
Astruc D. - Modern arene chemistry (2002)(en)
.pdf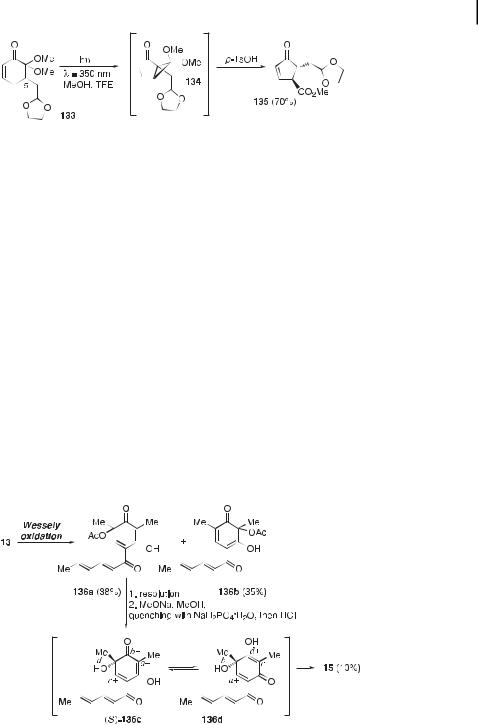

564 15 Oxidative Conversion of Arenols into ortho-Quinols and ortho-Quinone Monoketals
Fig. 35
A mechanistic description of this [4þ4] dimerization implies the participation of 136c and its cyclohexa-2,5-dienone tautomer 136d. An initial intermolecular Michael addition (connectivity a) is followed by a first ketalization (b), an intramolecular Michael addition (c), and a second and final ketalization (d). Nicolaou and co-workers utilized a similar approach in their synthesis of bisorbicillinoids [40, 171, 173]. The Wessely oxidation was also used to dearomatize 13 to give 136a, which was then slowly dimerized in the presence of CsOH H2O in MeOH to furnish 15 in 16 % yield, together with bisorbicillinol (19) in 22 % yield (Figure 6). Better yields of the [4þ2] endo-cycloadduct 19 were obtained by treating 136a with either solid KOH in aqueous THF or with concentrated HCl in THF (Figure 35). A rather intriguing observation was that the ortho-quinol acetate 136a did not undergo any dimerization, even when heated in benzene or acetic acid for several hours. Deacetylation and acidification of the reaction medium are required to unleash the reactivity of the quinolic system (Section 15.3.1).
ortho-Quinone monoketals and, in particular, ortho-quinol acetates continue to demonstrate their utility in biaryl synthesis [6]. Hoshino has shown that various N-acyl and N- methanesulfonyl-1,2,3,4-tetrahydro-7-methoxyisoquinolin-6-ols 137a can be transformed into noraporphine 139a and phenanthrene 140a derivatives following their conversion to the corresponding ortho-quinol acetates 138a through an apparent vinylogous SN20-type substitution at their C-2 center (Figure 36) [174]. In contrast, regioisomeric 6-methoxyisoquinolin-7- ols 137b prefer to undergo 1,3-acetate shifts to furnish cyclohexa-2,5-dienone derivatives 138c, which can then undergo ring-opening rearomatization to give 140b. Therefore, an electron-releasing alkyl group on the isoquinoline nitrogen (e.g. R ¼ Me) appears to be necessary to direct the system toward biaryl bond formation by acid-catalyzed Michael-type 1,6- addition at the C-5 center of 138b to furnish 139b [174].
In unbiased systems reacting in an intermolecular fashion, ortho-quinone monoketals usually behave as Michael 1,4-acceptors. This reactivity feature has been exploited for the rapid and convergent synthesis of fused aromatic polycycles and their quinonoid derivatives. Anionic annulation of phthalides with ortho-quinone monoketals was first proposed by Mitchell and Russell for the preparation of linearly fused anthracyclines [175, 176]. Mal and co-workers applied this strategy to the synthesis of benz[a]anthraquinone motifs 145a–d present in angucycline natural products (Figure 37). Dimethyl ketals 142a–c were generated by means of Pelter oxidation [177].


566 15 Oxidative Conversion of Arenols into ortho-Quinols and ortho-Quinone Monoketals
Fig. 38
Regioselective heteroatom–carbon bond formation is another item in the panoply of reactions available with ortho-quinol derivatives. The general trends of their electrophilic behavior with respect to both hard and soft heteroatomas well as carbon-based nucleophiles have been summarized previously [6]. Epoxidation of their double bonds is another example of a heteroatom–carbon bond-forming process. Analogues of scyphostatin (20, Figure 7) have been prepared by epoxidation of 147, which was derived from 4-bromoguaiacol (146) by Pelter oxidation; the bromide substitution e ect first described by Liao and co-workers (Section 15.3.1) was judiciously exploited to prepare an otherwise unstable ortho-quinone monoketal (Figure 38) [44, 126].
Our synthetic studies using ortho-quinol acetates have also taken us into the realm of carbon–heteroatom bond formation and notably concern regioselective benzannulation reactions. Intramolecular conjugate 1,4-additions to the enone system of ortho-quinol acetates were carried out to build benzannulated fiveto seven-membered ether rings, indoles, quinolines, and their oxo derivatives (Figure 39) [112, 113, 178]. The starting 2-methox- yphenols 151a–c and 154a–c were tethered with a protected nucleophilic center and submitted to PIDA-mediated oxidative acetoxylation to furnish the acetates 152a–c and 155a–c in excellent yields. The silyl-protected ortho-quinols 152a–c and 155a,b were then treated with a source of fluoride ions (i.e. TBAF ¼ tetrabutylammonium fluoride) to induce cyclization, which was followed by aromatization with concomitant loss of the 6-acetoxy group. The cyclization of N-benzylated acetates such as 155c was promoted by the action of a base (e.g. potassium tert-butoxide in THF). All observed cyclizations followed an exo-trig mode.
Applications of this benzannulation tactic can be anticipated in the synthesis of polyoxygenated polycyclic alkaloids and heterocyclic terpenoids. Work is currently in progress in our laboratory aimed at constructing the polyoxygenated tetracyclic core of Amaryllidaceae alkaloids such as lycorine (157). The stable bis(ortho-quinol acetate) Tsoc-protected secondary amine 159 has been subjected to the TBAF-mediated deprotection–cyclization conditions in the hope of inducing the formation of both the strategic NaC and CaC bonds in a domino fashion by taking advantage of an electrophile–nucleophile switch of reactivity of the eastern ortho-quinol moiety (Figure 40). The heterocyclization occurred, but led only to the indole product 160 [178].
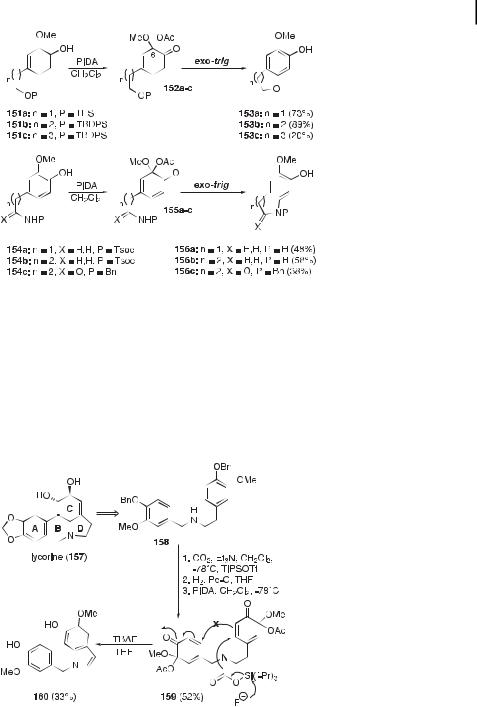

568 15 Oxidative Conversion of Arenols into ortho-Quinols and ortho-Quinone Monoketals
Fig. 41
15.4
Conclusion
The prime objective of writing this chapter was to demonstrate that the oxidative conversion of simple 2-substituted hydroxylated aromatic molecules into ortho-quinone monoketals or other ortho-quinol derivatives constitutes a powerful tactic for the rapid elaboration of structural complexity and diversity in organic synthesis. The concepts of this tactic are certainly well anchored in fifty years of research in the field of phenol oxidation chemistry, but its synthetic applications have long been thwarted by the di culties encountered in working with highly reactive ortho-quinol derivatives. Subtle electronic and steric e ects are often the keys to their high-yielding formation and controlled utilization in various types of chemical reactions. Today, ortho-quinol derivatives can be e ciently transformed by regioselective nucleophilic additions and substitutions, by photochemical ring-opening and ring-contracting rearrangements, and by Diels–Alder cycloadditions into versatile bicyclo[2.2.2]oct-5-en-2-one synthons. The increasing number of reports on successful utilizations of ortho-quinone monoketals and other ortho-quinols is certainly testament to their emerging prominent role in organic synthesis and, in particular, in natural products synthesis. May this chapter be a convincing compilation of examples from the flourishing chemistry of these 6-oxocyclohexa- 2,4-dienone derivatives.
Acknowledgements
The author thanks the De´le´gation Re´gionale a` la Recherche et a` la Technologie pour l’Aquitaine, the Conseil Re´gional d’Aquitaine, the Centre National de la Recherche Scientifique (CNRS), and the Centre de Recherche en Chimie Mole´culaire (CNRS, Fe´de´ration d’Unite´s FR1981) for their financial aid. The author also thanks his collaborators, Dr. Denis De eux and Dr. Laurent Pouyse´gu, for their help and continuous support.
References
1 A. J. Waring, in Advances in Alicyclic |
Karabatsos), Academic Press, New York |
Chemistry, Vol. 1 (Eds.: H. Hart, G. J. |
and London, 1966, pp. 129–256. |
|
|
|
References |
569 |
|
|
|
|
|
2 |
B. Miller, in Mechanisms of Molecular |
23 |
M. G. Burdon, J. G. Moffat, J. Am. |
|
|
Migrations, Vol. 1 (Ed.: B. S. |
|
Chem. Soc. 1966, 88, 5855–5864. |
|
|
Thyagarajan), Wiley, New York, 1968, |
24 |
K. E. Pfitzner, J. P. Marino, R. A. |
|
|
pp. 247–313. |
|
Olofson, J. Am. Chem. Soc. 1965, 87, |
|
3 |
S. Fujita, Yuki Gosei Kagaku Kyokaishi |
|
4658–4659. |
|
|
1982, 40, 307–320. |
25 |
J. P. Marino, K. E. Pfitzner, R. A. |
|
4 |
J. S. Swenton, Acc. Chem. Res. 1983, 16, |
|
Olofson, Tetrahedron 1971, 27, 4181– |
|
|
74–81. |
|
4194. |
|
5 |
J. S. Swenton, in The Chemistry of |
26 |
R. A. Olofson, J. P. Marino, Tetrahedron |
|
|
Quinonoid Compounds, Vol. 2, Part 2 (Eds.: |
|
1971, 27, 4195–4208. |
|
|
S. Patai, Z. Rappoport), John Wiley, New |
27 |
For a recent and elegant use of the Claisen |
|
|
York, 1988, pp. 899–962. |
|
rearrangement for the construction of |
|
6 |
S. Quideau, L. Pouyse´gu, Org. Prep. Proc. |
|
complex ortho-quinol intermediates in the |
|
|
Int. 1999, 31, 617–680. |
|
total synthesis of morellin natural |
|
7 |
For a recent example of an ortho-quinone |
|
products, see: K. C. Nicolaou, J. Li, |
|
|
methide-based cycloaddition approach |
|
Angew. Chem. Int. Ed. Engl. 2001, 40, |
|
|
to an ortho-quinol aryl ether, see: |
|
4264–4268, and references cited therein. |
|
|
G. G. Qiao, K. Lenghaus, D. H. |
28 |
S. Quideau, K. S. Feldman, Tetrahedron |
|
|
Solomon, J. Org. Chem. 1998, 63, 9806– |
|
2001, 57, ix–x. |
|
|
9811. |
29 |
K. Omura, J. Org. Chem. 1996, 61, 7156– |
|
8 |
R. Magnusson, Acta Chem. Scand. 1960, |
|
7161, and references cited therein. |
|
|
14, 1643–1653. |
30 |
E. J. Corey, L. F. Haefele, J. Am. Chem. |
|
9 |
R. Magnusson, Acta Chem. Scand. 1958, |
|
Soc. 1959, 81, 2225–2227. |
|
|
12, 791–792. |
31 |
Recent density functional theory (DFT) |
|
10 |
M. G. Dolson, J. S. Swenton, J. Am. |
|
calculations have suggested that cyclohexa- |
|
|
Chem. Soc. 1981, 103, 2361–2371. |
|
2,4-dienones and cyclohexa-2,5-dienones |
|
11 |
D. R. Henton, K. Anderson, M. J. |
|
have essentially the same heat of |
|
|
Manning, J. S. Swenton, J. Org. Chem. |
|
formation, see: D. Santoro, R. Louw, J. |
|
|
1980, 45, 3422–3433. |
|
Chem. Soc., Perkin Trans. 2 2001, 645–649. |
|
12 |
A. G. Schultz, Chem. Commun. 1999, |
32 |
D. J. Hart, P. A. Cain, D. A. Evans, J. |
|
|
1263–1271. |
|
Am. Chem. Soc. 1978, 100, 1548–1557. |
|
13 |
A. G. Schultz, J. P. Dittami, F. P. |
33 |
D. A. Evans, P. A. Cain, R. Y. Wong, J. |
|
|
Lavieri, C. Salowey, P. Sundararaman, |
|
Am. Chem. Soc. 1977, 99, 7083–7085. |
|
|
M. B. Szymula, J. Org. Chem. 1984, 49, |
34 |
L. Ku¨rti, L. Szilagyi, S. Antus, M. |
|
|
4429–4440. |
|
Nogradi, Eur. J. Org. Chem. 1999, 2579– |
|
14 |
J. M. Hook, L. N. Mander, M. Woolias, |
|
2581. |
|
|
Tetrahedron Lett. 1982, 23, 1095–1098. |
35 |
A. Nishiyama, H. Eto, Y. Terada, M. |
|
15 |
B. Miller, J. Org. Chem. 1970, 35, 4262– |
|
Iguchi, S. Yamamura, Chem. Pharm. Bull. |
|
|
4264. |
|
1983, 31, 2834–2844. |
|
16 |
B. Miller, J. Am. Chem. Soc. 1965, 87, |
36 |
A. Nishiyama, H. Eto, Y. Terada, M. |
|
|
5115–5120. |
|
Iguchi, S. Yamamura, Chem. Pharm. Bull. |
|
17 |
D. Y. Curtin, R. J. Crawford, J. Am. |
|
1983, 31, 2820–2833. |
|
|
Chem. Soc. 1957, 79, 3156–3159. |
37 |
B.-N. Su, Q.-X. Zhu, Z.-J. Jia, Tetrahedron |
|
18 |
D. Y. Curtin, R. J. Crawford, M. |
|
Lett. 1999, 40, 357–358. |
|
|
Wilhelm, J. Am. Chem. Soc. 1958, 80, |
38 |
B.-N. Su, L. Yang, K. Gao, Z.-J. Jia, Planta |
|
|
1391–1397. |
|
Medica 2000, 66, 281–283. |
|
19 |
D. Y. Curtin, D. H. Dybvig, J. Am. Chem. |
39 |
N. Abe, O. Sugimoto, K.-i. Tanji, A. |
|
|
Soc. 1962, 84, 225–232. |
|
Hirota, J. Am. Chem. Soc. 2000, 122, |
|
20 |
J. Zsindely, H. Schmid, Helv. Chim. Acta |
|
12606–12607. |
|
|
1968, 51, 1510–1514. |
40 |
K. C. Nicolaou, G. Vassilikogiannakis, |
|
21 |
M. G. Burdon, J. G. Moffat, J. Am. |
|
K. B. Simonsen, P. S. Baran, Y.-L. |
|
|
Chem. Soc. 1965, 87, 4656–4658. |
|
Zhong, V. P. Vidali, E. N. Pitsinos, |
|
22 |
M. G. Burdon, J. G. Moffat, J. Am. |
|
E. A. Couladouros, J. Am. Chem. Soc. |
|
|
Chem. Soc. 1967, 89, 4725–4735. |
|
2000, 122, 3071–3079. |

570 15 Oxidative Conversion of Arenols into ortho-Quinols and ortho-Quinone Monoketals
41 |
M. Tanaka, F. Nara, K. Suzuki-Konagai, |
59 |
M. Largeron, A. Neudorffer, M.-B. |
|
T. Hosoya, T. Ogita, J. Am. Chem. Soc. |
|
Fleury, J. Chem. Soc., Perkin Trans. 2 |
|
1997, 119, 7871–7872. |
|
1998, 2721–2727. |
42 |
S. Saito, N. Tanaka, K. Fujimoto, H. |
60 |
M. Largeron, H. Dupuis, M.-B. Fleury, |
|
Kogen, Org. Lett. 2000, 2, 505–506. |
|
Tetrahedron 1995, 51, 4953–4968. |
43 |
C. Arenz, M. Gartner, V. |
61 |
H. Eickhoff, G. Jung, A. Rieker, |
|
Wascholowski, A. Giannis, Bioorg. Med. |
|
Tetrahedron 2001, 57, 353–364, and |
|
Chem. 2001, 9, 2901–2904. |
|
references cited therein. |
44 |
K. A. Runcie, R. J. K. Taylor, Org. Lett. |
62 |
S. Torii, in Electroorganic Syntheses: |
|
2001, 3, 3237–3239. |
|
Methods and Applications, Part I: |
45 |
K. S. Feldman, M. D. Lawlor, K. |
|
Oxidations, VCH, Tokyo, 1984, pp. 97–152. |
|
Sahasrabudhe, J. Org. Chem. 2000, 65, |
63 |
M. Iguchi, A. Nishiyama, Y. Terada, S. |
|
8011–8019. |
|
Yamamura, Chem. Lett. 1978, 451–454. |
46 |
K. S. Feldman, M. D. Lawlor, J. Am. |
64 |
M. Iguchi, A. Nishiyama, Y. Terada, S. |
|
Chem. Soc. 2000, 122, 7396–7397. |
|
Yamamura, Tetrahedron Lett. 1977, 4511– |
47 |
K. S. Feldman, K. Sahasrabudhe, S. |
|
4514. |
|
Quideau, K. L. Hunter, M. D. Lawlor, in |
65 |
D. G. Hewitt, J. Chem. Soc. (C) 1971, |
|
Plant Polyphenols 2: Chemistry, Biology, |
|
2967–2973. |
|
Pharmacology, Ecology, Vol. Basic Life |
66 |
V. V. Karpov, M. L. Khidekel, Zhur. Org. |
|
Science 66 (Eds.: G. G. Gross, R. W. |
|
Khim. 1968, 4, 837–844. |
|
Hemingway, T. Yoshida), Kluwer |
67 |
E. Mu¨ller, R. Mayer, B. Narr, A. Rieker, |
|
Academic/Plenum Publishers, New York, |
|
K. Scheffler, Liebigs Ann. Chem. 1961, |
|
NY, 1999, pp. 101–125. |
|
645, 25–36. |
48 |
S. Quideau, K. S. Feldman, J. Org. Chem. |
68 |
F. Wessely, G. Lauterbach-Keil, F. |
|
1997, 62, 8809–8813. |
|
Sinwel, Monatsh. Chem. 1950, 81, 811– |
49 |
K. S. Feldman, S. Quideau, H. M. Appel, |
|
818. |
|
J. Org. Chem. 1996, 61, 6656–6665. |
69 |
F. Wessely, F. Sinwel, Monatsh. Chem. |
50 |
S. Quideau, K. S. Feldman, Chem. Rev. |
|
1950, 81, 1055–1070. |
|
1996, 96, 475–503. |
70 |
F. Wessely, J. Kotlan, F. Sinwel, |
51 |
D. J. Bennett, F. M. Dean, G. A. Herbin, |
|
Monatsh. Chem. 1952, 83, 902–914. |
|
D. A. Matkin, A. W. Price, M. L. |
71 |
F. Wessely, J. Kotlan, Monatsh. Chem. |
|
Robinson, J. Chem. Soc., Perkin Trans. 1 |
|
1953, 84, 291–297. |
|
1980, 1978–1985. |
72 |
F. Wessely, J. Kotlan, W. Metlesics, |
52 |
D. F. Bowman, F. R. Hewgill, B. R. Ken- |
|
Monatsh. Chem. 1954, 85, 69–79. |
|
nedy, J. Chem. Soc. (C) 1966, 2274–2279. |
73 |
W. Metlesics, E. Schinzel, H. Vilisek, |
53 |
D. R. Nelan, C. D. Robeson, J. Am. |
|
F. Wessely, Monatsh. Chem. 1957, 88, |
|
Chem. Soc. 1962, 84, 2963–2965. |
|
1069–1076. |
54 |
P. Schudel, H. Mayer, R. Ru¨egg, O. |
74 |
F. Takacs, Monatsh. Chem. 1964, 95, 961– |
|
Isler, Chimia 1962, 16, 368–369. |
|
977. |
55 |
V. K. Kansal, S. Funakoshi, P. |
75 |
F. Wessely, J. Swoboda, V. Guth, |
|
Mangeney, B. Gillet, B. Guittet, J. Y. |
|
Monatsh. Chem. 1964, 95, 649–670. |
|
Lallemand, P. Potier, Tetrahedron 1985, |
76 |
F. Wessely, M. Grossa, Monatsh. Chem. |
|
41, 5107–5120. |
|
1966, 97, 571–578. |
56 |
G. Pratviel, J. Bernadou, T. Ha, G. |
77 |
E. Hecker, R. Lattrell, Angew. Chem. |
|
Meunier, S. Cros, B. Meunier, B. |
|
1962, 74, 652. |
|
Gillet, E. Guittet, J. Med. Chem. 1986, |
78 |
A. McKillop, D. H. Perry, M. Edwards, |
|
29, 1350–1355. |
|
S. Antus, L. Farkas, M. No´gra´di, E. C. |
57 |
S. Itoh, M. Ogino, Y. Fukui, H. Murao, |
|
Taylor, J. Org. Chem. 1976, 41, 282–287. |
|
M. Komatsu, Y. Ohshiro, T. Inoue, Y. |
79 |
S. Antus, M. Nogradi, E. Baitz-gacs, L. |
|
Kai, N. Kasai, J. Am. Chem. Soc. 1993, |
|
Radics, H.-D. Becker, B. Karlsson, A.-M. |
|
115, 9960–9967. |
|
Pilotti, Tetrahedron 1978, 34, 2573–2577. |
58 |
J. L. Bolton, M. A. Trush, T. M. |
80 |
S. Quideau, M. A. Looney, L. Pouyse´gu, |
|
Penning, G. Dryhurst, T. J. Monks, |
|
S. Ham, D. M. Birney, Tetrahedron Lett. |
|
Chem. Res. Toxicol. 2000, 13, 135–160. |
|
1999, 40, 615–618. |
|
|
|
References |
571 |
|
|
|
|
|
81 |
D. H. R. Barton, S. V. Ley, P. D. |
107 |
A. Pelter, S. M. A. Elgendy, J. Chem. |
|
|
Magnus, M. N. Rosenfeld, J. Chem. Soc., |
|
Soc., Perkin Trans. 1 1993, 1891–1896. |
|
|
Perkin Trans. 1 1977, 567–572. |
108 |
L. Ku¨rti, P. Herczegh, J. Visy, M. |
|
82 |
D. H. R. Barton, P. D. Magnus, M. N. |
|
Simonyi, S. Antus, A. Pelter, J. Chem. |
|
|
Rosenfeld, J. Chem. Soc., Chem. Commun. |
|
Soc., Perkin Trans. 1 1999, 379–380. |
|
|
1975, 301. |
109 |
Y. Tamura, T. Yakura, J.-i. Haruta, |
|
83 |
K. Agbaria, O. Aleksiuk, S. E. Biali, V. |
|
Y. Kita, J. Org. Chem. 1987, 52, 3927– |
|
|
Bo¨hmer, M. Frings, I. Thondorf, J. Org. |
|
3930. |
|
|
Chem. 2001, 66, 2891–2899. |
110 |
A. J. Fatiadi, Synthesis 1974, 229–272. |
|
84 |
K. Agbaria, S. E. Biali, J. Org. Chem. |
111 |
A. Pelter, R. S. Ward, Tetrahedron 2001, |
|
|
2001, 66, 5482–5489. |
|
57, 273–282. |
|
85 |
G. Andersson, Acta Chem. Scand. 1976, B |
112 |
S. Quideau, L. Pouyse´gu, M. A. Looney, |
|
|
30, 64–70. |
|
J. Org. Chem. 1998, 63, 9597–9600. |
|
86 |
G. Andersson, Acta Chem. Scand. 1976, B |
113 |
S. Quideau, L. Pouyse´gu, M. Oxoby, |
|
|
30, 403–406. |
|
M. A. Looney, Tetrahedron 2001, 57, 319– |
|
87 |
E. Adler, G. Andersson, E. Edman, Acta |
|
329. |
|
|
Chem. Scand. 1975, B 29, 909–920. |
114 |
S. Quideau, M. A. Looney, L. Pouyse´gu, |
|
88 |
G. Andersson, P. Berntsson, Acta Chem. |
|
Org. Lett. 1999, 1, 1651–1654. |
|
|
Scand. 1975, B 29, 948–952. |
115 |
B. Miller, W.-O. Lin, J. Org. Chem. 1978, |
|
89 |
E. Adler, K. Holmberg, Acta Chem. |
|
43, 4441–4446. |
|
|
Scand. 1974, B 28, 465–472. |
116 |
J. L. Wood, B. D. Thompson, N. Yusuff, |
|
90 |
E. Adler, K. Holmberg, Acta Chem. |
|
D. A. Pflum, M. S. P. Mattha¨us, J. Am. |
|
|
Scand. 1974, B 28, 549–554. |
|
Chem. Soc. 2001, 123, 2097–2098. |
|
91 |
E. Adler, K. Holmberg, L.-O. Ryrfors, |
117 |
F. Wessely, W. Metlesics, Monatsh. |
|
|
Acta Chem. Scand. 1974, B 28, 888–894. |
|
Chem. 1954, 85, 637–653. |
|
92 |
H.-D. Becker, T. Bremholt, E. Adler, |
118 |
E. Zbiral, O. Saiko, F. Wessely, Monatsh. |
|
|
Tetrahedron Lett. 1972, 4205–4208. |
|
Chem. 1964, 95, 533–534. |
|
93 |
E. Adler, K. Holmberg, Acta Chem. |
119 |
G. Wells, A. Seaton, M. F. G. Stevens, J. |
|
|
Scand. 1971, 25, 2775–2776. |
|
Med. Chem. 2000, 43, 1550–1562. |
|
94 |
E. Adler, J. Dahlen, G. Westin, Acta |
120 |
V. Singh, Acc. Chem. Res. 1999, 32, 324– |
|
|
Chem. Scand. 1960, 14, 1580–1596. |
|
333. |
|
95 |
E. Adler, Angew. Chem. 1957, 69, 272. |
121 |
E. Adler, S. Brasen, H. Miyake, Acta |
|
96 |
E. Adler, S. Hernestam, Acta Chem. |
|
Chem. Scand. 1971, 25, 2055–2069. |
|
|
Scand. 1955, 9, 319–334. |
122 |
K. N. Houk, J. Am. Chem. Soc. 1973, 95, |
|
97 |
C. Arenz, A. Giannis, Angew. Chem. Int. |
|
4092–4094. |
|
|
Ed. Engl. 2000, 39, 1440–1442. |
123 |
O. Eisenstein, J. M. Lefour, N. T. Anh, |
|
98 |
C. Arenz, A. Giannis, Eur. J. Org. Chem. |
|
R. F. Hudson, Tetrahedron 1977, 33, 523– |
|
|
2001, 1, 137–140. |
|
531. |
|
99 |
A. Varvoglis, Synthesis 1984, 709–726. |
124 |
I. Fleming, J. P. Michael, L. E. |
|
100 |
A. Varvoglis, Hypervalent Iodine in |
|
Overman, G. F. Taylor, Tetrahedron Lett. |
|
|
Organic Synthesis, Academic Press, San |
|
1978, 15, 1313–1314. |
|
|
Diego, 1997. |
125 |
C.-C. Liao, C.-S. Chu, T.-H. Lee, P. D. |
|
101 |
A. Varvoglis, Tetrahedron 1997, 53, 1179– |
|
Rao, S. Ko, L.-D. Song, H.-C. Shiao, J. |
|
|
1255. |
|
Org. Chem. 1999, 64, 4102–4110. |
|
102 |
P. J. Stang, V. V. Zhdankin, Chem. Rev. |
126 |
C.-H. Lai, Y.-L. Shen, C.-C. Liao, Synlett |
|
|
1996, 96, 1123–1178. |
|
1997, 1351–1352. |
|
103 |
G. F. Koser, in The Chemistry of Functional |
127 |
W. Metlesics, W. Wessely, Monatsh. |
|
|
Groups (Eds.: S. Patai, Z. Rappoport), |
|
Chem. 1957, 88, 108–117. |
|
|
Wiley, New York, 1983, pp. 721–811. |
128 |
K. Holmberg, Acta Chem. Scand. 1974, B |
|
104 |
D. F. Banks, Chem. Rev. 1966, 66, 243–266. |
|
28, 857–865. |
|
105 |
A. Siegel, F. Antony, Monatsh. Chem. |
129 |
Y.-K. Chen, R. K. Peddinti, C.-C. Liao, |
|
|
1955, 86, 292–300. |
|
Chem. Commun. 2001, 1340–1341. |
|
106 |
A. Pelter, S. Elgendy, Tetrahedron Lett. |
130 |
C.-F. Yen, R. K. Peddinti, C.-C. Liao, |
|
|
1988, 29, 677–680. |
|
Org. Lett. 2000, 2, 2909–2912. |
