
Multiple Bonds Between Metal Atoms / 09-Ruthenium Compounds
.pdf
Ruthenium Compounds 387
Angaridis
A detailed theoretical analysis of the electronic structure of Ru2 tetracarboxylates reported by Norman and coworkers in the late 1970s provided an interpretation of the above experimental data.59,60 SCF-X_-SW calculations performed for Ru2(O2CH)4, [Ru2(O2CH)4]+, and [Ru2(O2CH)4Cl2]-, (HCO2- was used as a model of a carboxylate bridge) showed that Ru25+ tetracarboxylates do not exhibit the electronic configuration μ2/4β2β*2/* which is common for other dimetal complexes. Instead, the /* and β* molecular orbital levels are very close in energy (almost degenerate), regardless of the identity of the axial ligands and the use of a spinrestricted or spin-unrestricted model, giving rise to the electronic configuration μ2/4β2(/*β*)3 (Fig. 9.4). The fact that these almost degenerate /* and β* molecular orbitals are half-filled may be the reason for the higher stability of the Ru25+ tetracarboxylates compared to their Ru24+ analogs.
Fig. 9.4. The theoretically calculated molecular orbital energies of Ru2(O2CH)4 and [Ru2(O2CH)4]+ using the SCF-X_-SW method.
Based on this electronic structure, assignments of the bands in the electronic1,47 and resonance Raman spectra61 were suggested by Norman.60 In solution all Ru25+ tetracarboxylates exhibit a strong band at 21,000-22,000 cm-1 which does not show any dependence on the alkyl substituent, R, of the carboxylate bridge, and a weak band at ~9,000 cm-1. The former band, which was originally proposed to be a βΑβ* transition, has been reassigned to a charge transfer /(Ru–O, Ru2) Α /*(Ru2) transition, with the / level having ~75% Ru–O / character, while the weak near-IR band has been assigned to the β(Ru2) Α β*(Ru2) transition. Experiments using single crystal polarized optical spectroscopy and other studies supported Norman’s assignments.62 By using resonance Raman spectroscopy, Clark and Ferris showed that the band at 21,000-22,000 cm-1 is a dipole-allowed z-polarized /Α/* transition,63 while, Gray and Miskowski by using single crystal polarized optical spectroscopy provided evidence that the band at ~9,000 cm-1 is a z-polarized βΑβ* transition.64 The rest of the electronic absorption
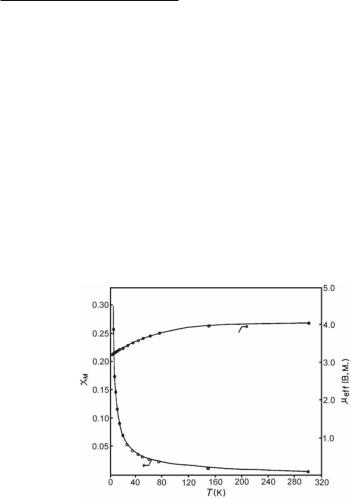
388Multiple Bonds Between Metal Atoms Chapter 9
spectra of the Ru25+ tetracarboxylate compounds has been examined in great detail by Gray and Miskowski.65
Early variable temperature magnetic susceptibility measurements for Ru2(O2CPrn)4Cl showed that this compound exhibits Curie-Weiss behavior in the temperature range 35-300 K, but at temperatures below 35 K there is a deviation (Fig. 9.5).48,66 Due to the polymeric chain structure of this compound, its magnetic behavior at lower temperatures was originally attributed to a combination of a contribution from antiferromagnetic exchange between the Ru25+ units and a large zero-field splitting. However, attempts to model the results of these magnetic measurements led to the conclusion that the system can be better modeled as if there is no intermolecular antiferromagnetic exchange but only a large zero-field splitting (a value ~70 cm-1 was calculated for the zero-field splitting parameter, D). Therefore, each of the [Ru2(O2CPrn)4]+ units of the polymeric chain behaves as an independent unit with S = 3/2. This can be explained in terms of the bent polymeric chain structure of the compound, Ru–Cl–Ru ~125º, which does not allow optimum orbital overlap between the paramagnetic Ru25+ units and the Cl- linkers necessary for sufficient intermolecular antiferromagnetic interaction.
Fig. 9.5. Plots of the temperature dependence of molar magnetic susceptibility and effective magnetic moment for Ru2(O2CPrn)4Cl.
In contrast to Ru2(O2CPrn)4Cl, variable temperature magnetic susceptibility measurements for Ru2(O2CCMePh2)4Cl, which exhibits a linear polymeric chain structure withRu–Cl–Ru = 180º, show that at temperatures below 70 K together with a large zerofield splitting, an interdimer antiferromagnetic coupling also exists with a coupling constant J = -10 cm-1, revealing that the geometry of the polymeric structure of Ru2(O2CR)4Cl compounds affects their magnetic behavior.67 A correlation between the Ru–Cl–Ru and the magnitude of the interdimer antiferromagnetic coupling has been proposed, according to which the latter increases as the Ru–Cl–Ru approaches 180º and becomes smaller for more acute angles.68 Indeed, for the compounds Ru2(O2CEt)4Cl, Ru2(O2CC(Me)=CHEt)4Cl and Ru2(O2CCMePh)4Cl, in which Ru–Cl–Ru ~180º, together with a large zero-field splitting (D ~70 cm-1) there is an antiferromagnetic exchange interaction between the Ru25+ units with coupling constants ranging from -8 to -13 cm-1,69 while in the compounds Ru2(O2CBun)4Cl and Ru2(O2C-n-C7H15)4Cl in which 125º < Ru–Cl–Ru < 180º, the coupling is weaker with coupling constants -1 and -5 cm-1, respectively.68
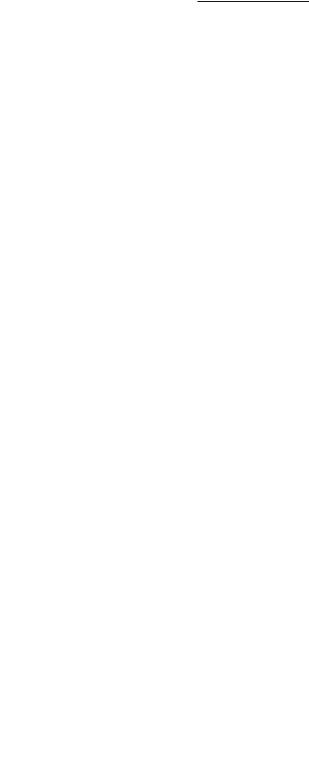
Ruthenium Compounds 389
Angaridis
In the case of polymeric Ru25+ tetracarboxylate compounds with linear or slightly bent chain structures linked by ligands other than halides, like [Ru2(O2CMe)4(pyz)]BPh4,70 [Ru2(O2CMe)4(4,4'-dipy)]PF6,71 [Ru2(O2CMe)4(dabco)]PF6,71 and [Ru2(µ-O2CMe)4](N(CN)2),42 a small, but not negligible, degree of interdimer antiferromagnetic coupling is also suggested to exist (the coupling constants range from -1 to -3 cm-1) together with a large zero-field splitting. In compounds of this type the axial ligand linking the Ru25+ units is of great importance, since the antiferromagnetic coupling effect becomes less important when longer linkers are used, as there is a lengthening of the distance between the interacting Ru25+ units.
A strong dependence of the magnetic behavior on the axial ligands is also observed in the three-dimensional coordination polymers [Ru2(O2CMe)4]3[M(CN)6] (M = Cr, Fe, and Co) with a Prussian blue type of structure.44 The data for the compound with the diamagnetic [Co(CN)6]3- linker show that there are no interactions between the paramagnetic Ru25+ units (a value of 0 K was calculated for the Weiss constant, ε). For the [Cr(CN)6]3- analog, there are antiferromagnetic interactions between the adjacent spin sites (ε ~ -40 K). However, for the paramagnetic linker [Fe(CN)6]3- the data suggest that there are ferromagnetic interactions between the adjacent Ru25+ units with ε ~0.7 K, while at 8 K a transition from short range ferromagnetic interactions to long range magnetic ordering takes place.
For the Ru25+ tetracarboxylates with non-polymeric structures the situation is simpler, because the Ru25+ units are not connected together and no coupling between them is expected. Indeed, measurements on the diadduct [Ru2(O2CCHMePh)4(H2O)2]BPh4 over the temperature range 6-300 K fit a model involving only a large zero-field splitting (D ~70 cm-1) and no intermolecular antiferromagnetic coupling.70 However, a recent study of the magnetic properties of the non-polymeric Ru2(O2CBut)4Cl and Ru2(O2CC4H4N)4Cl indicates that these complexes exhibit a weak intermolecular antiferromagnetic coupling, which is not associated with spinexchange between adjacent Ru25+ units as in the polymeric compounds (since these are not bridged by a linker), but allowed by a through-space pathway.72
The magnetic properties of Ru25+ carboxylate compounds with axially coordinated nitroxide radicals, such as tempo,73 nitme,74,75 nitet,74 nitph,18,76,77 p-pynit78,79 and m-pynit79 are of special interest, since these compounds exhibit two types of magnetic interactions: between the two axially coordinated radicals through the dimetal core (with coupling constant J1) and between the dimetal core and the radicals (with coupling constant J2) as shown in 9.3. For the discrete dimer [Ru2(O2CBut)4(nitme)2]BF4 both ferromagnetic interactions between the Ru2 core and the nitme ligands (J2 = 5 cm-1) and antiferromagnetic interactions between the nitme ligands (J1 = -40 cm-1) are observed.74 In contrast, in [Ru2(O2CBut)4(tempo)2][Ru2(O2CBut)4- (H2O)2](BF4)2 the cation [Ru2(O2CBut)4(tempo)2]+ exhibits only a large antiferromagnetic interaction between the Ru2 core and the nitroxide radical with J2 = -130 cm-1, and no coupling between the two axial nitroxide ligands (J1 = 0).73 In the cases of the polymeric chain compounds [Ru2(O2CBut)4(nitph)]BF418 and [Ru2(O2CBut)4(p-pynit)]BF478 only magnetic interactions between the Ru2 units and the nitme ligands are observed, with J2 coupling constants -100 and 20 cm-1, respectively. In the last two polymeric compounds only localized coupling is observed.
9.3

390Multiple Bonds Between Metal Atoms Chapter 9
O,O'-donor bridging ligands other than carboxylates
Other non-carboxylate-type O,O'-donor bridging ligands that have also been used for the synthesis of Ru25+ paddlewheel compounds include CO32-, SO42-, HnPO4-3+n, and hedp.
The polymeric compound K3[Ru2(O2CO)4]·6H2O was first reported to be synthesized from Ru2(O2CMe)4 and an excess of K2CO3 in H2O according to the disproportionation reaction:
5Ru2(O2CMe)4 + 16K2CO3 Α 2Ru + 4K3[Ru2(O2CO)4]·6H2O + 20K(O2CMe).80
Na3[Ru2(O2CO)4]·6H2O was made from K3[Ru2(O2CO)4]·6H2O by ion exchange. However, although the room temperature magnetic moments of these two compounds (~ 4.1 BM) suggest that they are Ru25+ species, the precise oxidation state of the Ru2 unit could not be inferred from the crystallographic data (for the Na+ salt, the formula appears to be Na3.5[Ru2(O2CO)4]·6H2O).
Alternatively, M3[Ru2(O2CO)4]·xH2O compounds (M = Na and x = 6, M = K and x = 4), respectively) have been synthesized from the ligand substitution reaction of Ru2(O2CMe)4Cl with excess of M2CO3 in H2O:
Ru2(O2CMe)4Cl + 4M2CO3 Α M3[Ru2(O2CO)4]·xH2O + 4M(O2CMe) + MCl.81
The crystal structures of the compounds obtained by this method were accurately determined as K3[Ru2(O2CO)4]·4H2O and Na3[Ru2(O2CO)4]·6H2O, establishing in this way unequivocally the oxidation states of the metal and the stoichiometries. They show that each one of the Ru2(O2CO)4 units participates with two O atoms in the formation of axial bonds with two neighboring Ru2(O2CO)4 units resulting in two-dimensional layers (Fig. 9.6). The average Ru–Ru bond length of 2.251 Å (Table 9.1) falls in the range of Ru25+ tetracarboxylates and is consistent with the μ2/4β2(/*β*)3 electronic configuration.
These Ru25+ tetracarbonates have been used as starting materials for the preparation of K3[Ru2(SO4)4(H2O)2]·2H2O and K4[Ru2(HPO4)3(PO4)(H2O)2].82 Although the former was originally synthesized by a different synthetic method which involved the reaction of [Ru2(O2CMe)4]+ with H2SO4,83 the utilization of [Ru2(O2CO)4]3- offers a fast, convenient and high-yield synthesis due to the ease of expelling CO2 in acidic media. The crystal structure of [Ru2(SO4)4(H2O)2]3-, determined as K2H[Ru2(SO4)4(H2O)2]·4H2O, shows the expected paddlewheel structure similar to those of Mo, Rh, and Pt compounds,83 while that of K4[Ru2(HPO4)3- (PO4)(H2O)2] reveals the existence of a three-dimensional network in which the dimetal units are held together by H-bonds between the axially coordinating H2O molecules and O atoms of the bridging ligands.82 The Ru–Ru bond lengths of 2.303(1) and 2.305(1) Å for K2H[Ru2(SO4)4- (H2O)2]·4H2O and K4[Ru2(HPO4)3(PO4)(H2O)2], respectively (Table 9.1), are slightly longer than the corresponding Ru–Ru distances in Ru25+ tetracarboxylates and tetracarbonates, but they are still in the typical range for Ru25+ compounds with three unpaired electrons and the μ2/4β2(/*β*)3 electronic configuration, as it is indicated by the room temperature magnetic moment of K3[Ru2(SO4)4(H2O)2]·2H2O.82,84
The related Ru25+ compound with bridging phosphonate ligands (NH4)3[Ru2(hedp)2]·2H2O has been synthesized by the hydrothermal reaction between RuCl3·xH2O and H4hedp·H2O.85 It exhibits a two-dimensional layer structure similar to those of K3[Ru2(O2CO)4]·4H2O and Na3[Ru2(O2CO)4]·6H2O, with a Ru–Ru bond length of 2.347(1) Å (Table 9.1). This distance is much longer than the distances for most Ru25+ compounds, due to the larger “bite” of the phosphonate ligands. Magnetic susceptibility measurements show that its room temperature magnetic moment is ~4.2 BM per dimer, which implies the presence of Ru25+ units with three unpaired electrons, while at low temperatures weak ferromagnetic interactions between the Ru25+ units within each layer are observed.
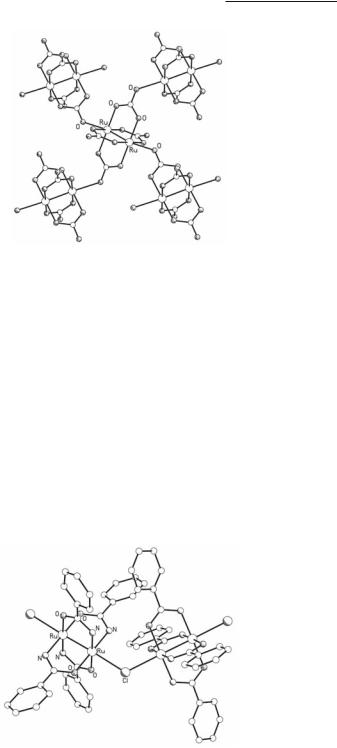
Ruthenium Compounds 391
Angaridis
Fig. 9.6. The structure of the polymeric anion in Na3[Ru2(O2CO)4].
9.2.2 Ru25+ compounds with N,O-donor bridging ligands
Amidate ligands
The Ru25+ tetraamidates are synthesized from the substitution reactions of Ru2(O2CMe)4Cl with excess of molten amides (RCONH2) at elevated temperatures.86 Attempts to synthesize such compounds from reactions in MeOH/H2O mixtures have been unsuccessful.
Structural data, although they are limited since only four compounds have been crystallographically characterized, show that in the solid state Ru25+ tetraamidates exhibit two structural types. Compounds of the type Ru2(ONHCR)4Cl show polymeric structures similar to the Ru25+ tetracarboxylate analogs, in which the [Ru2(ONHCR)4]+ units form infinite zig-zag chains through axial Cl- ions, as in Ru2(ONHCPh)4Cl87 (Fig. 9.7) and Ru2(ONHCC6H4-p-Cl)4Cl.88 There are also discrete diadducts of the type [Ru2(ONHCR)4L2]Y, as in the compounds [Ru2(ONHCC6H4-p-But)4(OPPh3)2]BF489 and [Ru2(ONHCC4H3S)4(THF)2]SbF6.90 The Ru–Ru distances are between 2.281 and 2.296 Å (Table 9.1). Similarly to the carboxylate analogs, the diadducts exhibit shorter distances than the polymeric compounds.
Fig. 9.7. Part of the polymeric zig-zag chain structure of Ru2(ONHCPh)4Cl.
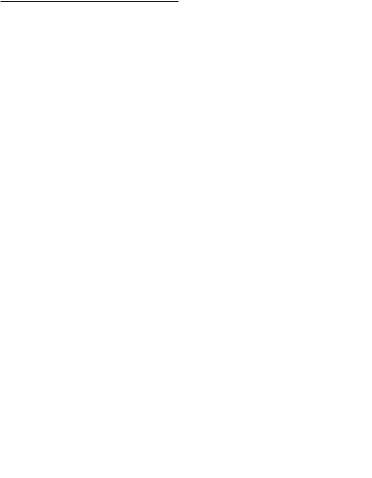
392Multiple Bonds Between Metal Atoms Chapter 9
Since amidates have two different donor atoms, N and O, the arrangement of four such ligands around the Ru25+ unit can give rise to four possible regioisomers as shown in Fig. 1.10. So far, only non-polar trans-(2,2) regioisomers have been isolated.
The Ru2(ONHCR)4Cl compounds react with Ag+ reagents in the presence of coordinating solvents or other suitable ligands to give diadducts, such as [Ru2(ONHCC4H3S)4(THF)2]SbF6.90 The axial Ru–Cl bonds can also be cleaved by strong polar solvents, such as DMSO.
A few unusual reactions of Ru25+ tetraamidates with phosphines have been reported. When the substituent R group of the bridging amidate ligand is a phenyl or aryl group, the phosphine undergoes metal-assisted P–C bond cleavage, resulting in the transfer of a phenyl (or aryl) group from the phosphine to the Ru atoms to give edge-sharing bioctahedral Ru(III)Ru(III) compounds. For example, the reaction of Ru2(ONHCPh)4Cl with Ph3P gives Ru2(ONHCPh)2- (Ph)2[Ph2POC(Ph)N]2 (9.4a), in which the metal atoms are in a slightly distorted octahedral environment at a distance of 2.566(1) Å from each other, indicative of a Ru–Ru single bond.91 Similar reactions occur between Ru2(ONHCPh)4Cl and (p-Me-C6H4)3P, and Ru2(ONHCC6H3- 3,5-(MeO)2)4Cl and Ph3P, in which the Ru–Ru distances of the resulting compounds are 2.570(2) Å and 2.567[1] Å, respectively.92 In another case, reaction of Ru2(ONHCPh)4Cl and Li(ap) followed by addition of PMe2Ph results in the edge-sharing bioctahedral complex 9.4b which has a Ru–Ru single bond of 2.573(2) Å.93
9.4
Electrochemical experiments for Ru2(ONHCCF3)4Cl in different solvents, and using various concentrations of Cl- ions show a medium-dependent reduction process, similarly to Ru2(O2CPrn)4Cl.86 While in strongly coordinating solvents, or in the presence of excess of Cl- ions, a single one-electron reduction process is observed at mild potentials (0.0 to -0.35 V), in non-coordinating solvents twoor three-step reduction processes occur. These are attributed to the association equilibria between [Ru2(ONHCCF3)4]+, Cl- ions and solvent molecules.
Of interest is the electrochemical behavior of Ru2(ONHCMe)4Cl.94 The cyclic and the differential pulse voltammograms in DMSO using LiCl as electrolyte (Fig. 9.8) show three oneelectron processes, one oxidation (+0.47 V) and two reductions (-0.96 and -1.22 V vs SCE). These have been assigned to the processes [Ru2(ONHCMe)4]+ Α [Ru2(ONHCMe)4]2+ + e-, [Ru2(ONHCMe)4]+ + e- Α Ru2(ONHCMe)4, and Ru2(ONHCMe)4 + e- Α [Ru2(ONHCMe)4]-, respectively. The first reduction process described by the second equation, typically occurs at less negative potentials than for Ru2(O2CR)4Cl and other Ru2(ONHCR)4Cl compounds. This substantial cathodic shift is attributed to the high basicity of the MeCONH ligand compared to the others, and this is probably responsible for the observation of the oxidation process described by the first equation. An explanation of the exact nature of the doubly reduced species in the second reduction process has not been given. An oxidation process is also observed in

Ruthenium Compounds 393
Angaridis
Ru2(ONHCCMe3)4Cl, which also shows a complex redox behavior with a dependence on solvent and concentration of Cl- ions.95
Fig. 9.8. Cyclic voltammogram (top) and differential pulse voltammogram (bottom) of Ru2(ONHCMe)4Cl in DMSO.
Room temperature magnetic susceptibility measurements of Ru25+ tetraamidates show magnetic moments of ~4.0 BM per Ru25+ unit, which are indicative of a quartet ground state and suggest the μ2/4β2(/*β*)3 electronic configuration. Structural data give support to this electronic configuration. The Ru–Ru bond distances in Ru25+ tetraamidates are similar to those for the Ru25+ teracarboxylates, for which it is known that they exhibit a quartet state and the μ2/4β2(/*β*)3 electronic configuration. Any change from the quartet state would entail a change in the number of /* electrons. Since the number of /* electrons has a large effect on the Ru–Ru bond length, any compound that does not have a quartet ground state ought to display a Ru–Ru bond distance outside of the range observed for Ru25+ tetracarboxylates. Conversely, all Ru25+ compounds with Ru–Ru bond lengths in that range may be presumed to have the μ2/4β2(/*β*)3 electronic configuration.
Variable temperature magnetic susceptibility measurements for polymeric compounds Ru2(ONHCR)4Cl and discrete diadducts [Ru2(ONHCR)4L2]Y show Curie-Weiss behavior over the temperature range of 70-290 K, which is consistent with three unpaired electrons.89,90 However, below 70 K a deviation from the Curie-Weiss behavior is observed, which is attributed to a large zero-field splitting (D ~ 45-70 cm-1) together with a weak, but not negligible, antiferromagnetic coupling with coupling constants ranging form -0.1 to -2.9 cm-1. It has been proposed that in the polymeric compounds the coupling takes place through the bridging Cl- ions, while in the diadducts a through-space antiferromagnetic interaction is present, similar to some of the non-polymeric Ru25+ tetracarboxylates.72
Oxopyridinate ligands
The first structurally characterized Ru25+ tetraoxopyridinate complex, Ru2(hp)4Cl(Hhp) (Fig. 9.9), was synthesized by reacting Ru2(O2CMe)4Cl with an excess of molten Hhp.96 A few other compounds of this type have been prepared in a similar way. By careful temperature control of these reactions, partial substitution of the acetate groups by Xhp ligands can be accomplished and complexes of the general type Ru2(O2CMe)4-x(Xhp)xCl (x = 1, 2, 3) can be synthesized. An example is the synthesis of Ru2(O2CMe)(chp)3Cl, which is obtained from the reaction of Ru2(O2CMe)4Cl with Hchp in boiling MeOH.97
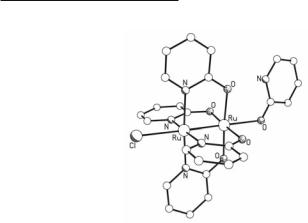
394Multiple Bonds Between Metal Atoms Chapter 9
Fig. 9.9. The structure of Ru2(hp)4Cl(Hhp).
In the solid state, the majority of Ru25+ tetraoxopyridinates exist as discrete paddlewheel complexes. However, there are a few cases in which association occurs, as in [Ru2(chp)4]2(BF4)2 which crystallizes as a dimer with bonds between the O atom of one Ru2(chp)4 unit and the axial position of the other and vice versa (Fig. 9.10).98
The Ru–Ru bond lengths (Table 9.1) lie in the narrow range of 2.254-2.286 Å, similar to the Ru25+ tetracarboxylates, and they do not show any dependence on the substituents of the bridging Xhp ligands. However, they are affected by the axial ligands, since complexes without axial ligands exhibit shorter Ru–Ru bond lengths than those with axial ligands, as shown in [Ru2(chp)4]2(BF4)2 and Ru2(chp)4Cl, in which the Ru–Ru distances are 2.254(1) and 2.281(1) Å, respectively.
Although all possible regioisomers are known for tetraoxopyridinate complexes of other metals, for Ru2(Xhp)4Cl complexes only the polar (4,0) arrangement has been observed, possibly due to the strong preference of the Ru2 complexes for axial coordination. In the polar arrangement (4,0) the preference for axial coordination is accommodated, since all the X groups of the bridging Xhp ligands are placed at one axial site leaving the other one unencumbered for the formation of an axial Ru–Cl bond. A second factor which plays an important role in determining the structures of the Ru25+ tetraoxopyridinates is the bulk of the X groups. For X groups with small steric demand, e.g., X = H or F, the polar arrangement is adopted with the bridging Xhp ligands in an eclipsed conformation.96,99 When X is larger, e.g., X = Cl, in order to relieve the repulsions between the Cl atoms which are in close proximity a twist of the Xhp ligands is induced (torsion angle ~19º).100,101 For the bulkier Me groups, the polar arrangement would result in so great a twist of the bridging ligands that all attempts to prepare a polar complex Ru2(mhp)4Cl have been unsuccessful. Only the complex trans-Ru2(O2CMe)2(mhp)2Cl has been isolated, which has two mhp ligands oriented in the same direction (Fig. 9.11).102
Reactions of Ru2(Xhp)4Cl complexes with Ag+ reagents result in the removal of the axial halide leaving an open position available for coordination by suitable ligands, L (e.g., THF, pyridine, CF3SO3-), and forming monoadducts of the general type [Ru2(Xhp)4L]+. Diadducts do not form because the polar arrangement of the bridging Xhp ligands makes the second axial site inaccessible.103,104 Upon reactions with /-acceptor reagents, such as PMe3 or CNC6H11, mononuclear decomposition products are obtained.105 However, in the case of reactions with Me3SnC>CPh, the paddlewheel structure is retained (even with excess of Me3SnC>CPh), and mono-alkynyl Ru25+ complexes are isolated.106
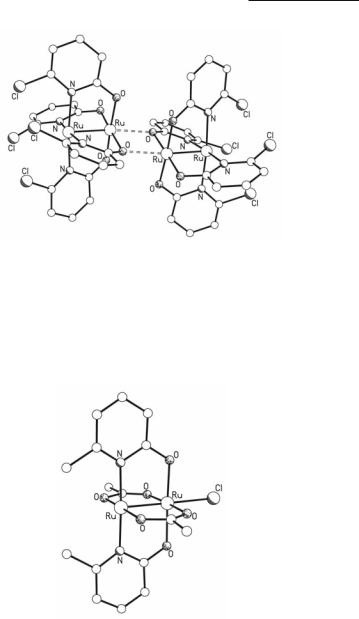
Ruthenium Compounds 395
Angaridis
Fig. 9.10. The structure of the cation in [Ru2(chp)4]2(BF4)2.
Cyclic voltammetry measurements of Ru2(Xhp)4Cl compounds show two metal-centered redox processes: a one-electron oxidation which corresponds to Ru25+ Α Ru26+ + e- and a oneelectron reduction which corresponds to Ru25+ + e- Α Ru24+.99,102,107 The electrochemical behavior of the Ru25+ oxopyridinates is similar to that of Ru25+ carboxylate and amidate analogs, which indicates that the polar arrangement of the Xhp ligands does not result in any significant electronic differences.
Fig. 9.11. The structure of trans-Ru2(O2CMe)2(mhp)2Cl.
Magnetic data for Ru25+ tetraoxopyridinates are very limited. An early magnetic measurement conducted for Ru2(hp)4Cl(Hhp) showed a room temperature magnetic moment of ~4.6 BM, which is indicative of three unpaired electrons, and the μ2/4β2(/*β*)3 electronic configuration was proposed.96 Structural data support this electronic configuration, as the Ru–Ru bond lengths of Ru25+ tetraoxopyridinates fall in the same range with those reported for Ru25+ tetracarboxylates.
Theonlyavailablevariabletemperaturemagneticsusceptibilitystudyisfor [Ru2(chp)4(py)]BF4 and {[(chp)4Ru2](µ-pyz)[Ru2(chp)4]}(BF4)2, in which two [Ru2(chp)4]+ units are linked by a pyz

396Multiple Bonds Between Metal Atoms Chapter 9
molecule (Fig. 9.12).104 Both complexes exhibit similar magnetic behavior: Curie-Weiss behavior in the temperature range 70-300 K with room temperature magnetic moments ~4.0 BM, and a decrease in their magnetic moments below 70 K, attributed primarily to the zero-field splitting effect. Due to this similarity, it was proposed that in [(chp)4Ru2](µ-pyz)[Ru2(chp)4]}(BF4)2 there are no significant magnetic exchange interactions between the Ru25+ units.
Fig. 9.12. The structure of the cation in {[(chp)4Ru2](µ-pyz)[Ru2(chp)4]}(BF4)2.
9.2.3 Ru25+ compounds with N,N'-donor bridging ligands
Aminopyridinate ligands
The Ru25+ tetraaminopyridinates are synthesized by reacting Ru2(O2CMe)4Cl with excess of molten aminopyridines (HXap) at elevated temperatures. By careful control of the experimental conditions of these reactions complexes with a mixed set of aminopyridinate/acetate ligands can be selectively synthesized.97,108 For example, by reacting Ru2(O2CMe)4Cl with excess of Hadmp in MeOH at room temperature the mono-substituted complex is obtained, while a second admp ligand is introduced when the reaction takes place in boiling THF; further substitution is carried out at higher temperatures using molten Hadmp.
In the solid state, Ru25+ aminopyridinates exist as discrete molecules, as it is shown by the crystal structure of Ru2(ap)4Cl in Fig. 9.13.96 Ru2(O2CMe)3(admp)Cl is an exception, as it dimerizes due to the interaction of an O atom of a bridging acetate ligand with the axial position of the Ru25+ unit of a neighboring molecule and vice versa.108
Fig. 9.13. The structure of Ru2(ap)4Cl.