
- •Textbook Series
- •Contents
- •1 Overview and Definitions
- •Overview
- •General Definitions
- •Glossary
- •List of Symbols
- •Greek Symbols
- •Others
- •Self-assessment Questions
- •Answers
- •2 The Atmosphere
- •Introduction
- •The Physical Properties of Air
- •Static Pressure
- •Temperature
- •Air Density
- •International Standard Atmosphere (ISA)
- •Dynamic Pressure
- •Key Facts
- •Measuring Dynamic Pressure
- •Relationships between Airspeeds
- •Airspeed
- •Errors and Corrections
- •V Speeds
- •Summary
- •Questions
- •Answers
- •3 Basic Aerodynamic Theory
- •The Principle of Continuity
- •Bernoulli’s Theorem
- •Streamlines and the Streamtube
- •Summary
- •Questions
- •Answers
- •4 Subsonic Airflow
- •Aerofoil Terminology
- •Basics about Airflow
- •Two Dimensional Airflow
- •Summary
- •Questions
- •Answers
- •5 Lift
- •Aerodynamic Force Coefficient
- •The Basic Lift Equation
- •Review:
- •The Lift Curve
- •Interpretation of the Lift Curve
- •Density Altitude
- •Aerofoil Section Lift Characteristics
- •Introduction to Drag Characteristics
- •Lift/Drag Ratio
- •Effect of Aircraft Weight on Minimum Flight Speed
- •Condition of the Surface
- •Flight at High Lift Conditions
- •Three Dimensional Airflow
- •Wing Terminology
- •Wing Tip Vortices
- •Wake Turbulence: (Ref: AIC P 072/2010)
- •Ground Effect
- •Conclusion
- •Summary
- •Answers from page 77
- •Answers from page 78
- •Questions
- •Answers
- •6 Drag
- •Introduction
- •Parasite Drag
- •Induced Drag
- •Methods of Reducing Induced Drag
- •Effect of Lift on Parasite Drag
- •Aeroplane Total Drag
- •The Effect of Aircraft Gross Weight on Total Drag
- •The Effect of Altitude on Total Drag
- •The Effect of Configuration on Total Drag
- •Speed Stability
- •Power Required (Introduction)
- •Summary
- •Questions
- •Annex C
- •Answers
- •7 Stalling
- •Introduction
- •Cause of the Stall
- •The Lift Curve
- •Stall Recovery
- •Aircraft Behaviour Close to the Stall
- •Use of Flight Controls Close to the Stall
- •Stall Recognition
- •Stall Speed
- •Stall Warning
- •Artificial Stall Warning Devices
- •Basic Stall Requirements (EASA and FAR)
- •Wing Design Characteristics
- •The Effect of Aerofoil Section
- •The Effect of Wing Planform
- •Key Facts 1
- •Super Stall (Deep Stall)
- •Factors that Affect Stall Speed
- •1g Stall Speed
- •Effect of Weight Change on Stall Speed
- •Composition and Resolution of Forces
- •Using Trigonometry to Resolve Forces
- •Lift Increase in a Level Turn
- •Effect of Load Factor on Stall Speed
- •Effect of High Lift Devices on Stall Speed
- •Effect of CG Position on Stall Speed
- •Effect of Landing Gear on the Stall Speed
- •Effect of Engine Power on Stall Speed
- •Effect of Mach Number (Compressibility) on Stall Speed
- •Effect of Wing Contamination on Stall Speed
- •Warning to the Pilot of Icing-induced Stalls
- •Stabilizer Stall Due to Ice
- •Effect of Heavy Rain on Stall Speed
- •Stall and Recovery Characteristics of Canards
- •Spinning
- •Primary Causes of a Spin
- •Phases of a Spin
- •The Effect of Mass and Balance on Spins
- •Spin Recovery
- •Special Phenomena of Stall
- •High Speed Buffet (Shock Stall)
- •Answers to Questions on Page 173
- •Key Facts 2
- •Questions
- •Key Facts 1 (Completed)
- •Key Facts 2 (Completed)
- •Answers
- •8 High Lift Devices
- •Purpose of High Lift Devices
- •Take-off and Landing Speeds
- •Augmentation
- •Flaps
- •Trailing Edge Flaps
- •Plain Flap
- •Split Flap
- •Slotted and Multiple Slotted Flaps
- •The Fowler Flap
- •Comparison of Trailing Edge Flaps
- •and Stalling Angle
- •Drag
- •Lift / Drag Ratio
- •Pitching Moment
- •Centre of Pressure Movement
- •Change of Downwash
- •Overall Pitch Change
- •Aircraft Attitude with Flaps Lowered
- •Leading Edge High Lift Devices
- •Leading Edge Flaps
- •Effect of Leading Edge Flaps on Lift
- •Leading Edge Slots
- •Leading Edge Slat
- •Automatic Slots
- •Disadvantages of the Slot
- •Drag and Pitching Moment of Leading Edge Devices
- •Trailing Edge Plus Leading Edge Devices
- •Sequence of Operation
- •Asymmetry of High Lift Devices
- •Flap Load Relief System
- •Choice of Flap Setting for Take-off, Climb and Landing
- •Management of High Lift Devices
- •Flap Extension Prior to Landing
- •Questions
- •Annexes
- •Answers
- •9 Airframe Contamination
- •Introduction
- •Types of Contamination
- •Effect of Frost and Ice on the Aircraft
- •Effect on Instruments
- •Effect on Controls
- •Water Contamination
- •Airframe Aging
- •Questions
- •Answers
- •10 Stability and Control
- •Introduction
- •Static Stability
- •Aeroplane Reference Axes
- •Static Longitudinal Stability
- •Neutral Point
- •Static Margin
- •Trim and Controllability
- •Key Facts 1
- •Graphic Presentation of Static Longitudinal Stability
- •Contribution of the Component Surfaces
- •Power-off Stability
- •Effect of CG Position
- •Power Effects
- •High Lift Devices
- •Control Force Stability
- •Manoeuvre Stability
- •Stick Force Per ‘g’
- •Tailoring Control Forces
- •Longitudinal Control
- •Manoeuvring Control Requirement
- •Take-off Control Requirement
- •Landing Control Requirement
- •Dynamic Stability
- •Longitudinal Dynamic Stability
- •Long Period Oscillation (Phugoid)
- •Short Period Oscillation
- •Directional Stability and Control
- •Sideslip Angle
- •Static Directional Stability
- •Contribution of the Aeroplane Components.
- •Lateral Stability and Control
- •Static Lateral Stability
- •Contribution of the Aeroplane Components
- •Lateral Dynamic Effects
- •Spiral Divergence
- •Dutch Roll
- •Pilot Induced Oscillation (PIO)
- •High Mach Numbers
- •Mach Trim
- •Key Facts 2
- •Summary
- •Questions
- •Key Facts 1 (Completed)
- •Key Facts 2 (Completed)
- •Answers
- •11 Controls
- •Introduction
- •Hinge Moments
- •Control Balancing
- •Mass Balance
- •Longitudinal Control
- •Lateral Control
- •Speed Brakes
- •Directional Control
- •Secondary Effects of Controls
- •Trimming
- •Questions
- •Answers
- •12 Flight Mechanics
- •Introduction
- •Straight Horizontal Steady Flight
- •Tailplane and Elevator
- •Balance of Forces
- •Straight Steady Climb
- •Climb Angle
- •Effect of Weight, Altitude and Temperature.
- •Power-on Descent
- •Emergency Descent
- •Glide
- •Rate of Descent in the Glide
- •Turning
- •Flight with Asymmetric Thrust
- •Summary of Minimum Control Speeds
- •Questions
- •Answers
- •13 High Speed Flight
- •Introduction
- •Speed of Sound
- •Mach Number
- •Effect on Mach Number of Climbing at a Constant IAS
- •Variation of TAS with Altitude at a Constant Mach Number
- •Influence of Temperature on Mach Number at a Constant Flight Level and IAS
- •Subdivisions of Aerodynamic Flow
- •Propagation of Pressure Waves
- •Normal Shock Waves
- •Critical Mach Number
- •Pressure Distribution at Transonic Mach Numbers
- •Properties of a Normal Shock Wave
- •Oblique Shock Waves
- •Effects of Shock Wave Formation
- •Buffet
- •Factors Which Affect the Buffet Boundaries
- •The Buffet Margin
- •Use of the Buffet Onset Chart
- •Delaying or Reducing the Effects of Compressibility
- •Aerodynamic Heating
- •Mach Angle
- •Mach Cone
- •Area (Zone) of Influence
- •Bow Wave
- •Expansion Waves
- •Sonic Bang
- •Methods of Improving Control at Transonic Speeds
- •Questions
- •Answers
- •14 Limitations
- •Operating Limit Speeds
- •Loads and Safety Factors
- •Loads on the Structure
- •Load Factor
- •Boundary
- •Design Manoeuvring Speed, V
- •Effect of Altitude on V
- •Effect of Aircraft Weight on V
- •Design Cruising Speed V
- •Design Dive Speed V
- •Negative Load Factors
- •The Negative Stall
- •Manoeuvre Boundaries
- •Operational Speed Limits
- •Gust Loads
- •Effect of a Vertical Gust on the Load Factor
- •Effect of the Gust on Stalling
- •Operational Rough-air Speed (V
- •Landing Gear Speed Limitations
- •Flap Speed Limit
- •Aeroelasticity (Aeroelastic Coupling)
- •Flutter
- •Control Surface Flutter
- •Aileron Reversal
- •Questions
- •Answers
- •15 Windshear
- •Introduction (Ref: AIC 84/2008)
- •Microburst
- •Windshear Encounter during Approach
- •Effects of Windshear
- •“Typical” Recovery from Windshear
- •Windshear Reporting
- •Visual Clues
- •Conclusions
- •Questions
- •Answers
- •16 Propellers
- •Introduction
- •Definitions
- •Aerodynamic Forces on the Propeller
- •Thrust
- •Centrifugal Twisting Moment (CTM)
- •Propeller Efficiency
- •Variable Pitch Propellers
- •Power Absorption
- •Moments and Forces Generated by a Propeller
- •Effect of Atmospheric Conditions
- •Questions
- •Answers
- •17 Revision Questions
- •Questions
- •Answers
- •Explanations to Specimen Questions
- •Specimen Examination Paper
- •Answers to Specimen Exam Paper
- •Explanations to Specimen Exam Paper
- •18 Index

7 Stalling
Stalling 7
Effect of CG Position on Stall Speed
CS-25.103(b) states that VCLMAX is determined with the CG position that results in the highest value of reference stall speed.
L
CP
TAIL
DOWNLOAD
W
Figure 7.24
If the CG is in front of the CP, Figure 7.24, giving a nose-down pitching moment and there is no thrust/drag moment to oppose it, the tailplane must provide a down load to maintain equilibrium. Lift must be increased to maintain an upwards force equal to the increased
downwards force. From the 1g stall formula it can be seen that CLMAX will be divisible into the increased lift force more times.
|
|
√ |
|
|
|
VS1g |
= |
|
L |
|
|
|
|
|
|||
|
|
½ ρ CLMAX |
S |
||
|
|
|
|
Forward movement of the CG increases stall speed.
174

Stalling 7
Effect of Landing Gear on the Stall Speed
L
CP
TAIL
DOWNLOAD
PROFILE DRAG
FROM GEAR
W
Figure 7.25
From Figure 7.25 it can be seen that with the undercarriage down, profile drag below the CG is increased. This will give a nose-down pitching moment which must be balanced by increasing the tail down load. Lift must be increased to balance the increased downwards force.
CG movement due to the direction in which the undercarriage extends will have an insignificant influence on stall speed. By far the greater influence is the increased profile drag of the gear when it is extended.
Extending the undercarriage increases stall speed.
Effect of Engine Power on Stall Speed
CS-25.103(b) states that VCLMAX is determined with zero thrust at the stall speed.
When establishing VCLMAX the engines must be at zero thrust and it is assumed that the weight of the aircraft is entirely supported by lift. If thrust is applied close to the stall, the nose high
attitude of the aircraft produces a vertical component of thrust, Figure 7.27, which assists in supporting the weight and less lift is required. Aircraft with propellers will have an additional effect caused by the propeller slipstream.
The most important factors affecting this relationship are engine type (propeller or jet), thrust to weight ratio and inclination of the thrust vector at CLMAX.
Stalling 7
175
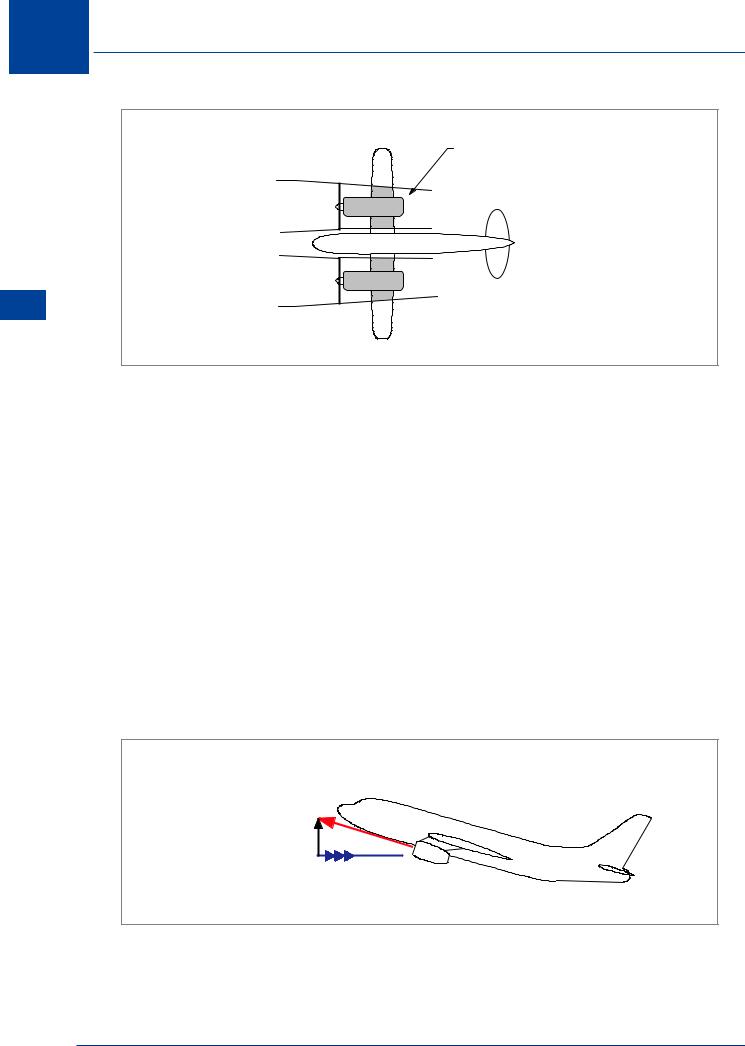
7 Stalling
INDUCED FLOW
FROM PROPELLER
SLIPSTREAM
Stalling 7
Figure 7.26
Propeller
Figure 7.26. The slipstream velocity behind the propeller is greater than the free stream flow, depending on the thrust developed. Thus, when the propeller aeroplane is at low airspeeds and high power, the dynamic pressure within the propeller slipstream is much greater than that outside and this generates much more lift than at zero thrust. The lift of the aeroplane at a given angle of attack and airspeed will be greatly affected. If the aircraft is in the landing flare, reducing power suddenly will cause a significant reduction in lift and a heavy landing could result. On the other hand, a potentially heavy landing can be avoided by a judicious ‘blast’ from the engines.
Jet
The typical jet aircraft does not experience the induced flow velocities encountered in propeller driven aeroplanes, thus the only significant factor is the vertical component of thrust, Figure 7.27. Since this vertical component contributes to supporting the weight of the aircraft, less aerodynamic lift is required to hold the aeroplane in flight. If the thrust is large and is given a large inclination at maximum lift angle, the effect on stall speed can be very large. Since there is very little induced flow from the jet, the angle of attack at stall is essentially the same poweron as power-off.
VERTICAL
COMPONENT
OF THRUST
Figure 7.27
Power-on stall speed is less than power-off. This will be shown to be significant during the study of windshear in Chapter 15.
176

Stalling 7
Effect of Mach Number (Compressibility) on Stall Speed
As an aircraft flies faster, the streamline pattern around the wing changes. Faster than about four tenths the speed of sound (M 0.4) these changes start to become significant. This phenomena is known as compressibility. This will be discussed fully in Chapter 13.
Pressure waves, generated by the passage of a wing through the air, propagate ahead of the wing at the speed of sound. These pressure waves upwash air ahead of the wing towards the lower pressure on the top surface.
Stalling 7
HIGH SPEED
LOW SPEED
Figure 7.28
Figure 7.28 shows that at low speed, the streamline pattern is affected far ahead of the wing and the air has a certain distance in which to upwash. As speed increases, the wing gets closer to its leading pressure wave, and the streamline pattern is affected a shorter distance ahead so must approach the wing at a steeper angle.
This change in the streamline pattern accentuates the adverse pressure gradient near the leading edge and flow separation occurs at a reduced angle of attack. Above M0.4 CLMAX decreases as shown in Figure 7.29.
CLMAX |
|
|
0 4 |
1 0 |
M |
|
|
|
|
Figure 7.29 |
|
177

7 Stalling
Stalling 7
Referring to the 1g stall speed formula:
|
|
√ |
|
|
|
VS1g |
= |
|
|
L |
|
|
|
|
|||
|
|
½ |
ρ CLMAX S |
||
|
|
|
|
||
If CLMAX decreases, VS1g will increase. |
|
|
|
|
|
To maintain a constant EAS as altitude increases, TAS is increased. Also, outside air temperature decreases with increasing altitude, causing the local speed of sound to decrease. Mach number is proportional to TAS and inversely proportional to the local speed of sound (a):
TAS
M = a
Therefore, at a constant EAS, Mach number will increase as altitude increases.
Alt
1g Stall Speed
EAS
Figure 7.30
Figure 7.30 shows the variation of stalling speed with altitude at constant load factor (n). Such a curve is called the stalling boundary for the given load factor, in which altitude is plotted against equivalent airspeed. At this load factor (1g), the aircraft cannot fly at speeds to the left of this boundary. It is clear that over the lower range of altitude, stall speed does not vary with altitude. This is because at these low altitudes, the Mach number at VS is less than M 0.4, too low for compressibility effects to be present. Eventually (approximately 30 000 ft), Mach number at VS has increased with altitude to such an extent that these effects are important, and the rise in stalling speed with altitude is apparent.
Using the example aeroplane from earlier, the VS1g of 150 kt is equal to M 0.4 at approximately 29 000 ft using ISA values.
As altitude increases, stall speed is initially constant then increases, due to compressibility.
178