
Wasserscheid P., Welton T. - Ionic Liquids in Synthesis (2002)(en)
.pdf126 |
Paul C. Trulove Robert A. Mantz |
|
|
|
65 R. A. Mantz, H. C. De Long, R. A. |
66 |
C. J. Dymek, L. A. King, J. Electrochem. |
|
|||
|
Osteryoung, P. C. Trulove, in Proceed- |
|
Soc., 1985, 132, 1375. |
|
ings of the Twelfth International Sympo- |
67 |
C. L. Hussey, J. R. Sanders, H. A. Øye, |
|
sium on Molten Salts (P. C. Trulove, |
|
J. Electrochem. Soc., 1985, 132, 2156. |
|
H. C. De Long, G. R. Stafford, S. Deki |
68 |
C. L. Hussey, H. A. Øye, J. Elec- |
|
eds.), The Electrochemical Society: |
|
trochem. Soc., 1984, 131, 1623. |
|
Pennington NJ, 2000, Vol. 99-41, |
69 |
C. L. Hussey, J. R. Sanders, J. Elec- |
|
pp.169–176. |
|
trochem. Soc., 1987, 134, 1977. |

Ionic Liquids in Synthesis. Edited by Peter Wasserscheid, Thomas Welton Copyright © 2002 Wiley-VCH Verlag GmbH & Co. KGaA
ISBNs: 3-527-30515-7 (Hardback); 3-527-600701 -Kolumnentitel1 (Electronic) 127
4
Molecular Structure and Dynamics
W. Robert Carper, Andreas Dölle, Christof G. Hanke, Chris Hardacre, Axel Leuchter, Ruth M. Lynden-Bell, Zhizhong Meng, Günter Palmer, and Joachim Richter
4.1
Order in the Liquid State and Structure
Chris Hardacre
The structure of liquids has been studied for many years. These investigations have, in general, been focussed on the arrangements in molecular solvents such as water, t-butanol, and simple chlorinated solvents. The field of molten salts and their structures is much less studied, and within this field the study of the structure of roomtemperature ionic liquids is in its infancy. A variety of techniques have been used to investigate liquid structure, including neutron diffraction, X-ray scattering, and extended X-ray absorption fine structure. This chapter summarizes some of the techniques used, including practical details, and shows examples of where they have been employed previously. The examples given are not meant to be exhaustive and are provided for illustration only. Where possible, examples relating to the more recent airand moisture-stable ionic liquids have been included.
4.1.1
Neutron Diffraction
Neutron diffraction is one of the most widely used techniques for the study of liquid structure. In the experiment, neutrons are elastically scattered off the nuclei in the sample and are detected at different scattering angles, typically 3º to 40º, for the purpose of measuring intermolecular structure whilst minimizing inelasticity corrections. The resultant scattering profile is then analyzed to provide structural information.
The data taken is normally presented as the total structure factor, F(Q). This is related to the neutron scattering lengths bi, the concentrations ci, and the partial structure factor Sij(Q) for each pair of atoms i and j in the sample, by Equation 4.1-1:
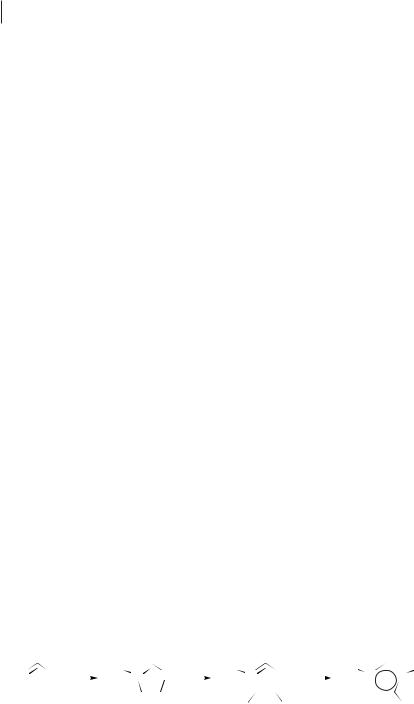
128 Chris Hardacre
F(Q) = ∑cicjbibj (Sij (Q) −1) |
(4.1-1) |
i, j |
|
where Q is the scattering vector and is dependent on the scattering angle θ, and the wavelength λ of the neutrons used.
Q = 4π sinθ |
(4.1-2) |
|
|
λ |
|
The real space pair distributions gij(r) is the inverse Fourier transform of (Sij(Q)-1), that is:
1 |
∞ |
|
2 sin(Qr) |
(Sij (Q) −1)dQ |
|
||
∫0 |
Q |
|
|||||
gij(r) = 1 + |
|
|
|
(4.1-3) |
|||
2π 2 ρ |
|
Qr |
normalized to the atomic density ρ.
In a neutron diffraction experiment, the quantity measured as a function of angle is the total scattering cross section, which consists of two components: (i) neutrons that scatter coherently (that is, where phase is conserved and the signal of which contains structural information) and (ii) incoherently scattered neutrons that result in a background signal. The scattering amplitude is then determined by the concentration, atomic arrangement, and neutron scattering lengths of the atoms involved. Since different isotopes have different neutron scattering lengths, it is possible to simplify the analysis of the neutron data simply by isotopic exchange experiments and by taking first and second order difference spectra to separate out the partial pair distribution functions. This is clearly set out by Bowron et al. [1] for a mixture of t-butanol/water and illustrates how isotopic substitution neutron scattering experiments can assist in distinguishing between intermolecular and intramolecular distributions within a sample, both of which would otherwise contribute to a measured diffraction pattern in a complex and often difficult to interpret combination.
4.1.2
Formation of Deuteriated Samples
In general, isotopic exchange is both expensive and difficult. In the case of many room-temperature ionic liquids, however, the manufacture of deuterated ionic liquids is relatively easily achievable. For example, the general synthesis of 1-alkyl-3- methylimidazolium salts is shown in Scheme 4.1-1 [2]. This methodology allows maximum flexibility in the deuteration on the imidazolium cation; that is, it can be either ring or side chain deuteration or both.
|
|
|
|
|
|
|
|
|
|
|
|
|
|
D |
|
|
D |
|
||||
HN N |
(a) |
D3C N N |
(b) |
D3C N |
|
|
|
|
N |
(c) |
D3C N |
|
|
N R |
||||||||
|
|
|
|
+ |
||||||||||||||||||
|
|
|
|
|
|
|
||||||||||||||||
|
|
|
|
|
|
|
|
|
|
|
|
|
|
|
|
|
|
|
|
|
||
|
|
|
|
|
|
|
|
|
|
|
|
D |
|
|
|
|
D |
|
D |
|
|
D |
|
|
|
|
|
|
|
|
|
|
|
|
|
|
|
|
|
|
|
||||
|
|
|
|
|
|
|
|
|
|
|
|
|
|
|
|
|
|
|
Scheme 4.1-1: Reaction scheme showing a method for deuteration of 1-alkyl-3-methylimidazoli- um salts: (a) CD3OD, RuCl3/(n-BuO)3P, (b) D2O, 10 % Pd/C, and (c) RX (CD3Cl, C2D5I).

4.1 Order in the Liquid State and Structure 129
4.1.3
Neutron Sources
The following sources and instruments dominate studies in the area of liquids and amorphous materials. Although there are a number of sources available, each is optimized for a particular class of experiment. The sources can be split into two types: pulsed neutron sources and reactor sources
4.1.3.1Pulsed (spallation) neutron sources
One example of a pulsed neutron source is to be found at ISIS, at the Rutherford Appleton Laboratory, UK. This source has the highest flux of any pulsed source in the world at present, and is therefore one of the most suitable for isotopic substitution work, as this class of experiment tends to be flux-limited. At ISIS, two stations are particularly well set up for the examination of liquids.
SANDALS station (Small Angle Neutron Diffractometer for Amorphous and Liquid Samples) This station is optimized for making measurements on liquids and glasses that contain light elements such as H, Li, B, C, N, and O. This set-up relies on collection of data at small scattering angles and the use of high-energy neutrons. This combination of characteristics has the effect of reducing the corrections necessary for the inelastic scattering that otherwise dominates the measured signal and hence complicates the extraction of structural signal information. This instrument is singularly specifically optimized for H-D isotopic substitution experiments.
GEM (GEneral Materials diffractometer) GEM is designed with extremely stable detectors, covering a very large solid angle, and is optimized for collection of data at a very high rate. It is a hybrid instrument that can perform both medium/high-res- olution powder diffraction studies on crystalline systems and very accurate total scattering measurements for liquids and glasses. Because of the high stability of the detectors and data acquisition electronics, it is suitable for isotopic substitution work on systems containing elements with only small differences in the isotope neutron scattering lengths, such as 12C and 13C.
ISIS is only one pulsed source available for the study of liquids. Both the USA and Japan have facilities similar to SANDALS and GEM for studying liquids, but with slightly lower neutron intensity: in the forms of the IPNS (Intense Pulsed Neutron Source) at the Argonne National Lab. on the instrument GLAD, and the KEK Neutron Scattering Facility (KENS) on the instrument Hit II, respectively.
4.1.3.2Reactor sources
The Institute Laue–Langevin (ILL, Grenoble, France) has arguably the premier neutron scattering instrument for total scattering studies of liquid and amorphous materials, in instrument D4C. The neutrons are provided by a reactor source that is very stable and delivers a very high flux. This makes the ILL ideal for isotopic substitution work for elements with atomic numbers greater than that of oxygen. For
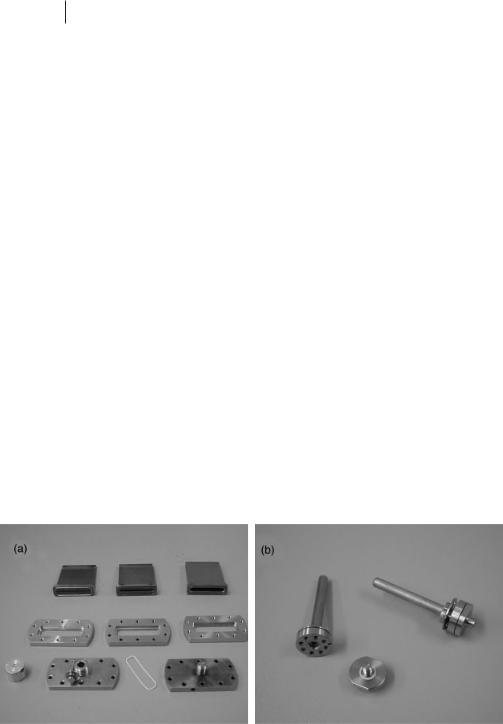
130 Chris Hardacre
light elements, the inelasticity corrections are a major problem, as the instrument collects data over a large angular range and with relatively low-energy neutrons. Typically the wavelength of the neutrons used on D4 is 0.7 Å, which is quite long when compared with a source such as ISIS where wavelengths ranging from 0.05 Å to 5.0 Å can be used on an instrument like SANDALS.
Other reactor sources with instruments like D4C but with much lower flux, and hence longer data collection times, are the Laboratoire Leon Brillouin (LLB, Saclay, France), on the instrument 7C2, and the NFL (Studsvik, Sweden), on the instrument SLAD
The following website provides links to all the neutron sites in the world: http://www.isis.rl.ac.uk/neutronSites/.
4.1.4
Neutron Cells for Liquid Samples
As in any scattering experiment, the ideal sample holder is one that does not contribute to the signal observed. In neutron scattering experiments, the typical cells used are either vanadium, which scatters neutrons almost completely incoherently (that is, with almost no structural components in the measured signal), or a null scattering alloy of TiZr alloy. Vanadium cells react with water and are not ideal for studies of hydrated systems, whereas TiZr is more chemically inert; TiZr cells have been used, for example, to study supercritical water and alkali metals in liquid ammonia. In addition, TiZr cells capable of performing measurements at high pressure have been constructed. Figure 4.1-1 shows typical sample cells made from Vanadium and TiZr alloy.
Cells used for high-temperature measurements in furnaces often consist of silica sample tubes, supported by thin vanadium sleeves. The key to the analysis is whether it is possible to have a container that scatters in a sufficiently predictable way, so that its background contribution can be subtracted. With the current neutron flux available from both pulsed and reactor sources, sample volumes of
Figure 4.1-1: Liquid sample cells made from (a) TiZr alloy and (b) vanadium.

4.1 Order in the Liquid State and Structure 131
between 1 and 5 cm3 are required. Obviously, with increasing flux at the new neutron sources being built in the USA and Japan, the sample sizes will decrease.
4.1.5
Examples
Neutron diffraction has been used extensively to study a range of ionic liquid systems; however, many of these investigations have focussed on high-temperature materials such as NaCl, studied by Enderby and co-workers [3]. A number of liquid systems with relatively low melting points have been reported, and this section summarizes some of the findings of these studies. Many of the salts studied melt above 100 oC, and so are not room-temperature ionic liquids, but the same principles apply to the study of these materials as to the lower melting point salts.
4.1.5.1Binary mixtures
A number of investigations have focussed on alkali haloaluminates: that is, (MX)y(AlX3)1–y mixtures in which M is an alkali metal and X is a halogen (Cl or Br). Blander et al. [4] have used neutron diffraction combined with quantum chemical calculations to investigate the salts formed from KBr and KCl where y = 0.25 and 0.33. The authors showed that, for both bromide and chloride salts, [Al2X7]– was the dominant species present, as expected, in full agreement with other spectroscopic techniques such as Raman and infra-red [5]. In the case of chloride, however, as the acidity of the melt increased (that is, as y decreased), although the proportion of [Al3X10]– ions did increase, the change was unexpectedly smaller than that predicted by the stoichiometry. A closer relationship with the stoichiometry was found for bromide. The neutron scattering data showed a strong correlation between the liquid structure and that found in, for example, the crystal structure of K[Al2Br7]. In both the liquid and crystal, the angle of the Al–Br–Al bridge within the [Al2Br7]– anion is found to be approximately 109 o and both also show that the neighboring structural units pack parallel to each other.
The structures of binary mixtures of AlCl3 with NaCl and LiCl were studied from pure AlCl3 to a 1:1 mixture by Badyal et al. [6]. In the pure AlCl3 liquid, the neutron data indicates that the long held view that isolated Al2Cl6 dimers make up the structure may not be the true scenario. The structure is reported to be a sparse liquid network made up of polymeric species containing corner-shared tetrahedra. On addition of the alkali halides, the presence of Al–Cl–Al linkages gradually decreases in proportion to the concentration of the halide added. This coincides with the formation of [AlCl4]– species. The neutron scattering also shows that the long-range order within the liquid decreases as the binary salt mixture is formed, consistently with the gradual breakdown of the polymeric aluminium trichloride structure. Of significance in the 1:1 binary mixture is the high level of charge ordering in the system. For example, in the case of LiCl, features at r = 6.65, 9.85, and 12.9 Å in the radial distribution function are clearly evident but do not correspond to distances in either of the pure components and therefore are probably associated with spacings between [AlCl4]– units.
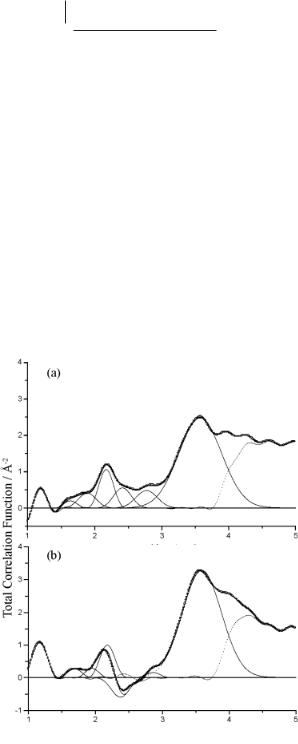
132 Chris Hardacre
Ion pairs |
b6Li bi |
b 7Li bi |
Li-S |
0.004 |
–0.005 |
Li-C |
0.009 |
–0.012 |
Li-N |
0.013 |
–0.017 |
Li-Al |
0.005 |
–0.006 |
Li-Cl |
0.041 |
–0.054 |
|
|
|
Table 4.1-1: Comparison of the neutron scattering crosssections for 6Li and 7Li with all the other atoms present in a binary mixture of LiSCN with AlCl3.
Binary mixtures of LiSCN with AlCl3 have also been studied [7]. In this case, the 1:1 mixture is liquid at ambient temperature and therefore provides a system analogous to room-temperature ionic liquids. Lee et al. used Li isotope substitution to enable the correlations between Li–X to be isolated. The weighting factors between 6Li and 7Li are positive and negative, respectively, and can be used to distinguish features in the partial radial distribution function. Table 4.1-1 compares the combined neutron scattering cross-sections bLibi for both isotopes with each other atom i in the liquid. Figure 4.1-2 shows the equivalent total correlation functions for the 1:1 mixture. Clearly the amplitude of the 7Li systems shows negative and reduced features compared with the 6Li sample, and these must therefore be associated with Li–X features.
r / Å
r / Å
Figure 4.1-2: Total correlation functions for (a) 6LiSCN/AlCl3 and (b) 7LiSCN/AlCl3. The bold lines are the experimental neutron data (•), the fit (–), the Gaussian functions for each of the
atomic pairs used to fit the data (–) and the deviation (......) used. Reproduced
from reference 7 with permission.
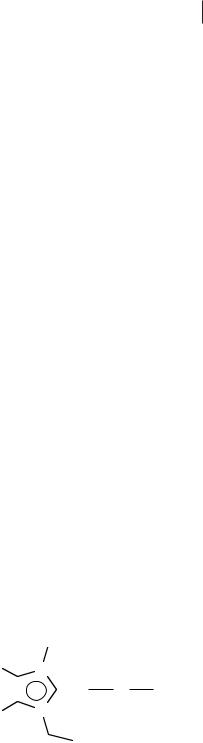
4.1 Order in the Liquid State and Structure 133
From the data, the liquid is shown to have tetrahedrally coordinated aluminium with three chlorines and the isocyanate group attached. The neutron data clearly shows nitrogen, as opposed to sulfur, coordination to the aluminium center, forming an AlCl3NCS– species, which is consistent with a hard base/hard acid interaction as compared with the softer sulfur donation. It was also possible to show that a tetrahedral chloride environment is present around the lithium.
Takahashi et al. have studied the structures of AlCl3/[EMIM]Cl mixtures over a range of concentrations from 46 to 67 mol% AlCl3 [8]. Below 50 mol% AlCl3, the neutron data could be simulated simply by using the isolated ions: that is, [EMIM]+ and [AlCl4]–. Above 50 mol% AlCl3, [Al2Cl7]– is also known to exist, and at 67 mol% AlCl3 becomes the major anion present. Unlike that of [AlCl4]–, the geometry of [Al2Cl7]– is changed substantially in the liquid compared with the isolated ions, implying a direct interaction between the imidazolium cation and the anionic species. This is manifested as a decrease in the torsion angle around the central Al–Cl–Al axis, from 57.5o to 26.2o, and hence as a decrease in the Cl–Cl distance across the anion.
Mixtures of HCl and [EMIM]Cl have also been studied [9, 10]. By analysis of the first order differences by hydrogen/deuterium substitution both on the imidazolium ring and the HCl, two intramolecular peaks were observed. These indicated the presence of [HCl2]– as an asymmetric species, which, coupled with analysis of the second order differences, allowed the structure in Figure 4.1-3 to be proposed.
4.1.5.2Simple salts
Bowron et al. [11] have performed neutron diffraction experiments on 1,3- dimethylimidazolium chloride ([MMIM]Cl) in order to model the imidazolium room-temperature ionic liquids. The total structure factors, F(Q), for five 1,3- dimethylimidazolium chloride melts – fully protiated, fully deuterated, a 1:1 fully deuterated/fully protiated mixture, ring deuterated only, and side chain deuterated only – were measured. Figure 4.1-4 shows the probability distribution of chloride around a central imidazolium cation as determined by modeling of the neutron data.
As well as charge-ordering in the system, out to two chloride shells, the specific local structure shows strong interactions between the chloride and the ring hydrogens, as well as some interaction between the methyl groups of adjacent imidazolium cations. This is consistent with the crystal structure and implies that the molecular packing and interactions in the first two or three coordination shells are similar in both the crystal and the liquid.
Figure 4.1-3: Proposed structure of the asymmetric [HCl2]– ion bound to the [EMIM]+ cation in a binary mixture of HCl/[EMIM]Cl. The figure has been redrawn from reference 10 with permission.
H |
N |
|
δ− |
|
|
δ− |
|
+ |
|
H Cl |
H |
|
Cl |
|
|
|
||||
H |
N |
|
|
|
|
|
|
|
|
|
|
|

134 Chris Hardacre
Figure 4.1-4: Chloride probability distribution around a central imidazolium cation as determined by the EPSR model of the neutron data from liquid [MMIM]Cl at 150 °C.
In all these examples, the importance of good simulation and modeling cannot be stressed enough. A variety of methods have been used in this field to simulate the data in the cases studies described above. Blander et al. [4], for example, used a semi-empirical molecular orbital method, MNDO, to calculate the geometries of the free haloaluminate ions and used these as a basis for the modeling of the data by the RPSU model [12]. Badyal et al. [6] used reverse Monte Carlo simulations, whereas Bowron et al. [11] simulated the neutron data from [MMIM]Cl with the Empirical Potential Structure Refinement (EPSR) model [13].
4.1.6
X-ray Diffraction
X-ray diffraction has been used for the study both of simple molten salts and of binary mixtures thereof, as well as for liquid crystalline materials. The scattering process is similar to that described above for neutron diffraction, with the exception that the scattering of the photons arises from the electron density and not the nuclei. The X-ray scattering factor therefore increases with atomic number and the scattering pattern is dominated by the heavy atoms in the sample. Unlike in neutron diffraction, hydrogen (for example) scatters very weakly and its position cannot be determined with any great accuracy.
In contrast with the study of the structure of the molten salts, full analysis of the scattering profile is not generally performed for liquid crystalline materials. In the latter, only the Bragg features are analyzed (that is, for a wavelength λ, incident on the sample at an angle θ to its surface normal, the position of the diffraction peaks are determined by Bragg’s law, nλ = 2dsinθ). From the angle of diffraction, the periodicity length, d, may be determined.

4.1 Order in the Liquid State and Structure 135
In both cases, laboratory X-ray sources may be used and the X-ray measurements taken in θ–2θ geometry. For weakly scattering systems synchrotron radiation is helpful.
4.1.6.1Cells for liquid samples
Sample cells include Lindemann/capillary tubes (normally < 1 mm in diameter) and aluminium holders. In the latter, thin aluminium windows sandwich the sample in a cylindrical aluminium sample holder. The diffraction from the aluminium is observed in this case, and may be used as a calibration standard. For low-tem- perature materials, the aluminium window can be replaced by the polymer Kapton. Beryllium may also be used [14]. Sample volumes of between 50 and 100 µL are typically required.
4.1.6.2Examples
Molten salts and binary mixtures X-ray diffraction has been performed by Takahashi et al. [15] on 1:1 binary mixtures of AlCl3 with LiCl and NaCl. In agreement with the neutron data obtained by Badyal et al. [6] and discussed above, the liquid has a degree of charge ordering, with sets of four [AlCl4]– units surrounding a central [AlCl4]– unit at distances of 6.75 Å for LiCl and 6.98 Å for NaCl. Similarly, Igarashi et al. [16] have studied a molten LiF/NaF/KF eutectic mixture. For the ionpairs Li–F, Na–F, and K–F, the nearest-neighbor coordination and distances were almost identical to those found in the individual melts of the component salts.
Binary mixtures that melt close to room temperature, namely AlCl3/N-butylpyri- dinium chloride mixtures, have also been investigated. Takahashi et al. [17] have also shown that for the 1:1 composition, [AlCl4]– predominates with a tetrahedral environment. At a ratio of 2:1, [Al2Cl7]– becomes the main species. At high temperature (above 150 oC), some decomposition to [AlCl4]– and Al2Cl6 was observed.
Liquid crystals A wide range of ionic liquids form liquid-crystalline phases. This is normally achieved by increasing the amphiphilic character of the cation through substitution with longer, linear alkyl groups. The salts have relatively low melting points, close to room temperature when the alkyl chain length (Cn) is small (n < 10), and display liquid crystal mesomorphism when n > 12. This section describes some of the results of studies in which X-ray diffraction has been used to examine the mesophase and liquid phase. There are also many examples of materials which form liquid-crystalline phases that have been studied by techniques such as NMR, DSC, single-crystal X-ray diffraction, and so on that have not been included (see, for example, [18–21].
Metal-containing systems
Many of the systems studied are based on [MCl4]2– anion. Neve et al. have extensively studied the formation of liquid-crystalline phases of N-alkylpyridinium salts with alkyl chain lengths of n = 12–18 with tetrahalometalate anions based upon Pd(II) [22] and Cu(II) [23]. In general, the liquid-crystalline phases exhibit lamellar-