
Biomedical EPR Part-B Methodology Instrumentation and Dynamics - Sandra R. Eaton
.pdf
LOOP-GAP RESONATORS |
49 |
Grist, T. M. and Hyde, J. S. (1985) Resonators for in Vivo |
NMR at 1.5 T. J. Magn. Reson. |
61, 571-578.
Halpern, H. J., Spenser, D. P., van Polen, J., Bowman, M. K., Nelson, A. C., Dowey, E. M., and Teicher, B. A. (1989) Imaging Radio Frequency Electron-Spin-Resonance Spectrometer With High Resolution And Sensitivity For In Vivo Measurements. Rev. Sci. Instrum. 60, 1040-1050.
Hardy, W. N. and Whitehead, L. A. (1981) Split-Ring Resonator For Use In Magnetic Resonance From 200-2000 Mhz. Rev. Sci. Instrum. 52, 213-216.
Harrison, A. E. (1947) Klystron Tubes. McGraw-Hill Book Company, New York, pp. 12-13. Hayes, C. E. (1996) Birdcage and Other High Homogeneity Radiofrequency Coils for Whole
Body Magnetic Resonance, in Encyclopedia of Nuclear Magnetic Resonance, Grant, D. M., and Harris, R. K., eds., John Wiley & Sons, Chichester, pp. 968-974.
He, G., Samouilov, A., Kuppusamy, P., and Zweier, J. L. (2002) In Vivo Imaging Of Free Radicals: Applications From Mouse To Man. Molecular and Cellular Biochem. 234/235 359-367.
Hill, H. D. W. (1996) Probes for High Resolution, in Encyclopedia of Nuclear Magnetic Resonance, Grant, D. M., and Harris, R. K., eds., John Wiley & Sons, Chichester, pp. 3762-3771.
Hirata, H. and Ono, M. (1996) Resonance Frequency Estimation Of A Bridged Loop-Gap Resonator Used For Magnetic Resonance Measurements. Rev. Sci. Instrum. 67, 73-78.
Hornak, J. and Freed, J. H. (1986) Spectral Rotation in Pulsed ESR Spectroscopy. J. Magn. Reson. 67, 501-518.
Hubbell, W. L., Froncisz, W., and Hyde, J. S. (1987) Continuous and Stopped Flow EPR Spectrometer Based on a Loop Gap Resonator, Rev. Sci. Instrum. 58, 1879-1886.
Hubbell, W. L., Mchaourab, H. S., Altenbach, C., and Lietzow, M. A. (1996) Watching proteins move using site-directed spin labelling. Structure (London) 4, 779-783.
Hubbell, W., L., Fross, A., Langren, R., and Lietzow, M. A. (1998) Recent advances in sitedirected spin labeling of proteins. Curr. Opinion Struct. Biol. 8, 649-656.
Hyde, J. S. and Froncisz, W. (1981) ESR S-Band Microwave Spectrometer For Process Control. National Electronics Conference Proceedings 35, 602-606.
Hyde, J. S., Froncisz, W. and Kusumi, A. (1982) Dispersion Electron Spin Resonance With The Loop-Gap Resonator. Rev. Sci. Instrum. 53, 1934-1937.
Hyde, J. S., Yin, J.-J., Froncisz, W., and Feix, J. (1985) Electron-Electron Double Resonance (ELDOR) with a Loop-Gap Resonator. J. Magn. Reson. 63, 142-150.
Hyde, J. S. and Froncisz, W. (1986) Loop-Gap Resonators, in “Electron Spin Resonance” Symons, M. C. R., ed., Specialist Periodical Reports, Royal Society of Chemistry, London, Vol. 10, pp. 175-184.
Hyde, J. S. and Froncisz, W. (1989) Loop Gap Resonators. in “Advanced EPR: Applications in Biology and Biochemistry,” Hoff, A. J., ed., Elsevier, Amsterdam, pp. 277-306.
Hyde, J. S., Froncisz, W. and Oles, T. (1989) Multipurpose Loop-Gap Resonator. J. Magn. Reson. 82, 223-230.
Hyde, J. S., Yin, J.-J., Froncisz, W. and Feix, J. B. (1985) Electron-Electron Double Resonance (ELDOR) with a Loop-Gap Resonator. J. Magn. Reson. 63, 142-150.
Ichikawa, T., Yoshida, H. and Westerling, J. (1989) Coupling Structure for the Loop-Gap Resonator. J. Magn. Reson. 85, 132-136.
Jiang, J. Bank, J. F., and Scholes, C. P. (1993) Subsecond Time-Resolved Spin Trapping Followed by Stopped-Flow EPR of Fenton Reaction Products. J. Am. Chem. Soc. 115, 4742-4746.

50 |
GEORGE A. RINARD AND GARETH R. EATON |
Kan, S., Gonord, P., Duret, C., Salset, J., and Vibet, C. (1973) A Versatile And Inexpensive Electronic System For A High Resolution NMR Spectrometer. Rev. Sci. Instrum. 44, 1725-1733.
Lin, C. P., Bowman, M. K., and Norris, J. R. (1985) A Folded Half-Wave Resonator for ESR Spectroscopy. J. Magn. Reson. 65, 369-374.
LoBrutto, R., Smithers, G. W., Reed, G. H., Orme-Johnson, W. H., Tan, S. L., and Leigh, J. S. Jr. (1986) Observation of Manganese(II)-Ligand Superhyperfine Couplings in Complexes with Proteins by Electron Spin-Echo Spectroscopy. Biochem. 25, 5654-5660.
Lurie, D. J., Li, H., Petryakov, S., and Zweier, J. L. (2002) Development of a REDRI FreeRadical Imager Using a 0.38 T Clinical MRI System. Magn. Reson. Med. 47, 181-186.
Mazúr, M. Morris, H., and Valko, M. (1997). Analysis of the Movement of Line-like Samples of variable Length along the X-Axis of a Double and a Single
Rectangular Cavity. J. Magn. Reson. 129, 188-200.
Mazúr, M., Valko, M., and Morris, H.,. (2000). Analysis of the Radial and Longitudinal Effect in a Double and a Single
Rectangular Cavity. J. Magn. Reson. 142, 3756.
Mehdizadeh, M., Ishii, T. K., Hyde, J. S., and Froncisz, W. (1983) Loop-Gap Resonator: A Lumped Mode Microwave Resonant Structure. IEEE Trans. Microwave Theory Tech. MTT31, 1059-1064.
Mims, W. B. (1965a) Electron Echo Methods in Spin Resonance Spectrometry. Rev. Sci. Instrum. 36, 1472-1479.
Mims, W. B. (1965b) Electron Spin Echoes, in Electron Paramagnetic Resonance, S.
Geschwind, ed., Plenum, New York. |
|
|
More, K. M., Eaton, G. R. and Eaton, S. S. (1984) Determination of |
and |
by Simulation |
of EPR Power Saturation Curves and Saturated Spectra: Application to Spin-Labeled Iron Porphyrins, J. Magn. Reson. 60, 54-65.
Oles, T., Hyde, J. S. and Froncisz, W. (1989) Gordon Coupler for K-Band Loop Gap Resonator. Rev. Sci. Instrum. 60, 389-391.
Ono, M., Ogata, T., Hsieh, K.-C., Suzuki, M., Yoshida, E., and Kamada, H. (1986a) Development of Lop-Gap Resonator and a Surface Coil With an Electric Shield. Rocky Mountain Conference, Abstract 105, Denver, CO, USA, August 1986.
Ono, M., Ogata, T., Hsieh, K.-C., Suzuki, M., Yoshida, E., and Kamada, H. (1986b) L-Band ESR Spectrometer Using a Loop-Gap Resonator for In Vivo Analysis. Chem. Lett. 491494.
Ogata, T., Ono, M., Yoshida, E., and Kamada, H. (1986) Application of L-band ESR Techniques to In Vivo Analysis. Rocky Mountain Conference, Abstract 103, Denver, CO, USA, August 1986.
Perman, W. H., Bernstein, M. A., and Sandstrom, J. C. (1989) A Method for Correctly Setting the rf Flip Angle. Magn. Reson. Med. 9, 16-24.
Pfenninger, S., Schweiger, A., Forrer, J., and Ernst, R. R. (1988) Echo-Detected ESR Spectroscopy with Magnetic Field Vector Jumps: A Novel Approach for Improving the Spectral Resolution in Disordered Systems. Chem. Phys. Lett. 151, 199-204.
Pfenninger, S., Froncisz, W., Forrer, J., Luglio, J., and Hyde, J. S. (1995) General Method for Adjusting the Quality Factor of EPR Resonators. Rev. Sci. Instrum. 66, 4857-4965.
Piasecki, W., Froncisz, W., and Hyde, J. S. (1996) Bimodal Loop-Gap Resonator. Rev. Sci. Instrum. 67, 1896-1904.
Poole, C. P. Jr. (1967) Electron Spin Resonance: A Comprehensive Treatise on Experimental Techniques. Wiley-Interscience, New York, page 291.
LOOP-GAP RESONATORS |
51 |
Poole, C. P. Jr. and Farach, H. A. (1999) Resonators, Chapter III in Handbook of Electron Spin resonance, Vol. 2, Poole, C. P. Jr. and Farach, H. A., eds., AIP Press SpringerVerlag, New York.
Quine, R. W., Rinard, G. A., Ghim, B. T., Eaton, S. S. and Eaton, G. R. (1996) A 1-2 GHz Pulsed and Continuous Wave Electron Paramagnetic Resonance Spectrometer. Rev. Sci. Instrum. 67, 2514-2527.
Reichert, J. F., and Townsend, J. (1965). Dynamic Nuclear Enhancement in Metallic Sodium,
Phys. Rev. 137, 476-482.
Rinard, G. A., Quine, R. W., Eaton, S. S. and Eaton, G. R. (1993) Microwave Coupling Structures for Spectroscopy, J. Magn. Reson. A105, 134-144.
Rinard, G. A., Quine, R. W., Eaton, S. S., Eaton, G. R., and Froncisz, W. (1994) Relative Benefits of Overcoupled Resonators vs. Inherently Low-Q Resonators for Pulsed Magnetic Resonance. J. Magn. Reson. A108, 71-81.
Rinard, G. A., Quine, R. W., Ghim, B. T., Eaton, S. S. and Eaton, G. R. (1996a) Easily Tunable Crossed-Loop (Bimodal) EPR Resonator. J. Magn. Reson. A122, 50-57.
Rinard, G. A., Quine, R. W., Ghim, B. T., Eaton, S. S. and Eaton, G. R. (1996b) Dispersion and Superheterodyne EPR Using a Bimodal Resonator. J. Magn. Reson. A122, 58-63.
Rinard, G. A., Eaton, S. S., Eaton, G. R., Poole, C. P. Jr., and Farach, H. A. (1999a) Sensitivity in EPR Measurements, Handbook of Electron Spin Resonance, C. P. Poole, Jr., and H. A. Farach, eds, vol 2, AIP Press, 1-23
Rinard, G. A., Quine, R. W., Song, R., Eaton, G. R. and Eaton, S. S. (1999b) Absolute EPR Spin Echo and Noise Intensities, J. Magn. Reson. 140, 69-83
Rinard, G. A., Quine, R. W., Harbridge, J. R., Song, R., Eaton, G. R. and Eaton, S. S. (1999c) Frequency Dependence of EPR Signal-to-Noise, J. Magn. Reson. 140, 218-227.
Rinard, G. A., Quine, R. W., and Eaton, G. R. (1999d) A Cryogenically Coolable Microwave Limiter. J. Magn. Reson. 136, 207-210.
Rinard, G. A., Quine, R. W., and Eaton, G. R. (2000) An L-band Crossed-Loop (Bimodal) Resonator, J. Magn. Reson. 144, 85-88.
Rinard, G. A., Quine, R. W., Eaton, G. R. and Eaton, S. S. (2002a) 250 MHz Crossed Loop Resonator for Pulsed Electron Paramagnetic Resonance, Magn. Reson. Engineer. 15, 3746.
Rinard, G. A., Quine, R. W., Eaton, S. S., and Eaton, G. R. (2002b) Frequency Dependence of EPR Signal Intensity, 248 MHz to 1.4 GHz, J. Magn. Reson. 154, 80-84.
Rothenberger, K. S., Nilges, M. J., Altman, T. E., Glab, K., Belford, R. L., Froncisz, W., and Hyde, J. S. (1986) L-Band Parallel Mode EPR. Measurement of Quadrupole Coupling Through Direct Observation of Secondary Transitions. Chem. Phys. Lett. 124, 295-298.
Saalmueller, J. W., Long, H. W., Maresch, G. G., and Spiess, H. W. (1995) Two-Dimensional Field-Step ELDOR. A Method for Characterizing the Motion of Spin Probes and Spin Labels in Glassy Solids. J. Magn. Reson. A 117, 193-208.
Schneider, H. J. and Dullenkopf, P. (1977). Slotted Tube Resonator: A New NMR Probe Head At High Operating Frequencies. Rev. Sci. Instrum. 48, 68-73.
Shin, Y.-K., Levinthal, C., Levinthal, F. and Hubbell, W. L. (1993) Colicin E1 Binding to Membranes: Time-Resolved Studies of Spin-Labeled Mutants. Science 259, 960-963.
Sienkiewicz, A., Qu, K., and Scholes, C. P. (1994) Dielectric Resonator-Based Stopped-Flow Electron Paramagnetic Resonance. Rev. Sci. Instrum. 65, 68-74.
Squires, T. L. (1963) Introduction to Microwave Spectroscopy. George Newnes Ltd., London, p. 53-54.
Sotgiu, A. (1985) Resonator Design for In Vivo ESR Spectroscopy. J. Magn. Reson. 65, 206-
214.
52 GEORGE A. RINARD AND GARETH R. EATON
Sotgiu, A. and Gualtieri (1985) Cavity Resonator For In Vivo ESR Spectroscopy. J. Phys. E Sci. Instrum. 18, 899-901.
Subczynski, W. K., Lukiewicz, S., and Hyde, J. S. (1986) Murine In Vivo L-Band ESR SpinLabel Oximetry with a Loop-Gap Resonator. Magn. Reson. Med. 3, 747-754.
Symons, M. C. R. (1995) Whole Body Electron Spin Resonance Imaging Spectrometer. in “Bioradicals Detected by ESR Spectroscopy.” Ohya-Nishiguchi, H. and Packer, L. eds. Birkhäuser Verlag, Basel, Switzerland.
Terman, F. E. (1943) Radio Engineers Handbook, McGraw-Hill, New York, page 55. Thomas, D. D., Wendt, C. H., Froncisz, W., and Hyde, J. S. (1983) Saturation Transfer EPR
Spectroscopy on Spin-Labeled Muscle Fibers Using a Loop-Gap Resonator. Biophys. J. 43, 131-135.
Tsapin, A. I., Hyde, J. S., and Froncisz, W. (1992) Bimodal Loop-Gap Resonator. J. Magn. Reson. 100, 484-490.
Wood, R. L., Froncisz, W., and Hyde, J. S. (1984) The Loop-Gap Resonator. II. Controlled Return Flux Three-Loop, Two-Gap Microwave Resonators for ENDOR and ESR Spectroscopy. J. Magn. Reson. 58, 243-253.
Chapter 3
EPR Interfaced To Rapid Mixing
Charles P. Scholes
Department of Chemistry, University at Albany –State University of New York, Albany, NY 12222
Abstract: We describe the development of flow and stopped-flow EPR starting with large mixing devices designed for standard metallized EPR cavities and proceeding to micro mixing systems intimately attached to micro resonant structures. The characteristics of resonant structures dedicated to close attachment of liquid mixing systems with low dead volumes, short mixing times, and minimal microwave microphonic perturbation are outlined. The application of such systems to study of radical systems, either spin labelled, naturally occurring, or trapped, is reviewed, and recent experiments to probe rapid folding kinetics of site-directed spin labeled protein are summarized.
1.INTRODUCTION
1.1Technical Background for Flow and Stopped-Flow EPR
As early as 1940 Britton Chance had provided insightful, groundbreaking technical designs for flow, accelerated flow, and stopped-flow apparatus to follow rapid chemical reactions (Chance, 1940a; Chance, 1940b). Limitations from dead time, quality of mixing, and signal-to-noise in UVVis detection were described. As compared with the continuous flow method, the stopped-flow method, in which observations starting soon after mixing were made on a stationary but still reacting system, provided an important saving in reactant.
53
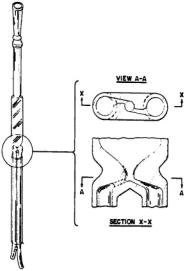
54 |
CHARLES P. SCHOLES |
1.2Flow and Stopped-Flow EPR Using Conventional Metallized EPR Cavities
Figure 1. Mix-and-flow cell is shown. This had inner dimensions of 45 X 9 X 0.25 mm for the flat chamber and 1.1 and 1.5 mm for the diameters of the offset inlet jets and of the mixing chambers. The dead volume from mixer to center of the EPR-active zone was Level X-X exhibits a top view through the mixing chamber, and A-A exhibits a crosssectional view through the mixing chamber. Figure used with permission of D. Borg.
By the late 1950’s EPR flow flat cells of a type still marketed by Wilmad had appeared. These cells were designed for insertion into the electric fieldfree region of a standard cavity. One such flat cell was reported to have a volume between mixer and center of the EPR active zone of
(Yamazaki et al., 1960). The flow was driven by compressed gas, and with a reported flow rate of 12 mL/s, spectra of radicals of age greater than ~15 ms could be obtained. The spectra of p-semiquinone and ascorbate radicals following enzymatic oxidation, respectively of quinol and ascorbate by peroxide, were observed during flow and collected by rapid recorder sweep (Yamazaki et al., 1960). The reactant usage for an overall experiment of the type reported by Yamazaki et al. (1960) was ~ 1 L (Borg and Elmore, 1967), thus limiting experiments to readily available, inexpensive compounds. Improved EPR cells of the mix-and-flow type shown in Figure 1 with smaller dead volumes and mixers placed as close as possible to the EPR cavity were reported by Borg (Borg, 1964a; Borg, 1964b). With a
dead volume from mixer to the center of the EPR-active zone, Borg’s fastest
EPR INTERFACED TO RAPID MIXING |
55 |
device allowed a dead time of several milliseconds. Detailed multi hyperfine line EPR spectra of short-lived tyrosyl radical intermediates arising from oxidation of a M tyrosine solution were recorded using a rapid field sweep during a flow which lasted ~3 s. Even here the reactant usage was >25 mL in 3 s. Although electrically driven solenoids were used for stopping flow, their stopping times were limited, and they provided electrical pick-up interference. It would appear that positive displacement devices for providing stopped-flow shots were avoided because of their potential for creating high pressures leading to breakage of glassware and to stopping/starting microphonic transients. It was recognized in principle that a Q-band cavity could provide considerably higher EPR sensitivity with considerable smaller usage of sample if equipped with a sample tube of ~0.2 mm diameter (Borg and Elmore, 1967).
2.THE LOOP GAP RESONATOR BASED STOPPED-FLOW SYSTEM
In the 1980’s the technology of small resonant structures, in contrast to large microwave cavities, had emerged. The loop-gap resonator (LGR) (see Chapter on Loop Gap Resonators) was notably developed at the National ESR Center (Froncisz and Hyde, 1982). Compared to a standard X-band cavity, the LGR had a greater filling factor, much smaller size, low Q, and relative insensitivity to dielectric losses. For small point samples the LGR could have a 50-fold sensitivity improvement over a standard rectangular cavity. With considerable isolation between magnetic and electric parts of the microwave field, the LGR was particularly useful for small static liquid samples, especially when
dispersion methods were used that took advantage of their low Q (Froncisz et al., 1985). In 1987 Hubbell et al. (1987) reported a novel design for continuous and stoppedflow EPR based on a loop-gap resonator (LGR) shown in Figure 2. Compared to conventional metallized cavity resonators, this design had a very high filling factor, relatively low resonator quality factor Q, and good signal-to-noise ratio for small microliter
sample volumes. The small size of the resonator made the very close location of the mixer to the resonator possible. The close location led to a small
dead volume (including mixer volume plus volume to center of EPR active zone) and ~4 ms dead time at a total flow rate of 1.5 mL/s. The liquid delivery system (manufactured by Update Instruments, Madison, WI) used a positive displacement syringe ram with a programmable dynamic braking motor. This delivery system fed the highly efficient Wiskind grid mixer, whose purpose was to assure that the mixing was completed at the mixer so that time-zero for a chemical reaction started at the mixer. Constant flow
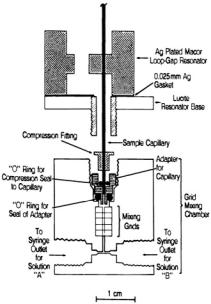
56 |
CHARLES P. SCHOLES |
velocity with high efficiency mixing is important for obtaining EPR signals at precisely controlled times after mixing and for inferring the existence of faster unresolved kinetics during the dead time. For kinetics at a single field approximately of each reactant was used per shot, and for the 100 ms period of a rapid field scan the amount of each reactant consumed during flow with a 2.4 ms dead time was
In concept the LGR was well adapted for kinetic studies of biological materials, which are characterized by a high dielectric loss and are often limited in supply. Integration of the LGR-based stopped-flow system into an overall EPR spectrometer was similar to that shown below in Figure 8 for the DR-based system. This stopped-flow device was used by Shin et al. (1993) to follow the interaction of the channel-forming fragment of toxin colicin E1 with membranes. The fragment interacted with the membrane in two distinct steps which were monitored by site-directed spin labels at separate, distinct positions: (i) rapid adsorption to the surface on the time scale of 5 s; and (ii) slow, rate-limiting insertion of the hydrophobic central helices into the membrane interior on the time scale of 100 s.
Figure 2. Figure showing the adaptation of the grid mixer to the loop gap resonator. The Lucite four-grid Wiskind mixer is commercially available from Update Instruments, Madison, WI. The sample capillary is sealed in place by O-ring fittings which are compressed as the mixer is screwed into the threaded extension from the resonator base. Figure reprinted from Hubbell et al. (1987) by permission of J. S. Hyde.

EPR INTERFACED TO RAPID MIXING |
57 |
2.1Application of LGR-Based Stopped-Flow EPR to Time-Resolved Spin Probe Oximetry
Our impetus for developing time-resolved spin probe oximetry was rapidly to measure oxygen consumption by cytochrome c oxidase, the enzyme which consumes the majority of oxygen for all aerobic organisms (Jiang et al., 1992). Cytochrome c oxidase takes four electrons from reduced ferrocytochrome c (cyt combines them in a sequential fashion with oxygen and protons to provide
plus ferricytochrome c (cyt
and couples the resultant free energy derived from this reaction to creating a transmembrane proton chemical gradient.
In probing cytochrome c oxidase kinetics under those limited turnover conditions where finite ratios of substrate (usually the cyt reductant) to enzyme hold, researchers had generally followed the transient spectrophotometric changes of cyt
We wanted to measure oxygen consumption in a similar time regime to the time regime in which cyt
consumption was measured. This would be done at a limited ratio of cyt
to cytochrome oxidase enzyme and also at a low, limited mole ratio of oxygen consumed to cytochrome oxidase enzyme.
The relaxation times of freely tumbling nitroxide spin probes such as CTPO (2,2,5,5-Tetramethyl-3-pyrrolin-1-oxyl-3-carboxamide; see Figure 3) are sensitive to oxygen concentration. Molecular collisions between spin probe and paramagnetic di-oxygen modulate the Heisenberg exchange between probe spin and the triplet oxygen molecule (Windrem and Plachy, 1980), thereby enhancing the magnetic and
relaxation rates of the spin probe. In pilot time-resolved spin probe oximetry following the development of the low-Q X-band LGR, Froncisz et al. (1985) used
dispersion
spin probe oximetry to measure oxygen consumption as shown in Figure 3 by microliter-size volumes containing
respiring cells. The oxygen-induced change in the field modulated adiabatic rapid-passage dispersion signal from CTPO was detected 90 ° out- of-phase with respect to 100 KHz field modulation. The 90 ° out-of-phase dispersion signal diminished with diminishing oxygen concentration, and the signal change was proportional to oxygen concentration at oxygen concentrations below
i.e., the solution oxygen concentration in
equilibrium with 20 % Air (Froncisz et al., 1985; Jiang et al., 1992).
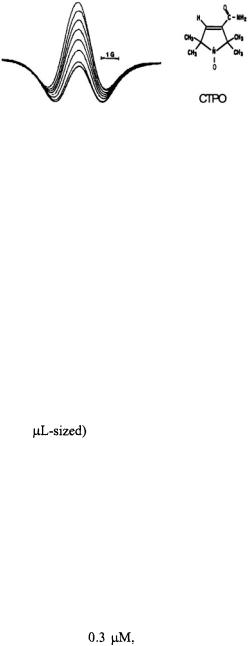
58 |
CHARLES P. SCHOLES |
Figure 3. Spectra showing the time course of the 90 ° out-of-phase dispersion signal from the central nitrogen hyperfine line of CTPO over the course of
consumption by Chinese hamster ovary cells. Scans were taken every two minutes, and the central feature diminished as the oxygen was consumed. Figure reprinted from Froncisz et al. (1985) by permission of J. S. Hyde.
The emergence of spin probe oximetry methodology (Lai et al., 1982; Froncisz et al., 1985), availability of stopped-flow EPR apparatus capable of rapid time resolution on small volumes (Hubbell et al., 1987), and our interest in structure and function of cytochrome oxidase (van Camp et al., 1978; Stevens et al., 1982; Mascarenhas et al., 1983; Fan et al., 1988) led us to develop time-resolved spin probe oximetry. The technique was applied to microliter volumes of purified mammalian cytochrome c oxidase enzyme at micromolar concentrations of enzyme. Continuously in time, micromolar oxygen concentration changes were followed where the amount of oxygen consumed became comparable with or ultimately even less than the amount of enzyme. The time-resolved spin probe oximetry method (Jiang et al., 1992) contrasted sharply with oxygen measuring methods such as polarography (Davies, 1962) or oxygen-dependent phosphorescence, both of
which used mL (vs |
samples with time resolution limited to > 100 |
ms by stirring (Vanderkooi |
et al., 1987; Wilson et al., 1988). In most |
polarographic oxygen monitoring experiments the amount of oxygen consumed was orders of magnitude larger than the amount of cytochrome c oxidase, unlike the case presented here.
In the kinetic case which is shown here, oxygen consumption was limited by the very availability of oxygen itself (i.e., the system became anaerobic) while cyt remained in excess. Zeroth-order kinetic rate behavior (i.e., a constant rate of oxygen consumption) was observed until almost all the oxygen was exhausted as shown in Figure 4. (Zeroth order kinetics mean that another processes besides the binding of oxygen, such as internal electron transfer within the cytochrome oxidase, limited the rate of oxygen
consumption.) |
The oxygen concentration where the rate diminished to half |
||
its maximal value was about |
and at that point the rate of oxygen |
||
consumption |
went exponentially |
to zero. |
Time-resolved spin-probe |