
Multidimensional Chromatography
.pdf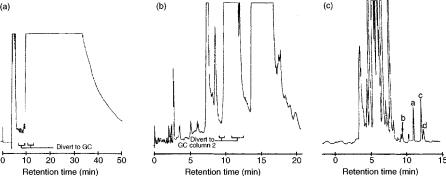
410 |
Multidimensional Chromatography |
Figure 15.2 Three-stage LC – GC – GC separation of stilbenes from corned beef: (a) initial HPLC stage; (b) first GC stage; (c) second GC stage showing separated analytes. Peak identification in (c) is as follows: a, trans-diethylstilbesterol; b, cis-diethylstilberterol; c, hexestrol; d, dienestrol. Adapted from Journal of High Resolution Chromatography, 16, G.C. Chappell et al., ‘On line high performance liquid chromatography – multidimensional gas chromatography and its application to the determination of stilbene hormones in corned beef, pp. 479 – 482, 1993, with permission from Wiley-VCH.
LC–GC, therefore, shows promise for forensic science applications, reducing sample handling and preparation steps by essentially using an on-line LC column in place of one or more extraction steps. This is followed by a traditional high resolution GC analysis. The methods described here for pesticides and hormones could be readily adapted to a variety of analyses, especially those involving fatty matrices, such as tissues, food or blood.
15.3 LIQUID – CHROMATOGRAPHY –LIQUID CHROMATOGRAPHY
Multidimensional liquid chromatography (LC–LC) has been employed mainly in efforts to directly inject biological materials such as urine, blood, plasma or serum, without prior clean-up steps. The particular advantage of direct injection methods actually lies in the disadvantages of classical extraction and preparation methods, which require extensive handling of potentially infectious samples, and losses of material due to these handling steps. Multidimensional chromatography also lends itself well to automation, which may be required in forensic or drug-monitoring laboratories. The analytes of interest in the direct injection of biological fluids include mostly drugs and other compounds of clinical interest. The use of guard columns and other pre-column devices is not described here, as these are generally used only for sample clean-up, rather than for improvement of fundamental chromatographic performance. Most systems for multidimensional LC–LC consist of two or more columns connected by column switching pneumatics, and these are described in detail in Chapter 5 of this text. Several examples of drug analysis from blood and
Forensic and Toxicological Applications |
411 |
plasma are described here, showing the utility of the first HPLC column to remove matrix components.
LC – LC has been widely applied for applications such as the determination of drugs and metabolites from blood. In this case, it is common to use an alkyl-bonded first column, to allow the proteinaceous material to pass through, while retaining the drugs of interest. Fouling of the first column with proteins and other high-molecular- weight interferences is a major problem in this technique. In order to avoid this problem, size-exclusion chromatography (SEC) is also commonly used as the precolumn procedure. Again, the large interferences elute rapidly, while the smaller drug molecules are retained.
Micellar HPLC, developed in the 1970s and 1980s has provided both alternatives to and interesting methods for multidimensional analysis. Micellar systems have been used by many workers for on-line sample clean-up, with an emphasis on the direct injection of biological fluids such as urine or blood. In a typical application, Posluszny and Weinberger (9) used sodium dodecyl sulfate above the critical micelle concentration as a mobile phase modifier. The micelles form a pseudo-stationary phase, which moves along with the solvent flow. This third phase can effectively partition interfering components, thus removing them from the column and avoiding fouling of the stationary phase. In multidimensional applications, the second column can mitigate the well-known efficiency limitations of single-dimension micellar chromatography. When the process is automated by the use of switching valves, automated, on-line enrichment and separation of drugs from complex urine and blood samples can be performed. Posluszny and Weinberger (9) analyzed several drugs from plasma, with direct injection of the plasma onto their multidimensional system. It is worth noting that they employed a ‘consumable’ inexpensive precolumn, which they simply replaced when fouled, typically after several hundred injections. In Figure 15.3, an analysis of tricyclic antidepressants from blood plasma is shown. Filtration was the only sample treatment prior to injection and no carryover between injections was seen. It is seen that excellent efficiency and separation of the drug from the matrix can be achieved in a relatively short (less than 20 min) analysis time. These authors also demonstrated the direct injection and identification of drugs in plasma by using multidimensional high performance liquid chromatography (HPLC) with photodiode array detection (10).
More recently, Carda-Broch et al. described the analysis of cardiovascular drugs from urine by using micellar HPLC (11). These authors present extensive method development information, including resolution maps showing micelle and mobile phase composition. In effect, this may be viewed as a multidimensional technique within a single column. Typical separations of beta-blockers, using three different mobile phase compositions, are shown in Figure 15.4. Although analysis times are somewhat long, excellent resolution can be obtained, and tremendous effects on the selectivity are seen.
Stopher and Gage used size-exclusion chromatography (SEL) coupled to reversed phase HPLC for the direct injection of plasma in the analysis of an antifungal agent, voriconazole (12). Their system consisted of three columns, i.e. first a size-exclusion
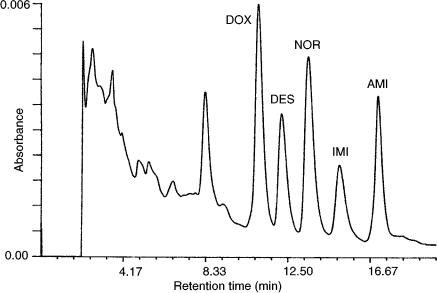
412 |
Multidimensional Chromatography |
Figure 15.3 Separation of tricyclic antidepressants by using multidimensional LC – LC. Peak identification is as follows: DOX, doxepin; DES, desipramine; NOR, nortryptylene; IMI, imipramine; AMI, amitryptyline. Adapted from Journal of Chromatography, 507, J. V. Posluszny et al., ‘Optimization of multidimensional high-performance liquid chromatography for the determination of drugs in plasma by direct injection, micellar cleanup and photodiode array detection’, pp. 267 – 276, copyright 1990, with permission from Elsevier Science.
column, primarily used for de-salting followed by a pre-column, followed by a C18 analytical column. The pre-column was used for a final clean-up, plus re-focusing of the analytes prior to separation on the analytical column. This system allowed the direct injection of approximately 0.5 mL of plasma. The limit of quantitation of the method was found to be 5 g/mL, with quantitation by internal standard. Figure 15.5 shows an analysis of voriconazole with an internal standard. Resolution between these very similar compounds is excellent, with a relatively short analysis time and little matrix interference.
Another system based on an SEC pre-column was employed by Earley and Tini for the analysis of a nonpeptidic inhibitor of human leukocyte elastase as part of a drug discovery project (13). The analytical column used here was octadecylsilane, although the authors commented that, depending on the particular application, any stationary phase could be used as the analytical column. In their application, a fraction of the effluent from the SEC column was transferred to the analytical column by the use of a C18 collection column that served to pre-concentrate and focus the analytes. They noted that frequent solvent flushing was required to avoid build-up of impurities on the collection column. A chromatogram obtained from a 50 L plasma standard injection is shown in Figure 15.6. This separation provides an excellent
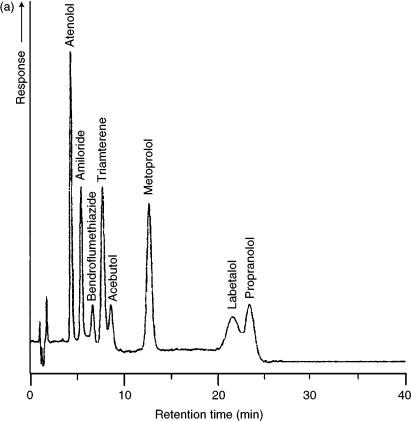
Forensic and Toxicological Applications |
413 |
Figure 15.4 Separation of mixtures of beta-blockers by using micellar HPLC, employing the following mobile phases: (a) 0.12M SDS, 5% propanol, 0.5% triethylamine; (b) 0.06 M SDS, 15% propanol; (c) 0.11M SDS, 8% propanol. Adapted from Journal of Chromatographic Science, 37, S. Carda-Broch et al., ‘Analysis of urine samples containing cardiovascular drugs by micellor liquid chromatography with fluorimetric detection’, pp. 93 – 102, 1999, with permission from Preston Publications, a division of Preston Industries, Inc.
(continued to p. 414)
example of the ability of LC – LC to provide on-line sample clean-up along with high resolution of closely related structures.
Multidimensional LC has also been used to determine ursodeoxycholic acid and its conjugates in serum (14). These compounds are used in the treatment of cholesterol gallstones, hepatitis and bilary cirrhosis. These authors employed a traditional (10 4 mm) pre-column and a micro-bore (35 2 mm) analytical column that were interfaced by using a six-port switching valve.
Drug discovery applications also provide insights for the eventual determination of the compounds in a forensic or toxicological setting; this is another area in which multidimensional chromatography allows the direct injection of bodily fluids. For
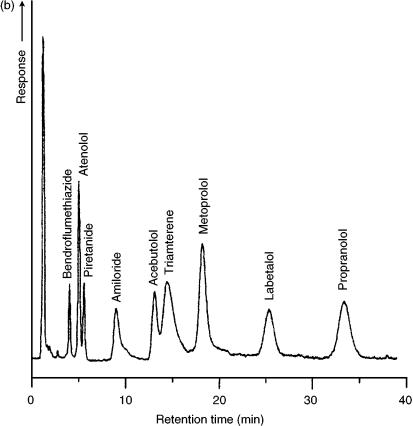
414 |
Multidimensional Chromatography |
Figure 15.4 (continued to p. 415)
example, Szuna and Blain (15) described the determination of a new antibacterial agent by multidimensional HPLC using a guard column and a reversed phase analytical column. Mulligan et al. (16) combined liquid – liquid extraction with multidimensional HPLC for the analysis of a platelet aggregating factor receptor antagonist, again, by direct injection of bodily fluids. These two applications from drug discovery also demonstrate the applicability of multidimensional LC to a variety of toxicologically related problems.
15.4GAS CHROMATOGRAPHY –GAS CHROMATOGRAPHY
GC – GC has typically been employed for complex samples or those requiring additional chemistry, such as chiral recognition, to be employed along with classical GC separation. Typical GC – GC systems employ multiple capillary columns connected
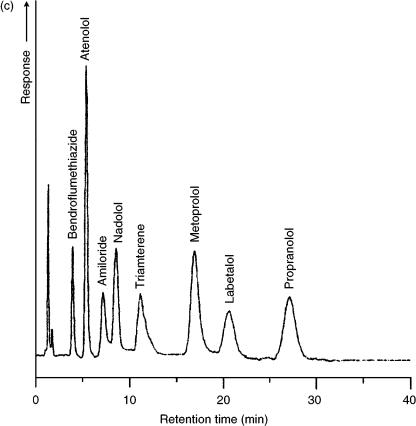
Forensic and Toxicological Applications |
415 |
Figure 15.4 (continued )
with a switching valve or a cryogenically cooled concentrator. The multidimensional separation may also be combined with on-column or large volume injection. Applications in forensic and toxicological analysis are presented here, while instrumental and theoretical details can be found in Chapters 3 and 4 of this volume.
Recently, multidimensional GC has been employed in enantioselective analysis by placing a chiral stationary phase such as a cyclodextrin in the second column. Typically, switching valves are used to heart-cut the appropriate portion of the separation from a non-chiral column into a chiral column. Heil et al. used a dual column system consisting of a non-chiral pre-column (30 m 0.25 mm 0.38 m, PS -268) and a chiral (30 m 0.32 mm 0.64 m, heptakis(2,3-di-O-methyl-6-O- tert-butyldimethylsilyl)- -cyclodextrin) (TBDM-CD) analytical column to separate derivatized urinary organic acids that are indicative of metabolic diseases such as short bowel syndrome, phenylketonuria, tyrosinaemia, and others. They used a FID following the pre-column and an ion trap mass-selective detector following the
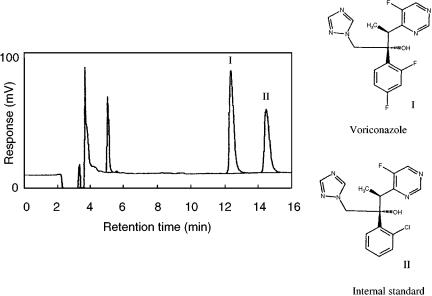
416 |
Multidimensional Chromatography |
Figure 15.5 Separation of Voriconazole and an internal standard by using SEC–HPLC. Adapted from Journal of Chromatography, B 691, D.A. Stopher and R. Gage, ‘Determination of a new antifungal agent, voriconazole, by multidimensional high-performance liquid chromatography with direct plasma injection onto a size exclusion column’, pp. 441 – 448, copyright 1997, with permission from Elsevier Science.
chiral column (17). Using the same approach, Podebrad et al. (18) determined several chiral amino acids that are indicative of maple syrup urine disease, which is a disorder involving several amino acids that leads to mental retardation and neurological damage. In addition, Kaunznger et al. used this approach in the analysis of 2-1 and 3-hydroxy aekanoic acids from the biomembranes of bacteria (19).
Figure 15.7 shows the chiral separation of several methyl esters of hydroxy alkanoic acids using multidimensional GC. The high resolving power of the achiral column is seen in chromatogram (a), which presents the separation of single enantiomers of three acids. The chiral selectivity of the second column is illustrated in chromatogram (b), which shows the separation of three enantiomeric pairs. The resolving power of capillary GC is also illustrated, as the selectivity of the chiral column is quite low, and yet the enantiomers are still resolved. The analysis time is relatively long, and could perhaps be lessened by using a shorter achiral column, as there is excess resolution of the three acids.
Figure 15.8 shows the multidimensional GC analysis of urinary acids, following lyophilization and derivatization by methyl chloroformate. In this figure, chromatogram (a) shows the complexity of the urine matrix and the need for a second separation dimension. A heart-cut is taken over a small range at about 45 min. The
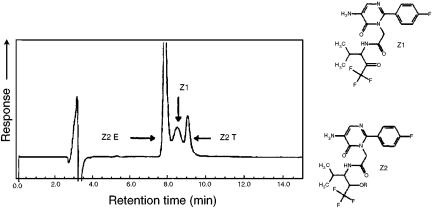
Forensic and Toxicological Applications |
417 |
Figure 15.6 Chromatogram of a plasma standard of human leukocyte elastase inhibitors obtained by using LC–LC. Adapted from Journal of Liquid Chromatography and Related Technologies, 19, R. A. Earley and L. P. Tini, ‘Versatile multidimensional chromatographic system for drug discovery as exemplified by the analysis of a non-peptidic inhibitor of human leukocyte elastase’, pp. 2527 – 2540, 1996, by courtesy of Marcel Dekker Inc.
second dimension separation (chromatogram (b) shows the GC/MS analysis (mass 84) for pyroglutamic acid enantiomers, separated on a chiral column. This allows the study of both enantiomers, which may be toxicologically significant, with a minimum of matrix interference. Again, these analysis times are relatively long, although high resolution in the first dimension is needed due to the complexity of the sample matrix.
By using a similar approach, bornane congeners, which indicate the presence of toxaphene, were analyzed by de Geus et al. (20). This presented a special analytical problem, as there are hundreds of congeners, many of which exist as racemates, with each of them having different toxicological properties. They also may be present in numerous biological and non-biological matrices. Samples of hake liver and dolphin blubber underwent Soxhlet extraction (pentane – dichloromethane (1:1)) and column chromatography to isolate the toxaphene compounds. Multidimensional GC was performed by using an Ultra-2 (24 m 0.2 mm 0.33 m) pre-column, followed by an OV-1701 column (25 m 0.25 mm 0.15 m), spiked with 10 wt% by TBDM-CD. Again, a heart-cutting technique was employed.
The enantioselective determination of 2,2 ,3,3 ,4,6 -hexachlorobiphenyl in milk was performed by Glausch et al. (21). These authors used an achiral column for an initial separation, followed by separation of the eluent fraction on a chiral column. Fat was separated from the milk by centrifugation, mixed with sodium sulfate, washed with petroleum ether and filtered. The solvent was evaporated and the sample was purified by gel permeation chromatography (GPC) and silica gel adsorption chromatography. Achiral GC was performed on DB-5 and OV-1701 columns, while the chiral GC was performed on immobilized Chirasil-Dex.
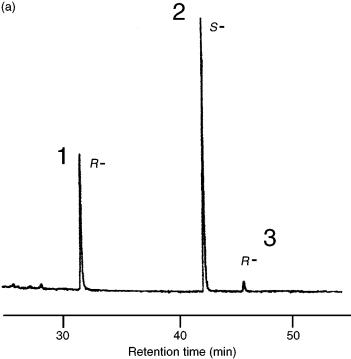
418 |
Multidimensional Chromatography |
Figure 15.7 Chromatographic separation of chiral hydroxy acids from Pseudomonas aeruginosa without (a) and with (b) co-injection of racemic standards. Peak identification is as follows: 1, 3-hydroxy decanoic acid, methyl ester; 2, 3-hydroxy dodecanoic acid, methyl ester; 3, 2-hydroxy dodecanoic acid, methyl ester. Adapted from Journal of High Resolution Chromatography, 18, A. Kaunzinger et al., ‘Stereo differentiation and simultaneous analysis of 2- and 3-hydroxyalkanoic acids from biomembranes by multidimensional gas chromatography’, pp. 191 – 193, 1995, with permission from Wiley-VCH. (continued p. 419)
Jayatilaka and Poole analyzed fire debris by using headspace extraction followed by multidimensional gas chromatography (22). Since petroleum products are commonly used to start ‘suspicious’ fires, the samples are very complex, with misidentification of the propellant being common when analyzed by classical methods. In addition because of the complexity of the samples, pattern recognition by humans or by computers must be used to identify the samples, thus causing errors. Multidimensional gas chromatography can be used to simplify the final pattern recognition. For example, a second-dimension polar stationary phase can be used to separate aliphatic and aromatic portions of a heart-cut from a complex chromatogram on a non-polar column.
The use of heart-cuts to analyze a sample of 90% evaporated gasoline is illustrated in Figure 15.9. Each of the lettered chromatograms illustrates the further separation of mixture components which is not possible on a single column. The initial
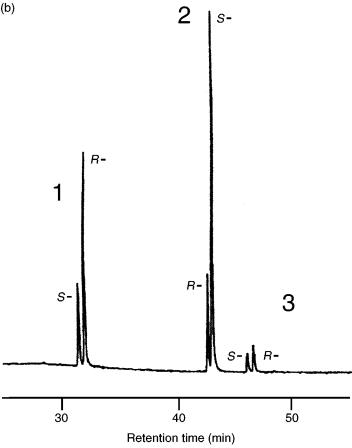
Forensic and Toxicological Applications |
419 |
Figure 15.7 (continued)
separation is performed on a non-polar DB-5 (5% phenyl polydimethylsiloxane) column, while and the second separation is camed out on a more polar DB-225 (50% cyanopropylphenyl methyl polysiloxane) column, which allows highly selective separation of the aromatic components. This presents an excellent example of target compound analysis from a very complex matrix. Note that the individual targets which are not resolved in the first dimension are mostly well resolved in the second. This is also an excellent example of the use of multiple heart-cuts within a single analytical run, opening up the possibility of performing numerous analyses with a single injection.
Multiple cryogenic traps between the pre-column and the analytical column were developed in 1993 by Ragunathan et al. (23). These authors found that a multiple trap configuration provided the necessary resolution and automated sampling for simultaneous GC/IR and GC/MS determination of very complex mixtures. A