
Cycloaddition Reactions in Organic Synthesis
.pdf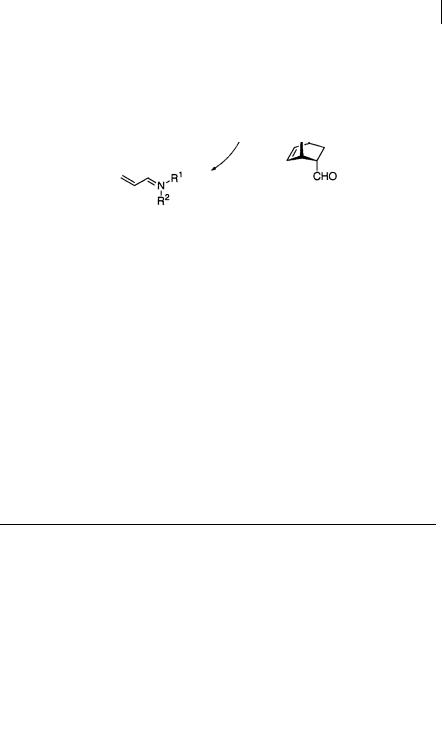
1.3 The Asymmetric Catalytic Diels-Alder Reaction Catalyzed by Base 47
fords the cycloaddition product and regenerates the chiral amine catalyst. Of the several amines examined, 40 was found to be optimum. This Diels-Alder reaction has wide generality in respect of both diene and dienophile (Scheme 1.74, 1.75, Table 1.30, 1.31). Acrolein, crotonaldehyde, and cinnamaldehyde react with cyclopentadiene to give addition products in high optical purity. Cyclohexadiene, isoprene, and 1-methoxybutadiene can all be successfully employed as the diene component.
Scheme 1.71
Scheme 1.72
Table 1.30 Asymmetric Diels-Alder reactions of cyclopentadiene catalyzed by 40 [59]
R |
Time (h) |
Yield (%) |
endo/exo |
endo ee (%) |
exo ee (%) |
|
|
|
|
|
|
Me |
16 |
75 |
1 : 1 |
90 |
86 |
Pr |
14 |
92 |
1 : 1 |
90 |
86 |
Ph |
21 |
99 |
11.3 |
93 |
93 |
|
|
|
|
|
|
Scheme 1.73

48 1 Catalytic Asymmetric Diels-Alder Reactions
Table 1.31 Asymmetric Diels-Alder reactions catalyzed by 40 [59]
R1 |
R2 |
R3 |
Yield (%) |
endo/exo |
ee (%) |
|
|
|
|
|
|
|
|
Me |
H |
H |
84 |
– |
|
89 |
Ph |
H |
H |
90 |
– |
|
83 |
Ph |
H |
Me |
75 |
– |
|
90 |
Me |
Me |
H |
75 |
5 |
: 1 |
90 |
H |
OAc |
H |
72 |
11 |
: 1 |
85 |
|
|
|
|
|
|
|
1.4
Conclusions
Many chiral metal complexes with Lewis acid properties have been developed and applied to the asymmetric Diels-Alder reaction. High enantioselectivity is, of course, one of the goals in the development of these catalysts. Enantioselectivity is not, however, the only factor important in their design. Other important considerations are:
(i)the generality of the reaction, with regard to the combinations of diene and dienophile that can be used;
(ii)chemical yield;
(iii)diastereomer selectivity (endo/exo);
(iv)ease of preparation of the ligand and catalyst;
(v)catalyst stability;
(vi)catalyst recovery; and
(vii)reaction conditions (reaction temperature and time).
Among the many chiral Lewis acid catalysts described so far, not many practical catalysts meet these criteria. For a, -unsaturated aldehydes, Corey’s tryptophan-derived borane catalyst 4, and Yamamoto’s CBA and BLA catalysts 3, 7, and 8 are excellent. Narasaka’s chiral titanium catalyst 31 and Evans’s chiral copper catalyst 24 are outstanding chiral Lewis acid catalysts of the reaction of 3-alkenoyl-1,2-oxazolidin-2-one as dienophile. These chiral Lewis acid catalysts have wide scope and generality compared with the others, as shown in their application to natural product syntheses. They are, however, still not perfect catalysts. We need to continue the endeavor to seek better catalysts which are more reactive, more selective, and have wider applicability.
1.5
Appendix
Below is a table of asymmetric Diels-Alder reactions of a, -unsaturated aldehydes catalyzed by chiral Lewis acids 1–17 (Fig. 1.10, 1.11). The amount of catalyst, reaction conditions (temperature, time), chemical yield, endo/exo selectivity, and optical purity are listed (Table 1.32).

Table 1.32 Asymmetric Diels-Alder reactions of -unsaturated aldehydes
Catalyst
1 [1] |
|
0.15 equiv. |
|
27% ee |
|
|
|
–78 C |
|
|
|
|
|
quant, 2 : 98 |
|
|
|
|
|
72% ee |
|
|
|
2 [4] |
|
0.005 equiv. |
|
|
|
|
|
–78 C, 4 h |
|
|
|
|
|
100%, 3 : 97 |
|
|
|
|
|
97.7% ee |
|
|
|
3 [5] |
0.1 equiv. |
0.1 equiv. |
0.1 equiv. |
0.1 equiv. |
0.1 equiv. |
|
–78 C, 10 h |
–78 C, 6 h |
–40 C, 12 h |
–78 C, 14.5 h |
–78 C, 10 h |
|
quant, 6 : 94 |
85%, 11 : 89 |
52% |
90%, 88 : 12 |
53%, 90 : 10 |
|
98% ee |
96% ee |
87% ee |
84% ee |
2% ee |
4 [6] |
0.05 equiv. |
92% ee |
0.05 equiv. |
40% ee |
|
|
–78 C, 1 h |
|
–40 C, 48 h |
|
|
|
95%, 4 : 96 |
|
76% |
|
|
|
99% ee |
|
92% ee |
|
|
5 [7] |
|
0.15 equiv. |
|
|
|
|
|
–78 C |
|
|
|
|
|
88%, 4 : 96 |
|
|
|
|
|
95% ee |
|
|
|
6 [8] |
|
0.2 equiv. |
|
|
|
|
|
–78 C, 12 h |
|
|
|
84%, >1:99 97% ee
Appendix 5.1
49

Table 1.32 Continued
Catalyst
7 |
[10] |
0.05 equiv. |
0.1 equiv. |
0.1 equiv. |
|
0.1 equiv. |
0.1 equiv. |
R = H |
|
|
–78 C, 4 h |
–78 C |
–40 C |
|
–78 C |
–78 C |
0.2 equiv. |
|
|
>99%, >1 : 99 |
>99%, >1 : 99 |
>99% |
|
91%, 91 : 9 |
12%, 89 : 11 |
–78 C |
|
|
>99% ee |
99% ee |
98% ee |
|
40% ee |
36% ee |
63% |
|
|
|
|
|
|
|
|
88% ee |
8 |
[10] |
0.05 equiv. |
|
0.1 equiv. |
0.1 equiv. |
0.05 equiv. |
0.2 equiv. |
|
|
|
–78 C |
|
–78 C |
–78 C |
–78 C |
–78 C |
|
|
|
>99%, 10 : 90 |
|
95% |
65%, 90 : 10 |
84%, 97 : 3 |
94%, 90 : 10 |
|
|
|
>99% ee |
|
>99% ee |
95% ee |
95% ee |
95% ee |
|
9 |
[11, 12]0.1 equiv. |
0.1 equiv. |
0.1 equiv. |
0.1 equiv. |
|
|
R = TMS |
|
|
|
–94 C, 2 h |
–94 C, 2 h |
–94 C, 1 h |
–94 C, 2 h |
|
|
0.2 equiv. |
|
|
99%, 9 : 91 |
99%, 12 : 88 |
99% |
99%, 96 : 4 |
|
|
–94–78 C |
|
|
98% ee |
90% ee |
96% ee |
93% ee |
|
|
68% |
|
|
|
|
|
|
|
|
87% ee |
10 |
[16] |
|
0.1 equiv. |
|
|
0.1 equiv. |
0.1 equiv. |
|
|
|
|
–78 C, 70 h |
|
|
–78 C, 3.5 h |
–40 C, 50 h |
|
|
|
|
75%, 1 : 99 |
|
|
70%, 85 : 15 |
76%, 70 : 30 |
|
|
|
|
94% ee |
|
|
96% ee |
95% ee |
|
11 |
[17] |
0.1 equiv. |
|
0.1 equiv. |
|
|
|
|
|
|
–78 C, 0.5 h |
|
–78 C, 48 h |
|
|
|
|
|
|
94%, 1 : 67 |
|
quant |
|
|
|
|
|
|
93% ee |
|
90% ee |
|
|
|
|
|
|
|
|
|
|
|
|
|
Reactions Alder-Diels Asymmetric Catalytic 1 50

Table 1.32 Continued
Catalyst
12 [18] |
|
|
0.05 equiv. |
|
|
|
|
–30 C, |
|
|
|
|
1–3 h |
|
|
|
|
95% |
|
|
|
|
95% ee |
|
13 [19] |
0.05 equiv. |
0.05 equiv. |
0.05 equiv. |
0.05 equiv. |
|
–20 C, 2 h |
–20 C, 20 h |
–20 C, 20 h |
–30 C, 16 h |
|
83%, 6 : 94 |
83%, 2 : 98 |
86%, 90 : 10 |
76%, 66 : 34 |
|
95% ee |
97% ee |
>99% ee |
81% ee |
14 [20] |
0.05 equiv. |
0.05 equiv. |
0.05 equiv. |
|
|
–20 C, 2 h |
–20 C, 22 h |
–20 C, 21 h |
|
|
93%, 7 : 93 |
91%, 3 : 97 |
29%, 90 : 10 |
|
|
92% ee |
92% ee |
95% ee |
|
16 [22] |
0.1 equiv. |
|
|
|
|
–40 C, 48 h |
|
|
|
|
93%, 3 : 97 |
|
|
|
|
86% ee |
|
|
|
17 [23] |
0.05 equiv. |
0.05 equiv. |
|
0.05 equiv. |
|
–78 C, 12 h |
–40 C, 8 h |
|
–20 C, 18 h |
|
>95% conv., |
>95% conv., |
|
96 : 4 |
|
2 : 98 |
3 : 97 |
|
85% ee |
|
96% ee |
92% ee |
|
|
For each reaction the data given are:
Amount of catalyst;
Temperature, time;
Yield, endo/exo; and
Optical yield.
51 Appendix 5.1
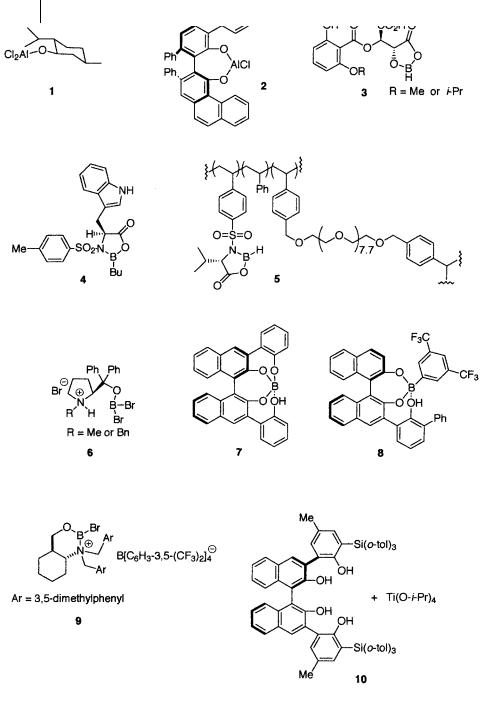
52 1 Catalytic Asymmetric Diels-Alder Reactions
Fig. 1.10
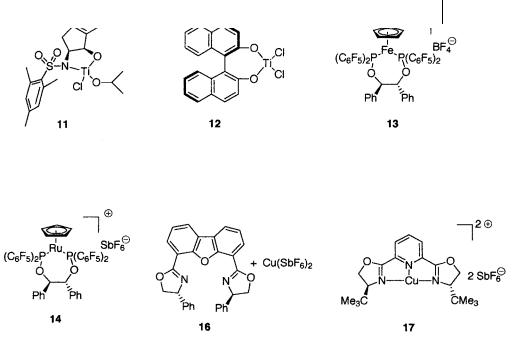
References 53
Fig. 1.11
Acknowledgment
I would like to thank Professors E. J. Corey and K. Narasaka for giving me a chance to work with super-reactive chiral catalyst 9 and TADDOL-based chiral titanium catalyst 31, respectively.
References
[1] |
(a) S. Hashimoto, N. Komeshima, K. |
|
lysts, in: Catalytic Asymmetric Synthesis, I. |
|
Koga, J. Chem. Soc., Chem. Commun. |
|
Ojima (Ed.), VCH, New York, 1993, |
|
1979, 437; (b) H. Takemura, N. Kome- |
|
p 413; (e) R. Noyori, Asymmetric Cataly- |
|
shima, I. Takahashi, S. Hashimoto, N. |
|
sis, in: Organic Synthesis, John Wiley and |
|
Ikota, K. Tomioka, K. Koga, Tetrahedron |
|
Sons, New York, 1994, p 212. |
|
Lett. 1987, 28, 5687. |
[3] |
(a) E. J. Corey, R. Imwinkelried, S. Pi- |
[2] |
(a) D. A. Evans, J. S. Johnson, Diels-Al- |
|
kul, Y. B. Xiang, J. Am. Chem. Soc. 1989, |
|
der Reaction, in: Comprehensive Asym- |
|
111, 5493; (b) E. J. Corey, S. Sarshar, J. |
|
metric Catalysis, Vol. III, E. N. Jacobsen, |
|
Border, J. Am. Chem. Soc. 1992, 114, |
|
A. Pfaltz, H. Yamamoto (Eds), Springer, |
|
7938. |
|
New York, 1999, p 1177; (b) H. B. Kagan, |
[4] |
(a) J. Bao, W. D. Wulff, A. L. Rhein- |
|
O. Riant, Chem. Rev. 1992, 92, 1007; (c) |
|
gold, J. Am. Chem. Soc. 1993, 115, 3814; |
|
K. Narasaka, Synthesis 1991, 1; (d) K. |
|
(b) J. Bao, W. D. Wulff, J. B. Dominy, |
|
Maruoka, H. Yamamoto, Asymmetric |
|
M. J. Fumo, E. B. Grant, A. C. Rob, M. C. |
|
Reactions with Chiral Lewis Acid Cata- |
|
Whitcomb, S.-M. Yeung, R. L. Ostran- |

54 1 Catalytic Asymmetric Diels-Alder Reactions
der, A. L. Rheingold, J. Am. Chem. Soc.
1996, 118, 3392.
[5](a) K. Furuta, S. Shimizu, Y. Miwa, H. Yamamoto, J. Org. Chem. 1989, 54, 1481;
(b) K. Ishihara, Q. Gao, H. Yamamoto,
J.Org. Chem. 1993, 58, 6917; (c) K. Ishihara, Q. Gao, H. Yamamoto, J. Am. Chem. Soc. 1993, 115, 10412; (d) K. Furuta, A. Kanematsu, H. Yamamoto, S. Takaoka, Tetrahedron Lett. 1989, 30, 7231.
[6](a) E. J. Corey, T-P. Loh, J. Am. Chem. Soc. 1991, 113, 8966; (b) E. J. Corey, T.-P. Loh, T. D. Roper, M. D. Azimioara,
M.C. Noe, J. Am. Chem. Soc. 1992, 114, 8290; (c) E. J. Corey, A. Guzman-Perez, T.-P. Loh, J. Am. Chem. Soc. 1994, 116, 3611; (d) E. J. Corey, T.-P. Loh, Tetrahedron Lett. 1993, 34, 3979.
[7]K. Kamahori, K. Ito, S. Itsuno, J. Org. Chem. 1996, 61, 8321.
[8]S. Kobayashi, M. Murakami, T. Harada, T. Mukaiyama, Chem. Lett. 1991, 1341.
[9]V. K. Aggarwal, E. Anderson, R. Giles,
A.Zaparucha, Tetrahedron: Asymmetry
1995, 6, 1301.
[10](a) K. Ishihara, H. Yamamoto, J. Am. Chem. Soc. 1994, 116, 1561; (b) K. Ishihara, H. Kurihara, H. Yamamoto, J. Am. Chem. Soc. 1996, 118, 3049; (c) K. Ishihara, S. Kondo, H. Kurihara, H. Yamamoto, S. Ohashi, S. Inagaki, J. Org. Chem. 1997, 62, 3026; (d) K. Ishihara, H. Kurihara, M. Matsumoto, H. Yamamoto, J. Am. Chem. Soc. 1998, 120, 6920.
[11]Y. Hayashi, J. J. Rohde, E. J. Corey, J. Am. Chem. Soc. 1996, 118, 5502.
[12]E. J. Corey, T. W. Lee, Tetrahedron Lett.
1997, 38, 5755.
[13](a) E. J. Corey, J. J. Rohde, A. Fischer,
M.D. Azimioara, Tetrahedron Lett. 1997,
38, 33; (b) E. J. Corey, J. J. Rohde, Tetrahedron Lett. 1997, 38, 37; (c) E. J. Corey,
D.Barnes-Seeman, T. W. Lee, Tetrahedron Lett. 1997, 38, 1699.
[14]M. T. Reetz, M. Hüllmann, W. Massa, S. Berger, P. Rademacher, P. Heymanns,
J.Am. Chem. Soc. 1986, 108, 2405.
[15]E. J. Corey, T.-P. Loh, S. Sarshar, M. Azimioara, Tetrahedron Lett. 1992, 33, 6945.
[16]K. Maruoka, N. Murase, H. Yamamoto,
J.Org. Chem. 1993, 58, 2938.
[17]E. J. Corey, T. D. Roper, K. Ishihara, G. Sarakinos, Tetrahedron Lett. 1993, 34, 8399.
[18]Y. Motoyama, M. Terada, K. Mikami,
Synlett 1995, 967.
[19](a) E. P. Kündig, B. Bourdin, G. Bernardinelli, Angew. Chem., Int. Ed. Engl.
1994, 33, 1856; (b) M. E. Bruin, E. P. Kündig, Chem. Commun. 1998, 2635.
[20]E. P. Kündig, C. M. Saudan, G. Bernardinelli, Angew. Chem., Int. Ed. Engl.
1999, 38, 1220.
[21]Y. Huang, T. Iwama, V. H. Rawal, J. Am. Chem. Soc. 2000, 122, 7843.
[22]S. Kanemasa, Y. Oderaotoshi, S. Sakaguchi, H. Yamamoto, J. Tanaka, E. Wada, D. P. Curran, J. Am. Chem. Soc.
1998, 120, 3074.
[23]D. A. Evans, D. M. Barnes, J. S. Johnson, T. Lectka, P. von Matt, S. J. Miller, J. A. Murry, R. D. Norcross, E. A. Shaughnessy, K. R. Campos, J. Am. Chem. Soc. 1999, 121, 7582.
[24](a) J. M. Hawkins, S. Loren, J. Am. Chem. Soc. 1991, 113, 7794; (b) J. M. Hawkins, S. Loren, M. Nambu, J. Am. Chem. Soc. 1994, 116, 1657.
[25]D. P. Heller, D. R. Goldberg, W. D. Wulff, J. Am. Chem. Soc. 1997, 119, 10551.
[26](a) D. A. Evans, K. T. Chapman, J. Bisaha, J. Am. Chem. Soc. 1988, 110, 1238;
(b) D. A. Evans, K. T. Chapman, D. T. Hung, A. T. Kawaguchi, Angew. Chem., Int. Ed. Engl. 1987, 26, 1184; (c) D. A. Evans, K. T. Chapman, J. Bisaha, Tetrahedron Lett. 1984, 25, 4071; (d) D. A. Evans, K. T. Chapman, J. Bisaha, J. Am. Chem. Soc. 1984, 106, 4261.
[27]K. Narasaka, M. Inoue, N. Okada,
Chem. Lett. 1986, 1109.
[28]E. J. Corey, R. Imwinkelried, S. Pikul,
Y.B. Xiang, J. Am. Chem. Soc. 1989, 111, 5493.
[29]E. J. Corey, S. Sarshar, J. Bordner, J. Am. Chem. Soc. 1992, 114, 7938.
[30]E. J. Corey, K. Ishihara, Tetrahedron Lett.
1992, 33, 6807.
[31](a) G. Desimoni, G. Faita, P. P. Righetti, Tetrahedron Lett. 1996, 37, 3027; (b) P. Carbone, G. Desimoni, G. Faita, S. Fi-

lippone, P. P. Righetti, Tetrahedron
1998, 54, 6099.
[32]T. Ichiyanagi, M. Shimizu, T. Fujisawa,
J.Org. Chem. 1997, 62, 7937.
[33](a) D. A. Evans, S. J. Miller, T. Lectka,
P.von Matt, J. Am. Chem. Soc. 1999,
121, 7559; (b) D. A. Evans, S. J. Miller,
T.Lectka, J. Am. Chem. Soc. 1993, 115, 6460; (c) D. A. Evans, J. A. Murry, P. von Matt, R. D. Norcross, S. J. Miller,
Angew. Chem., Int. Ed. Engl. 1995, 34,
798; (d) D. A. Evans, J. S. Johnson, J. Org. Chem. 1997, 62, 786; (e) D. A. Evans, E. A. Shaughnessy, D. M. Barnes, Tetrahedron Lett. 1997, 38, 3193;
(f) D. A. Evans, D. M. Barnes, Tetrahedron Lett. 1997, 38, 57; (g) J. S. Johnson,
D.A. Evans, Acc. Chem. Res. 2000, 33, 325.
[34]D. A. Evans, T. Lectka, S. J. Miller, Tetrahedron Lett. 1993, 34, 7027.
[35](a) I. W. Davies, L. Gerena, L. Castonguay, C. H. Senanayake, R. D. Larsen,
T.R. Verhoeven, P. J. Reider, J. Chem. Soc., Chem. Commun. 1996, 1753; (b)
I.W. Davies, R. J. Deeth, R. D. Larsen,
P.J. Reider, Tetrahedron Lett. 1999, 40, 1233.
[36]A. K. Ghosh, P. Mathivanan, J. Cappiello, Tetrahedron Lett. 1996, 37, 3815.
[37]I. Sagasser, G. Helmchen, Tetrahedron Lett. 1998, 39, 261.
[38]J. M. Brunel, B. D. Campo, G. Buono,
Tetrahedron Lett. 1998, 39, 9663.
[39]E. J. Corey, N. Imai, H.-Y. Zhang, J. Am. Chem. Soc. 1991, 113, 728.
[40](a) K. Narasaka, M. Inoue, T. Yamada, Chem. Lett. 1986, 1967; (b) K. Narasaka,
N.Iwasawa, M. Inoue, T. Yamada, M. Nakashima, J. Sugimori, J. Am. Chem. Soc. 1989, 111, 5340.
[41]Seebach et al. also investigated the Ti- TADDOlate-catalyzed Diels-Alder reaction, see D. Seebach, R. Dahinden,
R.E. Marti, A. K. Beck, D. A. Plattner,
F.N. M. Kühnle, J. Org. Chem. 1995, 60, 1788.
References 55
[42]K. Narasaka, H. Tanaka, F. Kanai, Bull. Chem. Soc. Jpn. 1991, 64, 387.
[43]K. Narasaka, I. Yamamoto, Tetrahedron
1992, 48, 5743.
[44]I. Yamamoto, K. Narasaka, Bull. Chem. Soc. Jpn 1994, 67, 3327.
[45]N. Iwasawa, J. Sugimori, Y. Kawase, K. Narasaka, Chem. Lett. 1989, 1947.
[46]K. Narasaka, M. Saitou, N. Iwasawa,
Tetrahedron: Asymmetry 1991, 2, 1305.
[47]N. Iwasawa, Y. Hayashi, H. Sakurai, K. Narasaka, Chem. Lett. 1989, 1581.
[48]K. V. Gothelf, R. G. Hazell, K. A. Jørgensen, J. Am. Chem. Soc. 1995, 117, 4435.
[49]C. Haase, C. R. Sarko, M. DiMare, J. Org. Chem. 1995, 60, 1777.
[50]J. B. Jaquith, C. J. Levy, G. V. Bondar, S. Wang, S. Collins, Organometallics 1998,
17, 914.
[51](a) S. Kobayashi, I. Hachiya, H. Ishitani, M. Araki, Tetrahedron Lett. 1993, 34, 4535; (b) S. Kobayashi, H. Ishitani, J.
Am. Chem. Soc. 1994, 116, 4083; (c) S. Kobayashi, H. Ishitani, I. Hachiya, M. Araki, Tetrahedron 1994, 50, 11623.
[52]A. Nishida, M. Yamanaka, M. Nakagawa, Tetrahedron Lett. 1999, 40, 1555.
[53]T. Morita, T. Arai, H. Sasai, M. Shibasaki, Tetrahedron: Asymmetry 1998, 9, 1445.
[54]E. J. Corey, S. Sarshar, D.-H. Lee, J. Am. Chem. Soc. 1994, 116, 12089.
[55]E. J. Corey, M. A. Letavic, J. Am. Chem. Soc. 1995, 117, 9616.
[56]E. Wada, W. Pei, S. Kanemasa, Chem. Lett. 1994, 2345.
[57]K. Mikami, Y. Motoyama, M. Terada, J. Am. Chem. Soc. 1994, 116, 2812.
[58](a) I. E. Markó, G. R. Evans, P. Seres, I. Chellé, Z. Janousek, Pure Appl. Chem. 1996, 68, 113; (b) I. E. Markó, G. R. Evans, Tetrahedron Lett. 1994, 35, 2771.
[59]K. A. Ahrendt, C. J. Borths, D. W. C. MacMillan, J. Am. Chem. Soc. 2000,
122, 4243.
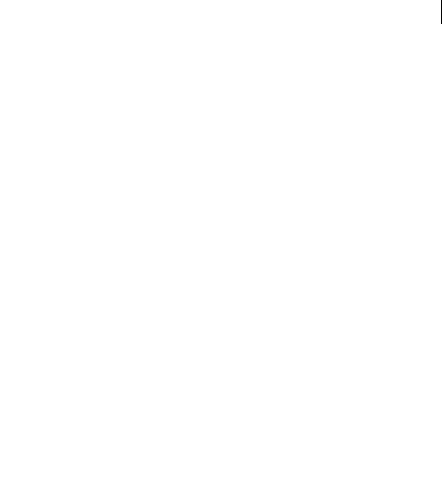
Cycloaddition Reactions in Organic Synthesis. 57
Edited by S. Kobayashi and K. A. Jorgensen
Copyright © 2001 Wiley-VCH Verlag GmbH
ISBNs: 3-527-30159-3 (Hardcover); 3-527-60025-6 (Electronic)
2
Recent Advances in Palladium-catalyzed Cycloadditions involving Trimethylenemethane and its Analogs
Dominic M. T. Chan
2.1
General Introduction
The discovery of palladium trimethylenemethane (TMM) cycloadditions by Trost and Chan over two decades ago constitutes one of the significant advancements in ring-construction methodology [1]. In their seminal work it was shown that in the presence of a palladium(0) catalyst, 2-[(trimethylsilyl)methyl]-2-propen-1-yl acetate (1) generates a TMM-Pd intermediate (2) that serves as the all-carbon 1,3-di- pole. It was further demonstrated that (2) could be efficiently trapped by an elec- tron-deficient olefin to give a methylenecyclopentane via a [3+2] cycloaddition (Eq. 1).
!1"
Since its inception, considerable progress has been made by the laboratories of Trost and others in expanding this TMM cycloaddition chemistry. The advancements include the development of substituted analogs, improved catalytic systems, other TMM precursors, and analogous cycloaddition reactions involving both interand intramolecular systems. In addition to two reviews by Trost [2], different aspects of the area were surveyed by Inoue in 1985, by Chan in 1989, and by Lautens in 1996 [3–5]. This chapter will focus only on the TMM chemistry using Trost’s approach, even though other methodologies are also available [3, 4]. It will provide some brief background information, but will concentrate primarily on recent advances. Both published works in the literature and unpublished results from Trost’s laboratory will be presented. Readers are encouraged to peruse the aforementioned reviews for more comprehensive surveys of the area in its earlier development.