however some ten times greater for pure nickel and somewhat greater than for pure copper. This observation harmonises with what is known of the structure of nickel-copper systems made in this way (Section 1.32). Product distributions were also almost constant, but with copper the 1-butene was initially more than 90% of the whole.
There have been several studies of palladium-nickel alloys, ranging in composition from Pd1Ni9988 through Pd5Ni9588 to Pd8Ni92:90 the (111) surface of this last gives a high value of S1 (>84%). Examination of polycrystalline Pd1Ni99 and Pd5Ni95 by LEIS and XPS show surface enrichment by palladium (respectively 20 and 50%),90 the latter in particular giving faster rates than pure palladium: values of Stot were always 100%. Pd 4d binding energies in the alloys were greater than for the pure metal, due it was thought to hybridisation of the two sets of valence orbitals; shifts were small (0.1 eV) for surface atoms, but larger (0.9 eV) for those inside. The active site was considered to comprise two palladium atoms. Depositing palladium on Ni(110) and (111) at 295 K gave rates only a little faster than those for the corresponding palladium faces, but annealing at 475 K, which induced formation of a superstructure, resulted in a further 6- to 10-fold increase.52 Amorphous Pd2Ni50Nb48 ribbon became highly selective even at 100% conversion after oligomer formation; butadiene adsorbed competitively with the oligomers, but the butenes did not.69
The (111) and (110) surfaces of platinum-nickel and platinum-iron alloys have also been studied. The (111) surface of Pd50Cu50 gave rates that were very much faster than that for palladium alone.22 By evaporation of silver onto palladium deposited on amorphous silica, bimetallic particles about 3 nm in size and containing 17% silver were formed: they were about 15 times more active than palladium alone,91 and the second stage of the reaction was entirely inhibited. This is one of the few significant observations to have been made with these model systems, and suggests an important way in which selectivity in the presence of excess alkene can be achieved. ‘Microfabricated’ square or cubic particles of palladiumgold have been formed on silica,92 but butene selectivities ( 75% 1-butene, 25% E-2-butene) were independent of gold content; Arrhenius parameters showed approximate compensation. The lack of more detailed kinetic studies with these surfaces so lovingly and laboriously constructed is much to be regretted.
There have been several studies in which intermetallic compounds have been used as catalysts, but care is necessary because in some cases structural changes can occur during pre-treatment.93 Palladium-rare earth intermetallics MPd3 (M = La,
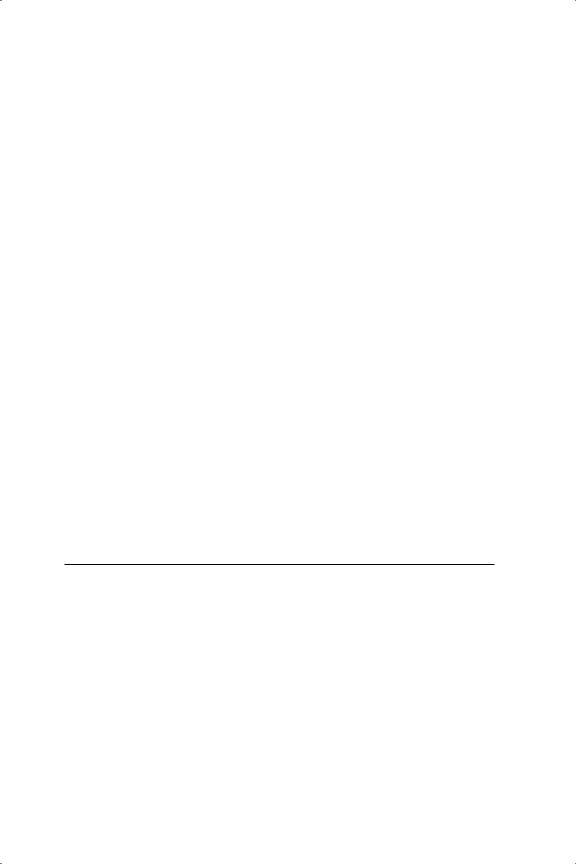
HYDROGENATION OF ALKADIENES AND POLY-ENES |
381 |
Ce, Pr, Nd, Sm) were active at 273 K, giving mainly higher values of Stot with lower values of S1 and of the E/Z ratio.93 Detailed studies have also been reported on CePd3 and ZrPd3 at higher temperatures;94 product selectivities resembled those shown by palladium sponge, but reactions stopped soon after the reactant was exhausted. With CePd3−xAux (0 < × < 1), selectivities were like those for CePd3,95 while for CeRh3−xPdx and ZrRh3−x there were complex variations that have been described in detail.96
The platinum-germanium system has six intermetallic phases, and Pt3Ge, Pt2Ge and PtGe have been examined; as the Pt/Ge ratio decreases, starting from pure platinum, the rates decreased, but Stot rose to unity; there were only minor changes in product selectivities.97 Other systems that have been studied include CoxGe, CoxSn and CoxAl.97
Available results for the hydrogenation of butadiene on supported bimetallic catalysts are surprisingly fragmentary; they are summarised in Table 8.8 for those catalysts where palladium is the major or active component. In view of the variety of methods of preparation and supports, and the general absence of surface analysis, definite conclusions are hard to detect, but it appears that addition of a Group 11 metal often improves Stot,98 and increases the conversion to which high selectivity is maintained. With palladium-silver particles formed by evaporation,99 it also inhibits the hydrogenation of excess butene; this advantage is however offset by lowered activity.68,71,79,98 The beneficial effect of Group 11 additives may thus be ascribed to a decrease in coverage by oligomer through removal of ensembles of the right size and disposition to allow their formation, while at the same time retaining small ensembles of palladium atoms that would otherwise need oligomers to create. Whether there is a loss or gain of activity therefore depends on whether lowering the number of active centres by the additive outweighs the gain by lesser oligomer
TABLE 8.8. Hydrogenation of 1,3 Butadiene: Effect of Metallic Additive on Supported Palladium Catalysts
|
|
|
|
|
Effect |
|
|
|
Additive |
Physical Form |
|
r |
Stot |
S1 |
E /Z |
Note |
References |
Cr |
Cr added to Pd/SiO2 |
|
— |
+ |
— |
— |
a |
138 |
Co |
Pd added to Co/A12 O3 |
|
— |
|
+ |
— |
|
100,131 |
Ni |
(Pd + Ni)/Nb2 O5 |
? |
? |
|
? |
|
132 |
|
Cu |
(Pd + Cu)/A12 O3 |
? |
+ |
? |
? |
b |
98,129 |
|
Ag |
(Pd + Ag)/A12 O3 |
|
— |
+ |
? |
? |
b,c |
68,71 |
Au |
(Pd + Au)/pumice |
— |
— |
|
— |
|
63 |
|
Zn |
Pd/Zn (Tred >473 K) |
|
— |
? |
+ |
? |
|
78 |
Sn |
(Pd + Sn)/A12 O3 |
? |
+ |
? |
? |
d |
139 |
a Cr added in various forms (Cr2 O7 2− , CrO4 − , Cr(CO)6 ; not all as Cr0 ). b Excess 1-butene in reactant flow.
c Unique use of 13 C-labelling to study selectivity. d Various Sn precursors used.
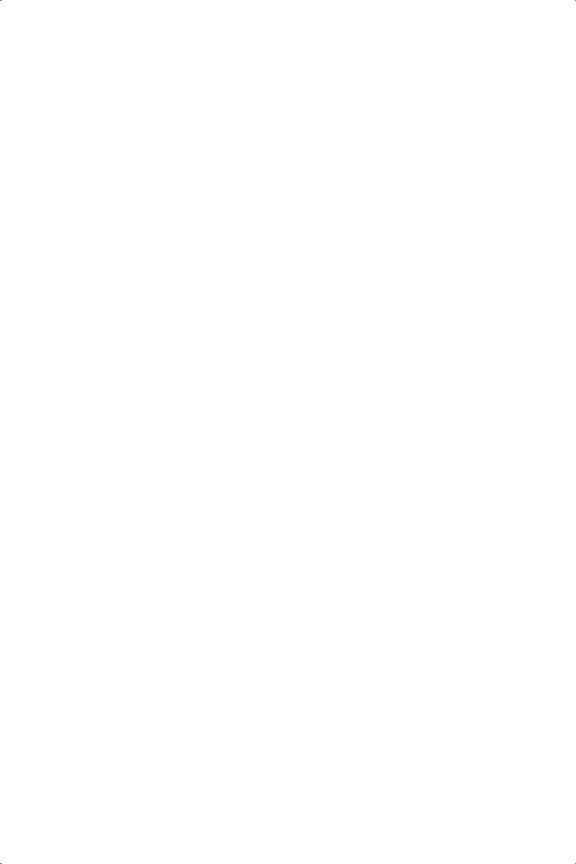
382 |
CHAPTER 8 |
formation. These effects will be seen again, and discussed further, in the following chapter. The most detailed investigation has been on the palladium-gold system,63 and this dates from 1966: initial values of Stot were above 98%, and S1 showed a maximum ( 60%) between Pd40Au60 and Pd30Au70. In this region the activation energy also passed through a maximum (62 kJ mol−1) before falling to 37 kJ mol−1 at Pd5Au95. Reaction orders were 0.7–0.9 in hydrogen and zero in butadiene. The results were discussed in great detail, and importance was attached to the dissolved hydrogen, and especially the β to α phase transition that occurred between 353 and 393 K. The butene isomer distribution characteristic of palladium (see Table 8.6) seems always to be shown by bimetallic catalyst in which palladium is a member.
The addition of tin to platinum caused a loss of activity when alumina was the support, but not when niobia was used; in this latter case, reduction at 773 K resulted in a very high value of S1 (89%).76The palladium-cobalt100 and palladiumtin101 combinations have also been examined.
8.4. HYDROGENATION OF HIGHER ALKADIENES
8.4.1. Linear Alkadienes
Extension of the linear carbon chain to five or six atoms opens up additional possible reaction paths. Higher 1,3-alkadienes are expected to behave in a similar fashion to 1,3-butadiene,102 except the two double bonds may differ in reactivity under the influence of the alkyl substituent, and their reduction naturally affords distinguishable products. Thus for example 1,3-hexadiene can give 1- and 3-hexenes by respectively 3,4- and 1,2-addition as well as 2-hexenes by 1,4-addition or isomerisation.103 A new feature in the higher 1,3- alkadienes is the existence of geometrical isomerism: Z - and E -1,3-pentadienes have been examined separately.102,104
It was mentioned in Section 8.1.1 that increasing the separation between the pair of double bonds enabled them to react more independently of each other. Comparison of the behaviours of 1,3- and 1,5-hexadienes,103,105 and the reactions of even larger α,ω-dienes,106,107 illustrates this well. With 1,2-alkadienes, their strong adsorption is a consequence of the mutual strain induced by the proximity of the double bonds, but with 1,3-dienes it is due to the simultaneous interaction of both with the surface,108 because the heat of hydrogenation of 1,3- butadiene to 1-butene is similar to that for 1-butene to butane (Table 7.1). When the separation is greater, the probability of both double bonds being accommodated by the surface is reduced, and the character of the reaction is changed. A possible complication, however, is the occurrence of double bond migration during reaction, to give the more stable conjugated 1,3-isomer, or at least an equilibrium mixture in which it predominates. However, with Pd/C catalyst, 1,5-hexadiene gave 1-hexene as the
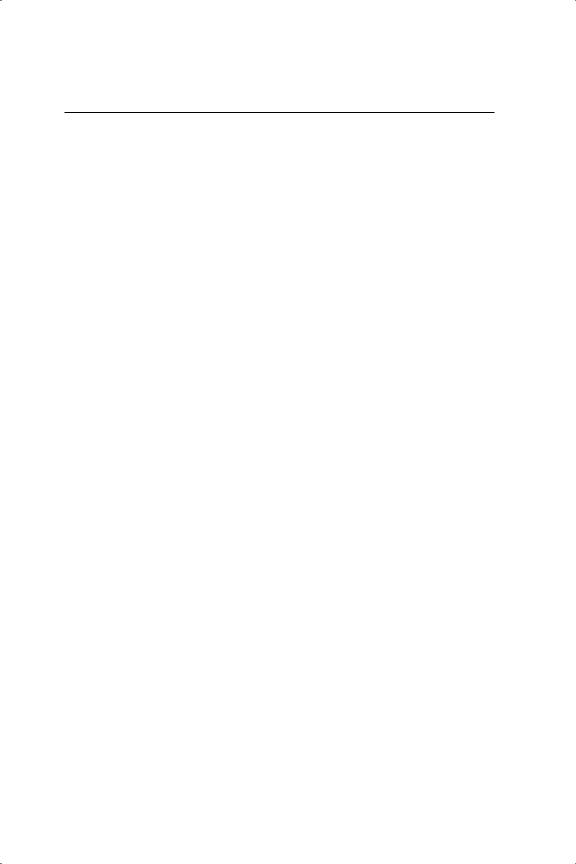
HYDROGENATION OF ALKADIENES AND POLY-ENES |
383 |
TABLE 8.9. Hydrogenation of 1,3-Pentadiene: Product Selectivities102,104
Metala |
Form |
Isomer |
Stateb |
Stot |
S1 |
SE 2 |
SZ 2 |
Co[B] |
/A12 O3 |
E |
V |
1 |
90 |
5 |
5 |
Co[B] |
/A12 O3 |
Z |
V |
1 |
88 |
6 |
6 |
Co[A] |
/A12 O3 |
E |
V |
1 |
31 |
55 |
14 |
Co[A] |
/A12 O3 |
Z |
V |
0.99 |
39 |
36 |
25 |
Ni[B] |
/A12 O3 |
Ec |
V |
1 |
29 |
60 |
11 |
Cu |
/A12 O3 |
E |
V |
1 |
69 |
19 |
12 |
Ru |
/C |
E |
L |
0.70 |
10 |
67 |
23 |
Rh |
/C |
E |
L |
0.80 |
20 |
67 |
13 |
Pd |
/C |
E |
L |
0.98 |
32 |
61 |
7 |
Pd |
/A12 O3 |
E |
V |
1 |
40 |
52 |
8 |
Pd |
/A12 O3 |
Z |
V |
1 |
38 |
44 |
18 |
Ir |
/C |
E |
L |
0.46 |
17 |
65 |
18 |
Pt |
/C |
E |
L |
0.68 |
38 |
49 |
13 |
Pt |
/A12 O3 |
Z |
V |
0.91 |
52 |
19 |
29 |
Notes: Product selectivities are those found at low conversions, but change with conversion is usually small.
a Cobalt and nickel catalysts are designated Type A or Type B, depending on reduction temperature: the latter are likely to be sulfur-contaminated.
b V, reactant as vapour; L, as liquid.
c The Z -isomer gives a similar distribution.
major initial product, followed by the E - and Z -isomers of 2-hexene, equilibrium proportions being found after 60% conversion.106
The hydrogenation of Z - and E -1,3-pentadienes has been studied in great detail on a number of metals, using the reactant as vapour,102 liquid or solution:104 a small selection of the available results is shown in Table 8.9. In the gaseous reactions, selectivities to pentenes were usually very high (but not with platinum), while in the liquid phase only palladium gave selectivities close to unity. Z-E isomerisation in the reactant was very marked with Cu/Al2O3 and Type B Co/Al2O3 giving an equilibrium mixture containing about 75% of the E -isomer at 433 K.102 Extended discussion of the mechanisms, structures and processes contributing in every case is not warranted, because the principles elaborated for the reactions of 1,3- butadiene must apply here also, with the additional effect of the methyl substituent. What is however quite clear is that 3,4-addition is favoured over the other modes, so that the methyl group must, by an electronic effect, cause greater reactivity in the adjacent double bond, and not an inhibiting effect due to steric interference.102 It is however possible to chart the reaction paths assuming the intervention of π4 or π3σ alkenylic intermediates (Figure 8.7).
The four possible orientations of the two reactants are shown, and they are then represented as the possible π3σ structures (π4 structures are only possible in the syn conformations). We then assume that in forming the alkene the σ C––M bond is first broken and the second hydrogen atom is added at one or other end of the delocalised bond. Assuming values for the relative stabilities of syn and anti conformers, and

384 |
CHAPTER 8 |
Figure 8.7. Syn and anti conformers of Z - and E -1,3-pentadiene, and their representation as alternative π3 σ structures.
for the effects of alkyl groups on the point of addition of the second hydrogen, product distributions can be calculated. It is important to minimise the number of arbitrary assumptions, and to keep assumed values within reasonable limits. The results shown in Table 8.10 take the ratio of anti:syn conformers to be four, and the positive weighting to the 3,4-addition mode to be 1.5 or 2.0. Good agreement is obtained with experimental results for the reaction of E -1,3-pentadiene over palladium catalysts, but it is not so good for the Z -isomer, which probably is partly isomerised during the reaction. To support this view, the calculation for the equilibrium mixture (Z , 83; E , 17) agrees quite well with observation. The two isomers interconverted in the absence of hydrogen on cobalt catalysts.109
The reactions of 1,3- and 1,5-hexadienes with hydrogen have been carried out with Pd/Al2O3 catalysts of various dispersions; the reaction was not structuresensitive nor were product distributions affected.103 With the 1,3-isomer, selectivities were very high until at least 95% conversion, and product selectivities were not greatly conversion-dependant. Representative values are shown in Table 8.11. The Z/E ratio of the reactant was not specified, so that model calculations cannot be made with certainty, but the predominance of Z-isomers in the product argues for the preference for anti-conformations in the adsorbed states. Pd5.2Sn0.8/Al2O3
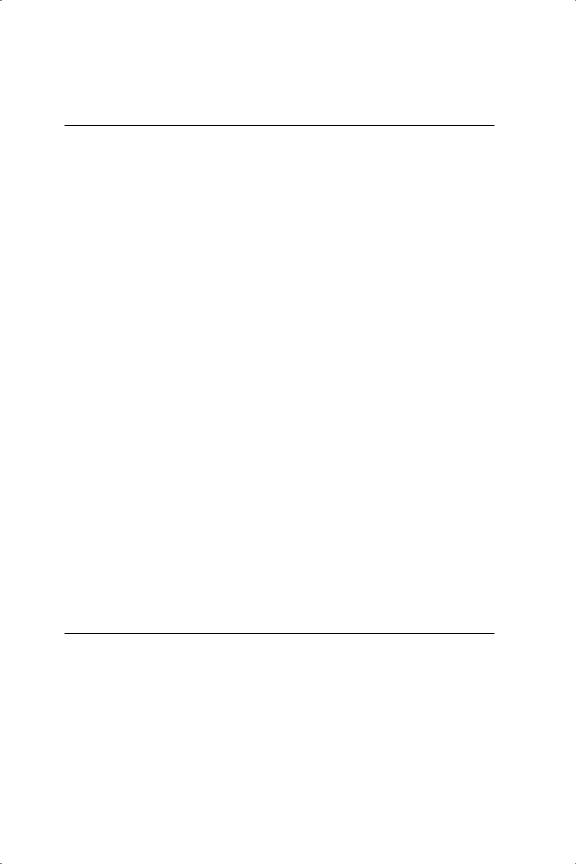
HYDROGENATION OF ALKADIENES AND POLY-ENES |
385 |
TABLE 8.10. Comparison of Calculated and Observed Product Selectivities in the Hydrogenation of 1,3-Pentadiene on Palladium Catalysts102,104
Isomer |
a/sb |
f3,4 c |
S1 |
SE 2 |
SZ 2 |
E |
4 |
1 |
25 |
65 |
10 |
E |
4 |
1.5 |
33 |
58 |
9 |
E |
— |
— |
32 |
61 |
7 |
E |
4 |
2 |
40 |
52 |
8 |
E |
— |
— |
40 |
52 |
8 |
Z |
4 |
1 |
25 |
40 |
35 |
Z |
4 |
1.5 |
33 |
36 |
31 |
Z |
— |
— |
39 |
40 |
22 |
a |
|
|
|
|
|
(Z + E )eqa |
4 |
1.5 |
33 |
54 |
13 |
(Z + E )eq |
— |
— |
38 |
51 |
11 |
Notes: aAssumed to have Z /E =17:83. bAssumed ratio of anti:syn conformers. cAssumed weighting of the 3,4-addition mode.
gave similar results. With 1,5-hexadiene, however, hydrogenation over Pd/Al2O3 and catalysts also containing tin or silver, total selectivity fell progressively with conversion, and product selectivities moved in the direction of equilibrium concentrations, indicative the relatively weaker adsorption of this reactant. The more extensive formation of the 2-hexenes reflects the occurrence of double-bond migration before desorption.
1,7-Octadiene on a palladium catalyst was hydrogenated at 323 K to a mixture of octenes and isomerised to other octadienes, but Stot was somewhat low.110
Pt/TiO2 hydrogenated 1,3-hexadiene with a total selectivity of 90%, with a broad mix of other products (see Table 8.4) but when deposited on a ceramic membrane the product was 100% 1-hexene;111 the suggestion that this was due to lack of back-mixing implies that re-adsorption of 1-hexene was somehow prevented, but this does not normally take place, and a full explanation still needs to be found.
TABLE 8.11. Product Selectivities for the Hydrogenation of Hexadienes on A12 O3 - (or TiO2 )- Supported Catalysts103,111
Metal |
Isomer |
S1 |
SE 2 |
SZ 2 |
SE 3 |
SZ 3 |
Note |
Pd |
1,3- |
35 |
31 |
3 |
31 |
0 |
|
Pd3.8 Ag5.2 |
1,3- |
27 |
31 |
5 |
35 |
0 |
a |
Pd |
1,5- |
30 |
26 |
12 |
6 |
26 |
|
Pd5.2 Sn0.8 |
1,5- |
48 |
18 |
10 |
10 |
12 |
|
Pt |
1,3- |
29 |
29 |
2 |
29 |
11 |
b |
|
|
|
|
|
|
|
|
a This is the distribution at 90% conversion: the others are approximate values obtained by extrapolating graphical results to zero conversion.
b TiO2 support (363 K).

386 |
CHAPTER 8 |
8.4.2. Branched Alkadienes44
Isoprene (2-methyl-1,3-butadiene) is the precursor to a great range of naturally occurring molecules, including terpenes, diand triterpenes, and steroids, the skeletons of which are composed of branched C5 units: it is also of course the origin of natural rubber. The addition of a methyl group at C2 to butadiene creates a molecule the hydrogenation of which has certain interesting features, because 1,2- and 3,4-additions are now distinguishable, and the products are 2-methyl-2- butene, 3-methyl-1-butene and 2-methyl-1-buene. No geometrical isomerism is possible in this system.
The reaction has been studied mainly on palladium catalysts of various types,110,112−116 including the bimetallic Pd-Ag/SiO2 and Pd-Au/SiO2 systems.115 Values of Stot were generally high (>0.96), but the conversion-independent product distributions varied slightly but significantly with composition, in an interesting manner (Table 8.12). Once again we may chart the progress of the reaction in terms of π3σ and π3 intermediates (Figure 8.8). The two half-hydrogenated π3 states differ in the disposition of the methyl substituents, and if they have no effect on the chances of adding the final hydrogen atom to the termini of the delocalised bond, the consequence will be
[3,1] : [2,2] : [2,1] = 1 : 2 : 1 |
(8.1) |
This ratio is indeed close to that often found115 (Table 8.12), but close examination has revealed small variations. These have been attributed to the different effects of the electron-releasing methyl groups on the locus of the addition of the final hydrogen atom. If the carbon atom carrying n methyls is nf times more able to accept a hydrogen atom than that having none, the ratio then becomes
[3,1] : [2,2] : [2,1] = (1 + 2 f ) : 2 : (1 + f ) |
(8.2) |
TABLE 8.12. Hydrogenation of Isoprene (2-Methyl-1,2-Butadiene): Product Selectivities Obtained with Palladium Catalysts,110,113,115 and Matching Calculations115,117
Form/Composition |
Stot |
S22 |
S31 |
S21 |
f |
/BaSO4 |
>95 |
45.4 |
29.9 |
24.7 |
— |
|
|
44.9 |
29.2 |
25.8 |
0.15 |
/SiO2 |
>95 |
49.1 |
26.8 |
24.1 |
— |
|
|
48.5 |
26.2 |
25.2 |
0.04 |
Pd20 Ag80 //SiO2 |
98 |
42.3 |
30.5 |
27.2 |
(0.225) |
Pd20 Au80 //SiO2 |
97 |
48.2 |
25.5 |
26.3 |
(0.05) |
/C+ 3 Bi |
86 |
56.6 |
20.0 |
23.5 |
— |
/δ-A12 O3 |
|
56.0 |
20.0 |
24.0 |
−0.14 |
— |
78 |
15 |
7 |
(−0.48) |
Note: Three examples of matching calculations are shown; values of f in brackets give approximate fits to the experimental distributions shown.