
- •Preface
- •Contents
- •1 Nonideal plasma. Basic concepts
- •1.1 Interparticle interactions. Criteria of nonideality
- •1.1.1 Interparticle interactions
- •1.1.2 Coulomb interaction. Nonideality parameter
- •1.1.4 Compound particles in plasma
- •1.2.2 Metal plasma
- •1.2.3 Plasma of hydrogen and inert gases
- •1.2.4 Plasma with multiply charged ions
- •1.2.5 Dusty plasmas
- •1.2.6 Nonneutral plasmas
- •References
- •2.1 Plasma heating in furnaces
- •2.1.1 Measurement of electrical conductivity and thermoelectromotive force
- •2.1.2 Optical absorption measurements.
- •2.1.3 Density measurements.
- •2.1.4 Sound velocity measurements
- •2.2 Isobaric Joule heating
- •2.2.1 Isobaric heating in a capillary
- •2.2.2 Exploding wire method
- •2.3 High–pressure electric discharges
- •References
- •3.1 The principles of dynamic generation and diagnostics of plasma
- •3.2 Dynamic compression of the cesium plasma
- •3.3 Compression of inert gases by powerful shock waves
- •3.4 Isentropic expansion of shock–compressed metals
- •3.5 Generation of superdense plasma in shock waves
- •References
- •4 Ionization equilibrium and thermodynamic properties of weakly ionized plasmas
- •4.1 Partly ionized plasma
- •4.2 Anomalous properties of a metal plasma
- •4.2.1 Physical properties of metal plasma
- •4.2.2 Lowering of the ionization potential
- •4.2.3 Charged clusters
- •4.2.4 Thermodynamics of multiparticle clusters
- •4.3 Lowering of ionization potential and cluster ions in weakly nonideal plasmas
- •4.3.1 Interaction between charged particles and neutrals
- •4.3.2 Molecular and cluster ions
- •4.3.3 Ionization equilibrium in alkali metal plasma
- •4.4 Droplet model of nonideal plasma of metal vapors. Anomalously high electrical conductivity
- •4.4.1 Droplet model of nonideal plasma
- •4.4.2 Ionization equilibrium
- •4.4.3 Calculation of the plasma composition
- •4.5 Metallization of plasma
- •4.5.3 Phase transition in metals
- •References
- •5.1.1 Monte Carlo method
- •5.1.2 Results of calculation
- •5.1.4 Wigner crystallization
- •5.1.5 Integral equations
- •5.1.6 Polarization of compensating background
- •5.1.7 Charge density waves
- •5.1.8 Sum rules
- •5.1.9 Asymptotic expressions
- •5.1.10 OCP ion mixture
- •5.2 Multicomponent plasma. Results of the perturbation theory
- •5.3 Pseudopotential models. Monte Carlo calculations
- •5.3.1 Choice of pseudopotential
- •5.5 Quasiclassical approximation
- •5.6 Density functional method
- •5.7 Quantum Monte Carlo method
- •5.8 Comparison with experiments
- •5.9 On phase transitions in nonideal plasmas
- •References
- •6.1 Electrical conductivity of ideal partially ionized plasma
- •6.1.1 Electrical conductivity of weakly ionized plasma
- •6.2 Electrical conductivity of weakly nonideal plasma
- •6.3 Electrical conductivity of nonideal weakly ionized plasma
- •6.3.1 The density of electron states
- •6.3.2 Electron mobility and electrical conductivity
- •References
- •7 Electrical conductivity of fully ionized plasma
- •7.1 Kinetic equations and the results of asymptotic theories
- •7.2 Electrical conductivity measurement results
- •References
- •8 The optical properties of dense plasma
- •8.1 Optical properties
- •8.2 Basic radiation processes in rarefied atomic plasma
- •8.5 The principle of spectroscopic stability
- •8.6 Continuous spectra of strongly nonideal plasma
- •References
- •9 Metallization of nonideal plasmas
- •9.1 Multiple shock wave compression of condensed dielectrics
- •9.1.1 Planar geometry
- •9.1.2 Cylindrical geometry
- •9.3 Metallization of dielectrics
- •9.3.1 Hydrogen
- •9.3.2 Inert gases
- •9.3.3 Oxygen
- •9.3.4 Sulfur
- •9.3.5 Fullerene
- •9.3.6 Water
- •9.3.7 Dielectrization of metals
- •9.4 Ionization by pressure
- •References
- •10 Nonneutral plasmas
- •10.1.1 Electrons on a surface of liquid He
- •10.1.2 Penning trap
- •10.1.3 Linear Paul trap
- •10.1.4 Storage ring
- •10.2 Strong coupling and Wigner crystallization
- •10.3 Melting of mesoscopic crystals
- •10.4 Coulomb clusters
- •References
- •11 Dusty plasmas
- •11.1 Introduction
- •11.2 Elementary processes in dusty plasmas
- •11.2.1 Charging of dust particles in plasmas (theory)
- •11.2.2 Electrostatic potential around a dust particle
- •11.2.3 Main forces acting on dust particles in plasmas
- •11.2.4 Interaction between dust particles in plasmas
- •11.2.5 Experimental determination of the interaction potential
- •11.2.6 Formation and growth of dust particles
- •11.3 Strongly coupled dusty plasmas and phase transitions
- •11.3.1 Theoretical approaches
- •11.3.2 Experimental investigation of phase transitions in dusty plasmas
- •11.3.3 Dust clusters in plasmas
- •11.4 Oscillations, waves, and instabilities in dusty plasmas
- •11.4.1 Oscillations of individual particles in a sheath region of gas discharges
- •11.4.2 Linear waves and instabilities in weakly coupled dusty plasmas
- •11.4.3 Waves in strongly coupled dusty plasmas
- •11.4.4 Experimental investigation of wave phenomena in dusty plasmas
- •11.5 New directions in experimental research
- •11.5.1 Investigations of dusty plasmas under microgravity conditions
- •11.5.2 External perturbations
- •11.5.3 Dusty plasma of strongly asymmetric particles
- •11.5.4 Dusty plasma at cryogenic temperatures
- •11.5.5 Possible applications of dusty plasmas
- •11.6 Conclusions
- •References
- •Index

154 |
WEAKLY IONIZED PLASMAS |
Table 4.11 “Surface energy” of atom α, heat of vaporization q, and ratio α/q at the triple point (Pogosov and Khrapak 1988)
Parameter |
Li |
Na |
K |
Pb |
Cs |
Fe |
Cu |
|
α, eV |
0.98 |
0.68 |
0.60 |
0.54 |
0.52 |
2.9 |
2.2 |
|
q, eV |
1.6 |
1.1 |
0.93 |
0.85 |
0.82 |
3.5 |
4.4 |
|
α/q |
0.60 |
0.63 |
0.64 |
0.65 |
0.63 |
0.69 |
0.64 |
|
ng0 = An10 exp{−[−gkT ln(p/ps) + αg2/3]/kT }. |
(4.48) |
The pre–exponential factor A, which is usually assumed to be equal to unity, will be determined below, with the aid of the experimental data for the equation of state of saturated vapor.
The condition of plasma neutrality and expression (4.45) for the concentration of charged droplets yield the equations for determining the electron number density,
∞
ne = |
ZngZ = |
Z(nes/ne)Z F (Z2) |
|
g,Z |
|
Z=−∞ |
|
∞ |
|
|
|
|
Z[(nes/ne)Z − (ne/nes)Z ]F (Z2). |
|
|
= |
(4.49) |
Z=0
Because F (Z2) > 0, it follows from (4.49) that the number density ne is always less than the Richardson concentration. The latter corresponds to the equilibrium electron concentration near a plane emitting surface,
nes = 2(h2/2πmkT )−3/2 exp(−W/kT ). |
(4.50) |
4.4.3Calculation of the plasma composition
Equation of state for matter and conservation of mass are p = kT nZg and
g,Z
ρ = M gnZg , respectively. Using the known dependencies of pressure and
g,Z
density on the saturation curve (Vargaftic et al. 1985), now we can determine densities n01 and ne and constant A. For su ciently small complexes, one must include the dependence of the surface tension on the droplet radius,
γ(R) = γ0/(1 + δ/R),
where γ0 is the surface tension of a flat surface. The correction for the curvature δ for alkali metals was calculated in Iakubov et al. (1986). Both quantities, γ0 and δ, depend on temperature. Figure 4.11 gives the calculation results for the quantity g (T ) in saturated cesium vapor (g is the value of g, at which n+g attains maximum).
Figure 4.12 gives the results of the calculation for the electron number density in plasma of Na, K, Rb, and Cs vapors.
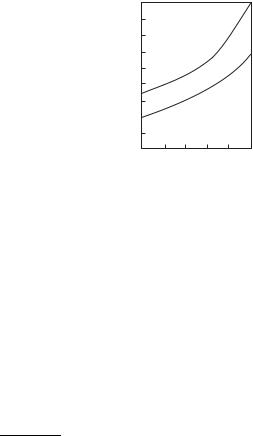
DROPLET MODEL OF NONIDEAL PLASMA OF METAL VAPORS |
155 |
g*
1
10
2
0
13 |
16 |
T, 103 K |
Fig. 4.11. The temperature dependence of g |
in saturated cesium vapor (Pogosov and |
Khrapak 1988): 1 — δ is taken into account, 2 — for δ = 0.
The validity of the droplet model is mainly determined by the values of g (T ) and by the half–width of the distribution n+g (T ). Figure 4.13 shows the normalized distribution of charged complexes for cesium versus the number of atoms, g, for T = 1400 K and T = 1800 K. As the temperature rises, the distribution of droplets over the number of particles becomes broader and the positions of the maxima shift towards larger values of g.
The magnitude of A turned out to be temperature dependent. For cesium, A = 5.3 · 102 at T = 1400 K, and A = 3.3 · 103 at T = 1800 K. Taking into account the very sharp variation of ne(T ), such a dependence, as well as the magnitude of A itself, appears acceptable.
Figure 4.14 gives the results of the calculation and measurements for the specific electrical conductivity of saturated cesium vapors. The electrical con-
ductivity was calculated with the Lorentz formula σ = e2ne/(¯qmvn¯ 01), where
v¯ = 8kT /(πm) and q¯ is the cross section of electron–atom scattering. In highly polarizable media, the density e ects may cause a strong increase in mobility. This is due to the fact that for high densities, the long–range (polarization) components of electron–atom potentials overlap. The resulting potential field is smoothed out and the electron is scattered by the short–range components of potentials (see Chapter 6). Therefore, the cross–section q¯ used by Pogosov and Khrapak (1988) is an order of magnitude smaller than the tabular value.
For the remaining alkali metals, the qualitative picture is similar. Pogosov and Khrapak (1988) do not describe the transition from anomalous to normal electrical conductivity. Based on the results of measurements by Borzhievsky et al. (1988), this transition might occur at T 1200 K. By Zhukhovitskii (1989), yet another version of the droplet model was suggested, with the attempt of a unified description of the vapor equation of state and electrical conductivity, including its transition from the anomalous to normal.