
- •VOLUME 4
- •CONTRIBUTOR LIST
- •PREFACE
- •LIST OF ARTICLES
- •ABBREVIATIONS AND ACRONYMS
- •CONVERSION FACTORS AND UNIT SYMBOLS
- •HYDROCEPHALUS, TOOLS FOR DIAGNOSIS AND TREATMENT OF
- •HYPERALIMENTATION.
- •HYPERBARIC MEDICINE
- •HYPERBARIC OXYGENATION
- •HYPERTENSION.
- •HYPERTHERMIA, INTERSTITIAL
- •HYPERTHERMIA, SYSTEMIC
- •HYPERTHERMIA, ULTRASONIC
- •HYPOTHERMIA.
- •IABP.
- •IMAGE INTENSIFIERS AND FLUOROSCOPY
- •IMAGING, CELLULAR.
- •IMAGING DEVICES
- •IMMUNOLOGICALLY SENSITIVE FIELD–EFFECT TRANSISTORS
- •IMMUNOTHERAPY
- •IMPEDANCE PLETHYSMOGRAPHY
- •IMPEDANCE SPECTROSCOPY
- •IMPLANT, COCHLEAR.
- •INCUBATORS, INFANTS
- •INFANT INCUBATORS.
- •INFUSION PUMPS.
- •INTEGRATED CIRCUIT TEMPERATURE SENSOR
- •INTERFERONS.
- •INTERSTITIAL HYPERTHERMIA.
- •INTRAAORTIC BALLOON PUMP
- •INTRACRANIAL PRESSURE MONITORING.
- •INTRAOCULAR LENSES.
- •INTRAOPERATIVE RADIOTHERAPY.
- •INTRAUTERINE DEVICES (IUDS).
- •INTRAUTERINE SURGICAL TECHNIQUES
- •ION-EXCHANGE CHROMATOGRAPHY.
- •IONIZING RADIATION, BIOLOGICAL EFFECTS OF
- •ION-PAIR CHROMATOGRAPHY.
- •ION–SENSITIVE FIELD-EFFECT TRANSISTORS
- •ISFET.
- •JOINTS, BIOMECHANICS OF
- •JOINT REPLACEMENT.
- •LAPARASCOPIC SURGERY.
- •LARYNGEAL PROSTHETIC DEVICES
- •LASER SURGERY.
- •LASERS, IN MEDICINE.
- •LENSES, CONTACT.
- •LENSES, INTRAOCULAR
- •LIFE SUPPORT.
- •LIGAMENT AND TENDON, PROPERTIES OF
- •LINEAR VARIABLE DIFFERENTIAL TRANSFORMERS
- •LITERATURE, MEDICAL PHYSICS.
- •LITHOTRIPSY
- •LIVER TRANSPLANTATION
- •LONG BONE FRACTURE.
- •LUNG MECHANICS.
- •LUNG PHYSIOLOGY.
- •LUNG SOUNDS
- •LVDT.
- •MAGNETIC RESONANCE IMAGING
- •MAGNETOCARDIOGRAPHY.
- •MANOMETRY, ANORECTAL.
- •MANOMETRY, ESOPHAGEAL.
- •MAMMOGRAPHY
- •MATERIALS, BIOCOMPATIBILITY OF.
- •MATERIALS, PHANTOM, IN RADIOLOGY.
- •MATERIALS, POLYMERIC.
- •MATERIALS, POROUS.
- •MEDICAL EDUCATION, COMPUTERS IN
- •MEDICAL ENGINEERING SOCIETIES AND ORGANIZATIONS
- •MEDICAL GAS ANALYZERS
- •MEDICAL PHOTOGRAPHY.
- •MEDICAL PHYSICS LITERATURE
- •MEDICAL RECORDS, COMPUTERS IN
- •MICROARRAYS
- •MICROBIAL DETECTION SYSTEMS
- •MICROBIOREACTORS
- •MICRODIALYSIS SAMPLING
- •MICROFLUIDICS
- •MICROPOWER FOR MEDICAL APPLICATIONS
- •MICROSCOPY AND SPECTROSCOPY, NEAR-FIELD
- •MICROSCOPY, CONFOCAL
- •MICROSCOPY, ELECTRON
- •MICROSCOPY, FLUORESCENCE
- •MICROSCOPY, SCANNING FORCE
- •MICROSCOPY, SCANNING TUNNELING
- •MICROSURGERY
- •MINIMALLY INVASIVE SURGICAL TECHNOLOGY
- •MOBILITY AIDS
- •MODELS, KINETIC.
- •MONITORING IN ANESTHESIA
- •MONITORING, AMBULATORY.
- •MONITORING, FETAL.
- •MONITORING, HEMODYNAMIC
- •MONITORING, INTRACRANIAL PRESSURE
- •MONITORING, NEONATAL.
- •MONITORING, UMBILICAL ARTERY AND VEIN
- •MONOCLONAL ANTIBODIES
- •MOSFET.
- •MUSCLE ELECTRICAL ACTIVITY.
- •MUSCLE TESTING, REHABILITATION AND.
- •MUSCULOSKELETAL DISABILITIES.
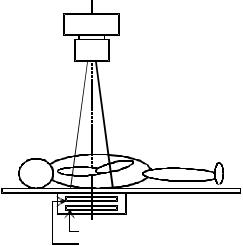
298 MAMMOGRAPHY
field hyperpolarized noble gas MRI. J Magn Reson 2005;174: 116–124.
42.Kvistad KA, et al. Breast lesions: Evaluation with dynamic contrast-enhanced T1-weighted MR imaging and with T2 - weighted first-pass perfusion MR imaging. Radiology 2000; 216:545–553.
43.Kuhl CK, Schild HH. Dynamic interpretation of MRI of the breast. JMRI 2000;12:965–974.
MAGNETOCARDIOGRAPHY. See BIOMAGNETISM.
MANOMETRY, ANORECTAL. See ANORECTAL
MANOMETRY.
MANOMETRY, ESOPHAGEAL. See ESOPHAGEAL
MANOMETRY.
MAMMOGRAPHY
PEI-JAN PAUL LIN
Beth Israel Deaconess
Medical Center
Boston, Massachusetts
INTRODUCTION
It has been shown that reduced breast cancer mortality in the past decade can be attributed to the high sensitivity of screening mammography in detecting nonpalpable lesions (1,2). There has been a wide variety of equipment and imaging modalities employed in the breast cancer detection and imaging; ranging from ultrasound imager, X-ray mammography, computed tomography scanner (CT), to magnetic resonance imager (MRI). Additionally, thermography, light diaphanography, electron radiography, and microwave radiometry have also been utilized, experimentally, to detect breast cancer without much success. Brief explanations of these imaging modalities have been described in the first edition of this Encyclopedia, NCRP Report No.85, and in a review article by Jones (3–5). Among those modalities; ultrasound, X-ray mammography, CT, and MRI, X-ray mammography is the most practical and relatively inexpensive, and is the only main stream of equipment available for breast cancer detection. At present, the ultrasound imager is often employed as an adjunct to the X-ray mammography and is not the primary screening imaging device. However, when mammography is mentioned, it is normally meant to say ‘‘X-ray mammography’’. For these reasons, this article will devote all of its effort to X-ray mammography.
THE MAMMOGRAPHY QUALITY STANDARDS ACT OF 1992 (MQSA), (PUBLIC LAW 102–539)
Breast cancer was a major public health issue in the early 1990s; U.S. Congress enacted MQSA ‘‘to ensure that all women have access to quality mammography for the
detection of breast cancer in its earliest, most treatable stages’’. Thus, it is required by law that facilities providing mammography services be properly accredited and be certified by the U.S. Food and Drug Administration (FDA). ‘‘Accreditation and Certification’’ of mammography facilities are beyond the scope of this article, and interested readers are requested to refer to the FDAs WEB Site (6), and the American College of Radiology (ACR) WEB Site (7).
X-RAY MAMMOGRAPHY
Conventional X-ray equipment was initially employed for breast cancer imaging with industrial (thick) emulsion film, or portal imaging film for use in radiation therapy as the image receptor in order to visualize the small microcalcifications, prior to, as late as 1970s. The breast entrance dose of this imaging process exceeded well over 85 mGy per film ( 10 R per film) and the radiographic techniques were typically in the range of 45–55 kVp, and 1000 mAS with a radiation beam quality of half-value layer (HVL) ¼ 1.0– 1.5 mm of aluminum (mmAl) (8). The X-ray beam spectrum produced by a conventional X-ray tube, equipped with tungsten anode, is not necessarily optimized for breast cancer detection. The mammography images obtained in this manner had the desired spatial resolution ( 20 lp mm 1), but had a less than desirable radiographic contrast. This combination of ‘‘high entrance dose’’ with ‘‘low radiographic contrast’’ was not an acceptable approach. The radiology community was searching for a new breast imaging solution. In the 1970s, xeromammography imaging plates provided the much needed improvement in image quality and lowered the breast entrance dose by a factor of two thirds to one half compared to using the thick emulsion industrial type film (9).
Due to its unique patient positioning of breast imaging, the geometrical arrangement of dedicated mammography units should be pointed out. As shown in Fig. 1, with the
X-ray tube
Collimator
Radiaion field
Patient
Examination table
Screen-film cassette
Anti-scatter grid
Figure 1. Geometric arrangement of conventional radiography. The X-ray system, the anatomy of interest, and the screen-film cassette are centered and aligned for exposure.

Lateral View of Mammography Examination Setup
X-ray tube
Collimator |
Radiaion field
Chest wall
Compression paddle
Breast holder |
Anti-scatter grid
Screen-film cassette
(a) Correct alignment of X-ray |
(b) Improper setup. The "black" |
|
system with the patient's |
||
triangular shaped area is not |
||
chest wall included in the |
||
in the field-of-view. |
||
field-of-view. |
||
|
Figure 2. Geometry of dedicated mammography systems. On the left side of (a), the geometrical arrangement of a dedicated mammography unit is correctly setup where the center of the radiation beam is aligned to the chest wall of the patient with the compression cone pressing down on the breast being imaged. On the right side (b), improper setup for mammography examination is evident. The ‘‘black’’ triangular area is not included in the image captured by the image receptor, potentially missing the suspected cancer site.
conventional radiography system, the X-ray tube is setup in the center of the anatomy of interest. The radiation field is a projection of diverging X rays restricted by the collimator, centered to the area of interest. The geometry of a dedicated mammography system on the other hand is offset so as to maximize inclusion of breast tissue close to the chest wall (see Fig. 2a). If the geometry were setup in the same manner as the conventional radiography as depicted in Fig. 2b, the black triangular area of the breast would not be included in the imaging field possibly missing a suspected cancer site.
THE PHYSICS OF MAMMOGRAPHIC IMAGING
Considering that various tissues in the breast are radiologically similar, but not identical, the task of differentiating the fibrous, ductal, and glandular tissues is extremely difficult. This is due to the fact that (1) water; the main ingredient of human tissue, has a density of r ¼ 1.0 g cm 3
and effective atomic number of Zeff ¼ 7.4–7.6, and (2) fat has a density of ¼ 0.9 g cm 3 and effective atomic number
of Zeff ¼ 5.9–6.5 (10). Thus, various breast tissues with varying degrees of fat and water contents are very similar from radiological point of view.
The development of screen-film mammography was, in reality, paired with the redesigning of dedicated X-ray equipment for breast imaging. Breasts are relatively thin (physical thickness and X-ray attenuation property) compared to other body parts. Radiographs of extremities, for example, are obtained with X-ray tube potential in the
MAMMOGRAPHY 299
range of 50–60 kVp. Breast tissues contain no high attenuation anatomy, such as bones, a lower tube potential (< 35 kVp) would be more suitable for breast imaging (11). Use of lower tube potential has a potential benefit of taking advantage of the photoelectric effect in differentiating the subtle differences of breast tissues.
It should be pointed out that there are five basic ways that X-ray photons interact with matter; they are (1) coherent scattering, (2) photoelectric effect, (3) Compton effect, (4) pair production, and (5) photodisintegration (12). Of these five interactions, photoelectric effect and Compton effect predominantly contribute to the image formation in diagnostic radiology. The probabilities of photoelectric effect and the Compton effect can be expressed as following;
Photoelectric effect Z3=E3 |
ð1Þ |
Compton effect E Z |
ð2Þ |
In equations (1) and (2), E is the photon energy, and Z is the atomic number. Clearly, from Eq. 1, the lower the X- ray photon energy, and the higher the atomic number the probability of the photoelectric effect will be higher. Since the photoelectric effect is proportional to the ‘‘cube’’ of
(Z/E), the differential of breast tissues in Zeff is ‘‘enhanced’’ in the image formation. Thus, a better approach
to differentiate and image the breast tissues is to employ a lower X-ray tube potential for imaging. While this approach is ‘‘good’’ for imaging, the radiation dose absorbed by the breast would still be a major concern.
Conventional X-ray tubes employ tungsten as the target material and produce a broad X-ray spectrum through the bremsstrahlung process. At X-ray tube potential of 35 kVp and lower, the tube potential used on most dedicated mammography systems, the tungsten target X-ray tubes would produce broad energy spectrum that contribute less to the image formation and more to the radiation dose. Tungsten, rhodium, and molybdenum are ideal for use in X-ray production due to their relatively high melting points than other metallic elements. Typically, dedicated mammography equipment is equipped with molybdenum target X-ray tube with beryllium window (port), and 30-mm thick molybdenum filter. The X rays, generated at 25–30 kVp tube potential, produced by the molybdenum target X-ray tube contain a large fraction of characteristic X rays at energies 17–20 keV (13,14). It is, therefore, quite natural to optimize and utilize these characteristic X rays for image formation and, consequently, patient exposure reduction at the same time. Most dedicated mammography equipment employ a combination of molybdenum target with aluminum, molybdenum, or rhodium filters. The characteristic X rays generated at the molybdenum target have X-ray photon energies of 17.4 and 19.6 keV, just below the K-absorption edge of 20 keV, thus are quite transparent to the molybdenum filter. This is illustrated in Fig. 3. On the left of Fig. 3a, is a schematic drawing of the X-ray spectrum generated at 30 kVp with a molybdenum target and aluminum filter. In the middle of Fig. 3b, is the same system with 30 mm thick molybdenum filter. Notice that the K-absorption edge curve (dashed curve) of molybdenum shows that the X-ray photons with energies just above

300 MAMMOGRAPHY
Relative Intensity
(a) Mo Target/Al Filter
1.0
Kα=17.4
0.8
0.6
Kβ=19.6
0.4
0.2
0
10 20 30 Photon Energy (keV)
Relative Intensity
(b) Mo Target/Mo Filter
1.0
0.8
K-edge=20.0
0.6
0.4
0.2
0
10 20 30 Photon Energy (keV)
Relative Intensity
(c) Rh Target/Rh Filter
1.0
Kα=20.2
0.8
Kβ=22.7 K-edge=23.2
0.6
0.4
0.2
0
10 20 30 Photon Energy (keV)
Figure 3. The spectrum of X rays generated with molybdenum and rhodium. On the far left (a) is the X-ray spectrum generated by a molybdenum target at 30 kVp and filtered with aluminum filter. The characteristic X rays Ka (17.4 keV), and Kb (19.6 keV) appear as the two peaks in the graph. In the middle (b) is the X-ray spectrum generated by the same X-ray system in part a, but is filtered with 30 mm thick molybdenum. The K-absorption edge curve is shown in dashed line. On the far right (c) is an X-ray spectrum generated by a rhodium target at 30 kVp and filtered with 20 mm thick rhodium. The general shapes of middle figure and far right figure are similar with differences in the peak energies of K-characteristic X rays (Ka ¼ 20.2 keV, and Kb ¼ 22.7 keV), and the K-absorption edge energy (23.2 keV).
20 keV would be preferentially absorbed than those just below 20 keV. Similarly, the same can be said for the rhodium target with rhodium filter as shown in Fig. 3c. Figure 3b and c are graphically speaking quite similar. It is noteworthy to point out that a careful study of Fig. 3b, and c will reveal that X-ray beams generated from rhodium target X-ray tube ‘‘must not’’ be filtered with molybdenum! The intensity of rhodium K-characteristic X rays (Ka, and Kb) would be in the energy range where the molybdenum K absorption is high. Taking advantage of the spectral information, in 1991,GE introduced the Senographe DMR unit equipped with a dual track and dual filter (molybdenum and rhodium) X-ray tube for mammography applications. Some of the physical characteristics and the spectral energy data of molybdenum, rhodium, and tungsten are summarized in Table 1.
For illustration purposes and descriptions of imaging components, following this paragraph, the screen-film mammography (SFM) system manufactured by GE, the Senogaphe DMR mammography unit, is shown in Fig. 4. The photograph in Fig. 4 represents the overall external
Table 1. K-Characteristic X Rays and K-Absorption Edge of Molybdenum, Rhodium, and Tungsten
|
Molybdenum |
Rhodium |
Tungsten |
|
|
|
|
Atomic Number |
42 |
45 |
74 |
Kaa |
17.4 |
20.2 |
59.3 |
Kba |
19.6 |
22.7 |
69.1 |
K-edgea |
20.0 |
23.7 |
69.5 |
aEnergy in keV.
and mechanical design of a typical ‘‘dedicated’’ X-ray mammography unit. It represents ‘‘the overall’’ design with respect to the tilting gantry with the X-ray tube housing at the top and the image receptor at the bottom. And, the gantry is attached to a column (or stand), which houses the elevation mechanism of entire gantry.
THE SCREEN-FILM MAMMOGRAPHY
The screen-film mammography employs the same basic image receptor system as conventional radiographic imaging. While conventional radiography employs a doubleemulsion film sandwiched between two intensifying \ screens yielding a spatial resolution of (up to) 8 lp mm 1 for a detailed screen (15), a typical SFM image receptor consists of a single-emulsion film and a single thin rareearth phosphor intensifying screen yielding a spatial resolution of 20 lp mm 1 (16).
In order to optimize the efficiency of the SFM, manufacturers including Agfa, Kodak, Konica, and Fuji, have produced matching pairs of the intensifying screen and film specifically for use with mammography. And, to further improve the sensitometric characteristics of the screen-film, the processing chemistry, particularly the developer, have also been carefully prescribed along with its development conditions. Note that the sensitometric characteristics of screen-film system refers to the ‘‘photographic effect or blackening effect’’ of the screen-film system in response to the X-ray absorption (17). An example of this matching pair of intensifying screen and film is depicted in Fig. 5, the emission and absorption

Figure 4. A typical dedicated mammography unit; GE Senographe DMR. (Courtesy of GE Healthcare.) The overall mechanical design of a typical dedicated X-ray mammography showing the tilting gantry with the X-ray tube housing at the top and the image receptor at the bottom. The gantry (or the elongated C arm) is normally attached to a column or a stand in which the elevation mechanism of entire gantry is housed.
characteristics of intensifying screen and film employed in SFM. Note that the emission of 550 nm wavelength light from AD Mammo Screens is matched by the absorption spectra of the AD-M Film.
|
|
|
|
|
MAMMOGRAPHY |
|
301 |
||
|
|
|
Spectral characteristics |
|
|
|
|
||
|
1.0 |
|
|
|
|
|
|
20 |
transmittanceFilter |
sensitivityRelative |
|
|
AD-M film |
|
|
|
|
||
0.5 |
|
|
|
|
|
SLG-8U |
10 |
||
|
|
|
|
|
|
|
|||
|
|
AD mammo screens |
|
|
|
|
|||
|
|
|
|
|
|
|
|||
|
0 |
400 |
450 |
500 |
550 |
600 |
650 |
0 |
|
|
350 |
700 |
|
Wavelength (nm)
Figure 5. Spectral characteristics of screen-film mammography system. The AD Mammo screens are green-emitting and the AD-M film is orthochromatic. The figure shows the light-emitting spectrum of the AD Mammo Screens, the spectral sensitivity curve of the AD-M film, and the transmittance spectrum of SLG-8U safety light filter. (Courtesy of Fujifilm Medical Systems USA, Inc.)
In order to accommodate the varying size of breasts, two imaging cassette sizes are available with dedicated mammography equipment, they are 18 24 cm (8 10 in.), and 24 30 cm (10 12 in.). The film is loaded on the topside of the cassette with the emulsion side facing the intensifying screen. Figure 6 is a schematic drawing showing the crosssection of a typical screen-film mammography cassette (the single emulsion film, single intensifying screen) with enlarged views of the film (top, right), and the screen (bottom, right). The ‘‘sponge’’, in the cassette, is employed to assure good screen-film contact.
THE ANTISCATTER GRID
The antiscatter grid is placed between the breast holder, and the cassette slot, refer to Fig. 4. Scattered radiation is one of the main causes of degrading the image quality in X-ray imaging. The antiscatter grid is employed to minimize the scattered radiation from reaching the screen-film system while allowing the primary radiation to pass through. Although, mammography examinations are typically conducted with X-ray potentials <30 kVp, would still require a moving (reciprocating) antiscatter grid to cleanup the scattered radiation. Typically, the antiscatter grid used in mammography has a grid ratio of 5 : 1, or 4 : 1. While the exposure time in X-ray mammography imaging is relatively long ( 1 s), the speed of the moving grid must be
Protective coating
Antihalation layer
Cassette front
Film base
Film base |
Lower emulsion |
|
Emulsion |
Upper emulsion |
|
Intensifying screen |
Protective coating |
|
|
|
|
Screen base |
Phosphor layer |
|
|
|
|
Sponge |
(Gd2O2:Tb) |
|
Fine grain |
|
|
(for film-screen contact) |
|
|
Highly transparent binder |
|
|
|
|
|
Cassette back |
High packing density |
Figure 6. The cross-sectional view of screen- |
|
film cassette. (Courtesy of Fujifilm Medical |
|
|
Screen base |
|
|
Systems USA, Inc.) |

302 MAMMOGRAPHY
carefully adjusted to avoid artifact associated with grids. For the antiscatter grids, the grid line rate must be sufficiently high, or the attenuation material structure must be so designed to avoid being imaged as grid artifacts on the mammograms. The honeycomb design antiscatter grid; Cellular (HTC) Grid utilized in LoRad mammography systems and marketed by Hologic is well known for its high transmission of useful primary radiation with increased absorption of scattered radiation (18).
THE COMPUTED RADIOGRAPHY FOR MAMMOGRAPHY
Computed radiography was introduced to radiology in 1981 and the ‘‘digitization’’ of routine radiographic examinations had started in earnest. The CR image plate housed in cassette replaced, directly, the screen-film cassette in routine radiography applications. This direct replacement design required no mechanical modifications on the existing radiographic equipment. With the introduction of CR, a whole new set of technology including the optical CR readers, image processing software programs, image display subsystems, and so on, made the filmless radiology department within an achievable reality (19).
The difference between the routine CR and the CR for mammography (CR-M) is largely due to the optical response of the CR phosphor; thus, the radiation dose required to reduce the image noise, and the spatial resolution capability for mammography applications. In 2001, Fuji introduced the FCR 5000MA, which was used in the America College of Radiology Imaging Network (ACRIN); Digital versus Screen-Film Mammography (DMIST) study (20,21). While the study had already been concluded, the official results are in the final preparation stage, and have not been released yet. The FCR 5000MA differentiated itself from the previous generation of CR products by utilizing a 50 mm pixel spot size for the laser and introduced dual side IP reading to the market.
In January 2004, Fuji introduced the Profect CS, or ‘‘ClearView-CS’’ in the United States. Clear View CS is pending FDA approval and not yet commercially available in the United States. Fuji recently finished the Premarketing Approval (PMA) application to FDA, and the approval is anticipated sometime during the second half of 2005 (22). Note, however, that the 100 mm pixel spot size digital mammography system has been in use for the past few years in Europe, Australia, and Japan. The mechanism in which ‘‘how’’ the IP and the CR reader work together to produce an image is beyond the scope of this article and readers are suggested to turn to publications available (23,24), or corresponding articles in this Encyclopedia.
The obvious advantage of CR-M, and the full field digital mammography (FFDM) systems, is its wide dynamic range and its linear response to radiation. In screen-film mammography, over-or underexposure was one of the main causes of ‘‘repeat’’ examinations. Such exposure related repeats can be minimized due to the wide latitude in exposure acceptable for imaging. Furthermore, the images are no longer ‘‘fixed’’ or ‘‘limited’’; the subject contrast and the overall image brightness can be adjusted for image interpretation by adjusting the display window ‘‘level’’, and
|
|
|
Comparison of dynamic range |
|
||||||||||
|
105 |
|
Imaging plate (IP) |
|
|
|
|
|
3 |
Optical |
||||
|
|
|
|
|
|
|
||||||||
|
103 |
|
|
|
|
|
|
|||||||
relativeIP's luminescence |
104 |
|
|
|
|
|
|
|
|
|
|
|
density |
|
101 |
|
|
|
|
|
|
|
|
|
|
|
2 |
||
|
|
|
|
|
|
|
|
|
|
|
|
|||
|
102 |
|
|
|
|
|
|
|
|
|
|
|
|
|
|
|
|
|
|
|
|
|
|
|
|
|
1 |
|
|
|
|
|
|
|
|
|
|
|
|
|
|
|
||
|
|
|
|
|
|
|
|
|
|
|
|
|
|
|
|
|
|
|
|
|
|
|
|
|
|
||||
|
|
|
|
|
|
|
|
|
|
|
|
|||
|
100 |
|
|
|
|
Screen-film |
|
|||||||
|
|
|
|
|
|
|
|
|
|
|
|
|
|
|
|
|
|
|
|
|
|
|
|
|
|
|
|
|
|
|
|
|
10–2 |
10–1 100 |
101 |
102 |
|
|
|
|||||
|
|
|
|
|
Exposure (mR) |
|
|
|
|
|
Figure 7. Comparison of dynamic range of CR and screen-film image receptors. (Courtesy of Fuzifilm Medical Systems, USA, Inc.) The image plate (IP) has wider linear response to radiation extends over four decades of dose and eliminates the inherent limitations of the ‘‘toe’’ and ‘‘shoulder’’ portions of the film’s H&D Curve. (Courtesy of Fujifilm Medical Systems USA, Inc.)
‘‘width’’. The display ‘‘window level’’ corresponds to the film optical density in SFM, and the ‘‘widow width’’ corresponds to the contrast. In digital imaging systems, both window level and width may be adjusted to optimize the image rendered on the monitor. Figure 7 shows the response differences of these two image receptor systems to the radiation exposure. The exposure range for the CRs ‘‘Image Plate’’ is wider than that of a typical screen-film combination by an order of 2 to 3 in magnitude.
THE STEREOTACTIC BREAST BIOPSY MAMMOGRAPHY
Breast biopsy is often required when an area of suspicious malignancy site is revealed after a mammography is obtained. In order to accurately extract the specimen, a stereo mammogram may be obtained so that the biopsy needle can be accurately inserted to the site where it is suspected of malignancy; core biopsy (25). Most dedicated mammography equipment are designed to perform routine (screen-film, or FFDM) mammography, and with an attachment to perform stereotactic mammography and core biopsy in an ‘‘up right’’ posture; either the patient is standing or sitting on a chair.
From a pair of stereo images separated by 308 ( 158 from the centerline), using the triangulation technique, the three-dimensional (3D) coordinates of the suspicious site can be calculated within an accuracy of 1 mm (25), see the schematic diagram of stereotactic imaging geometry in Fig. 8. It is essential that the patient remain still before and after the acquisition of the stereo images. The core biopsy can be localized accurately only if the patient positioning remain the same.
Stereotactic mammography requires that the breast being examined is compressed (just as in routine mammography), but the patient should remain standing still while the mammogram is being processed. The processing time alone takes at least 90 s with typical automatic film processor. Thereafter, the best location of the biopsy needle entrant site, and the coordinates of the sampling must be determined. The prolonged time represents substantial

+/– 15°
Z |
Y |
X |
Compressed breast
Breast holder
Imaging plane/film
Right image Left image Target
Figure 8. Schematic drawing of geometrical setup for stereotactic mammography. From the stereo images, with known geometry and the X–Y coordinates, the depth in Z direction is calculated via triangulation.
discomfort on the part of the patient not to mention the anxiety of fear for the possibility of being diagnosed and confirmed as having breast cancer.
DIGITAL SPOT MAMMOGRAPHY
Before the introduction of FFDM, in order to ease the discomfort of undergoing the biopsy process, a small detector with imaging area of 5 5 cm had been developed by LoRad, for example, so that the stereo images of the breast can be displayed immediately after exposures are made. This is one of the successful biomedical applications that have resulted from collaborative technology transfer programs between the National Aeronautics and Space Administration (NASA), the National Cancer Institute (NCI), and the U. S. Department of Health and Human Services Office on Women’s Health (OWH) (26). Since the imaging area is only 5 5 cm, it is referred to as Digital Spot Mammography. The biopsy coordinate is then calculated via the built-in software program with a shorter overall examination time.
In addition, dedicated breast biopsy systems have been developed where the patient would be lying on her stomach (recumbent). The breast under examination is positioned through a hole in the examination table and aligned with the X-ray equipment and the biopsy needle gun. The recumbent core biopsy mammography unit manufactured by LoRad is depicted in Figs. 9 and 10. LoRad is now one of the brand names sold under Hologic. A similar recumbent core biopsy unit, called Mammo Test Biopsy System, is available from Fisher Imaging.
FULL FIELD DIGITAL MAMMOGRAPHY
The CR-M is a direct replacement of the screen-film cassette. In other words, the IP cassette replaced the screen-
MAMMOGRAPHY 303
Figure 9. The recumbent stereotactic core biopsy system. A recumbent design provides a stable patient positioning to acquire a pair of stereo images for accurate localization of biopsy site while the patient lays flat on her stomach with comfort during the entire examination. (Courtesy of Hologic.)
film cassette. Therefore, no major mammography equipment modification would be necessary. In DR on the other hand, just as in conventional radiography and fluoroscopy applications, the image receptor system may be built into the image receptor compartment as an integral part of the imaging assembly (27,28). Manufacturers had attempted to physically fit the DR detector assembly, or more accurately; the Flat Panel (FP) detector assembly, into the space occupied by the SFM cassette. To date, no commercial product of FP detector assembly has been fitted as a direct physical replacement of the SFM cassette is available (29). In other words, due to technical difficulties, there is no FP detector assembly that is sufficiently compact to fit into the space designed to accommodate the SFM cassette. The image acquired with FP detector is transmitted to and processed by the image processing chain thereafter.
While there are a hand full of FFDM systems either under development or manufactured for clinical applications, three FFDM units are currently available in the United States, and are described here (27). The fact that
Figure 10. The zoomed view of the recumbent stereotactic core biopsy system. In the zoomed view, the breast under the examination is positioned through the opening in the tabletop. (Courtesy of Hologic.)

304 MAMMOGRAPHY
Figure 11. Schematic drawing of an amorphous silicon digital detector. (Courtesy of GE Healthcare.)
these three systems are designed to cover an imaging field of at least ( ) 18 24 cm, the smaller cassette size of SFM, the name; FFDM was assigned. This is to signify the full size imaging filed coverage as opposed to the small field detector of LoRad’s Digital Spot Mammography, or Fischer Imaging’s Mammo Test Biopsy System.
There is a trend that mammography equipment is being transformed to ‘‘digital’’ format. One reason of the slow pace of this transformation is the large initial capital expenditure of FFDM. Both, analog and digital formats are expected to coexist for many more years to come. The impact of Fuji’s CR-M cannot be ignored due to the fact that it is possible to convert and replace the screen-film cassette directly. Any of the existing SFM units will be able to join the ‘‘digital era’’.
Currently, there are three FFDM systems that have received FDA approval and are sold in the United States. The GE Senogaphe 2000D was approved by FDA on January 28, 2000 and ‘‘accredited’’ by ACR in February, 2003. Fischer Senoscan received its FDA approval on September 25, 2001 with subsequent ACR accreditation in August, 2003. Hologic/LoRad’s Selenia received FDA approval and ACR accreditation, respectively, on October 2, 2002, and in September of 2003 (27).
GEs FFDM: SENOGRAPHE 2000D, AND SENOGRAPHE DS
Figure 11 depicts the FP detector developed by General Electric Medical Systems, and the photo inset (top left) shows the Cesium–Iodide (CsI) scintillator crystals. The incident X rays are absorbed by the CsI crystals, which in turn, convert the X-ray photon energy to light. The CsI crystals are deposited in columnar shape so as to minimize the scatter, and optical diffusion of scintillation lights from one column to the other. The underlying photodiode– transistor amorphous Silicon (a-Si) arrays is connected to control data lines, then, converts the light to electrical signals for further processing (30).
GEs FP detector is an indirect-conversion digital detector system. The detective quantum efficiency (DQE) of this FP detector system has been shown to be 60% at Zero Frequency (31,32). Essentially, being the first FFDM system introduced to the commercial market, GEs Senographe
2000D ushered in the digital mammography era to the radiology community. Depicted in Fig. 12 is the secondgeneration FFDM unit, Senographe DS, with the X-ray gantry set to þ 158 with the stereotactic biopsy needle assembly attached. Both Senographe systems (2000D and DS) are equipped with CsI scintillator on an a-Si photodiode–transistor arrays with pixel size of 100 mm and an image matrix size of 1920 2304, covering a field of view 19 23 cm.
FISCHER IMAGING’S SENOSCAN FFDM
On the other hand, Fischer Imaging Corporation’s answer to the FFDM is the Senoscan FFDM unit. The unique feature of Senoscan unit is that it employs a slot mechanism for the X-ray filed collimation that is synchronized to the detector as the slot and detector assembly sweeps across the imaging field, see Fig. 13. The detector of
Figure 12. Photograph of GE FFDM unit; Senographe DS with stereotactic attachment installed. The gantry is tilted to þ158 and prepped for Stereotactic imaging. Note, this is the FFDM version of similar unit shown in Fig. 1, but with zoomed up view for the stereotactic attachment. (Courtesy of GE Healthcare.)

MAMMOGRAPHY 305
|
|
|
|
|
|
|
|
X-ray tube |
|
|
|
|
|
|
|
|
|
Swing arm pivot point |
||||||||||||||||||||||
|
|
|
|
|
|
|
|
|
|
|
|
|
|
|
|
|
|
|
|
|
|
|
|
|
|
|
|
|
|
|
|
|
|
|
|
|
|
|
|
|
|
|
|
|
|
|
|
|
|
|
|
|
|
|
|
|
|
|
|
|
|
|
|
|
|
|
|
|
|
|
|
|
|
|
|
|
|
|
|
|
|
Filter/collimator
assembly
|
|
|
|
|
|
|
|
|
|
|
|
|
|
|
|
|
|
|
|
|
|
|
|
|
|
|
.88 in |
|
84 in |
|
|||||
|
14.5 cm |
|
|
Detector |
10.5 cm |
|
||
|
|
|||||||
|
2977cmi |
|
|
|
|
|||
|
|
|
|
|
121.69cmin
Figure 13. A schematic drawing of the scanning mechanism of Fischer Senoscan FFDM. (Adapted from Fisher Imaging Sanoscan user’s manual with permission. Courtesy of Fischer Imaging.)
Senoscan unit consists of a layer of thallium activated CsI scintillator connected to charge-coupled-device (CCD) chips via fiber optics (33). Hence, this is also an indirectconversion digital detector system.
The use of slot mechanism also means ‘‘restricting’’ the X-ray beams for exposure. It offers an advantage of less scattered radiation for improved image contrast (34). Therefore, no antiscatter grid is necessary with Senoscan unit. The absence of the antiscatter grid compensate for a less efficient use of the available X rays. In addition, a tungsten anode X-ray tube (35) is employed with this unit, since the tungsten anode X-ray tube would produce X rays more efficiently than a molybdenum or rhodium target. Senoscan is equipped with CCD chips having pixel size of
(1) 27 mm, covering a field of view 11 15 cm under the high resolution mode, and (2) pixel size of 54 mm, covering a field of view 19 29 cm for the normal mode. The image matrix size of this system is 4096 5625.
HOLOGIC/LORAD’S FFDM; SELENIA
Selenia’s image receptor is a 250 mm thick amorphous Selenium (a-Se) photoconductor. Underneath a layer of a-Se photoconductor is a thin-film transistor (TFT) arrays that serves as an active readout mechanism. The TFT arrays are typically deposited onto a glass substrate, which provides a physical support for the entire detector components. Selenia uses a 250 mm thick a-Se photoconductor to capture the X-ray photons impinging on the detector sur-
face without the aid of a scintillator. It is said that a thickness of 250 mm a-Se photoconductor is adequate to stop 95% of the X-ray photons in the mammographic energy range (36). The photon energy is converted to a pair of electron, which is negatively charged, and a positively charged ‘‘hole’’. With bias voltage applied, the signal is read off by the TFT arrays. Thus, this is a directconversion digital detector system. The detector is an a-Se photoconductor and TFT arrays with pixel size of 70 mm, covering a field of view 24 29 cm, resulting in an image matrix size of 3328 4096. A summary of the detector characteristics for these three FFDM systems is given in Table 2, (37).
READING MAMMOGRAPHY IMAGES
Initially, the FFDM images were printed on dry laser printers and read on high luminance view boxes (ACR accreditation required of a luminance of > 3000 cd m 2) (38). All three FFDM systems are equipped with workstations where images are soft-copy read. The workstations are commonly equipped with two 5-megapixel high resolution monitors. With the soft-copy reading, an assortment of image manipulation are possible including, but not limited to, basic window width and window level adjustments, zooming, image reversal, and so on for viewing the details of the pathology. More importantly, the impact of image processing in transforming the acquired raw data set to the image displayed for soft-copy reading should be recognized (39). For example, as can be seen in Fig. 14, substantial differences not only in the brightness and contrast of the image but also in the impression of pathology in the images can be noticed. While all three images are acceptable and diagnostic, the two enhanced images are easier to recognize various details.
SUMMARY
A brief history of mammography was described as the introduction to the X-ray mammography. The SFM continues to play its major role in breast cancer detection while FFDM is gaining its installed base. Upon the anticipated FDA approval, the CR-M is poised for introduction for clinical applications in the second-half of 2005. However, all three modalities employed in breast cancer detection, namely; the SFM, FFDM, and the CR-M are expected to
Table 2. Summary of Imaging Characteristics of FFDM Units
Manufacturer |
Model Name |
Scintillator |
Detector |
Pixel Size, mm Image Matrix Size |
Imaging Size, L W cm |
||||||
GE |
Senographe 2000D, DS |
CsI (Tl)a |
|
TFT |
100 |
1920 |
2304 |
19 |
|
23 |
|
Fisher Imaging |
Senoscan |
(Tl) |
|
CCD |
24/48 |
4096 |
|
5625 |
22 |
|
30 |
Hologic/LoRad |
Selenia |
CsI b |
|
TFT |
70 |
3328 |
|
25 |
29 |
||
a-Se |
|
4096 |
|
||||||||
Fuji |
DSM |
CsI (Tl) |
c |
CCD |
48 |
1025 |
1024 |
5 |
|
5 |
|
CR-M |
BaFBr(Eu) |
|
Computed |
50 |
1770 |
2370 |
18 |
|
24 |
||
|
|
|
|
radiography |
|
2364 |
2964 |
24 |
|
30 |
aCsI(Tl) ¼ Talium Activated Cesium Iodide. ba-Se ¼ Amorphous Selenium.
cBaFBr(Eu) ¼ Barium Fluorobromide with Europium.

306 MAMMOGRAPHY
Figure 14. Comparison of ‘‘processed’’ images. The unprocessed image (a) can be dramatically improve its appearance with ‘‘Thickness Equalzation’’ processing (b), or the ‘‘Premium View’’ processing (c). The impact of image processing is quite evident. (Courtesy of GE Healthcare.)
play their roles in contributing to reduction of breast cancer deaths through the early detection of mammography screening.
Display of FFDM images and its workstations are important components of the total picture of FFDM (40,41). However, monitors and workstations associated with diagnostic radiology imaging including mammography are in a domain of their own and no attempt is made to include these subjects in this article. Such subject matters belong to digital image processing and display. Finally, archiving of the acquired digital images is also a very important aspect of digitized diagnostic images in the overall operation of radiology. However, this subject is better handled in Picture Archiving and Communication Systems (PACS), and readers are refered to articles under PACS for additional information.
ACKNOWLEDGMENT
The information and materials presented here had been provided by numerous numbers of representatives and scientists from respective manufacturers mentioned in the main text. The author would like to express his thanks and sincere appreciation by listing the manufacturers here, (in alphabetical order) in lieu of listing the individuals who had provided their supports: General Electric Healthcare, Fischer Imaging Corporation, Fujifilm Medical Systems USA, Inc., and Hologic Inc. (LoRad).
BIBLIOGRAPHY
1.Nystrom L, et al. Breast cancer screening with mammography: Overview of Swedish randomised trial, Lancet 1993; 341:973–978.
2.Hendrick RE, et al. Benefit of screening mammography in women aged 40–49: A new meta-analysis of randomized controlled trial. J Nat Cancer Inst Monograph 1997; (22):87–92.
3.Mammography–-A User’s Guide, Washington, DC: National Council on Radiation Protection and Measurements, 1986. NCRP Report No. 85.
4.Zermenhof RA. Mammography, Encyclopedia of Medical Devices and Instrumentation, Vol 3. New York: John Wiley
&Sons; 1988.
5.Jones CH. Methods of breast imaging. Phys Med Biol 1982;27(4)463–499.
6.FDA Home page. [Online]. Available at http://www.fda.gov/ cdrh/mammography/frmamcom2.html.
7.ACR Home page. [Online]. Available at http://www.acr.org/ s_acr/sec.asp?CID¼589&DID¼14253.
8.Speiser RC, Zanrosso EM, Jeromin LS. Dose comparisons for mammographic systems. Med Phys 1986;13(5):667– 673.
9.Boag JW. Xeroradiography. Phys Med Biol 1973;18:3.
10.Wolbarst AB. Dependence of attenuation on atomic number and photon energy. Physics of Radiology. Appleton & Lange; 1992. Chapt. 14.
11.Hoeffken W. Soft tissues–-mammography. The invisible light applied, the advancing diagnostic evaluation of the skeleton and thorax following Roentgen’s discovery. In: Rosenbusch G, Oudekerk M, Ammann E, editors. Radiology in Medical Diagnostics, Evolution of X-ray Applications 1895–1995. Oxford: Blackwell Science Ltd.; 1995. Chapt. 2.
12.Curry III TS, Dowdey JE, Murry Jr. RC. Christensen’s Physics of Diagnostic Radiology, 4th ed., Philadelphia: Lea
&Febiger; 1990.
13.Fewell TR, Shuping RE. Handbook of Mammographic X-ray Spectra. Rockville, MD: HEW Publication (FDA) 79–8071; 1978.
14.Boone JM, Fewell TR, Jennings RJ. Molybdenum, rhodium, and tungsten anode spectral modeling using interpolating polynomials with application to mammography. Med Phys Dec. 1997:24(12).
15.Sprawls Jr. P. Chapter 18: Blur, Resolution, and Visibility of Detail. Physical Principles of Medical Imaging. Aspen Publishers, Inc.; 1987.
16.Haus AG. Technologic improvements in screen-film mammography. Radiology 1990;174:628–637.
17.Chapter V. Film Response. The Fundamentals of Radiography, 4th ed., Rochester, N.Y.: Eastman Kodak Co.; 1980.
18.Rezentes PS, de Almeida A, Barnes GT. Mammography grid performance. Radiology 1999;210:227.
19.Sonoda M, Takano M, Miyahara J, Kato H. Computed radiography utilizing laser stimulated luminescence. Radiology 1983;148:833–838.
20.Pisano ED, et al. American College of Radiology Imaging Network Digital Mammographic Imaging Screening Trial: Objectives and Methodology. Radiology published online. June 16, 2005.
21.ACRIN Home Page. [Online]. Available at http://www.acrin. org.
22.Communication with National Program Manager, Women’s Healthcare Imaging Systems, Fuji Photo Film Ltd.
23.FCR (Fuji Computed Radiography) General Description of Image Processing, Fuji Photo Film Co., Ltd.
24.Tateno Y, Iinuma T, Takano M, editors. Computed Radiography. Tokyo: Springer-Verlag; 1987.
25.Carr JJ, et al. Stereotactic localization of breast lesions: How it works and methods to improve accuracy. RadioGraphics 2001;21:463.
26.Winfield DL. Aerospace technology transfer to breast cancer imaging. Acta Astronau 1997;41(4–10):515–523.
27.Pisano ED, Yaffe MJ. Digital Mammography. Radiology 2005;234:353–362.
28.Pisano ED. Current status of full-field digital mammography. Radiology 2000;214:26.
29.Personal communications with various X-ray industry marketing departments.